U.S. patent application number 16/358227 was filed with the patent office on 2019-07-11 for sige fins formed on a substrate.
The applicant listed for this patent is INTERNATIONAL BUSINESS MACHINES CORPORATION. Invention is credited to Veeraraghavan S. Basker, Kangguo Cheng, Theodorus E. Standaert, Junli Wang.
Application Number | 20190214253 16/358227 |
Document ID | / |
Family ID | 58777308 |
Filed Date | 2019-07-11 |



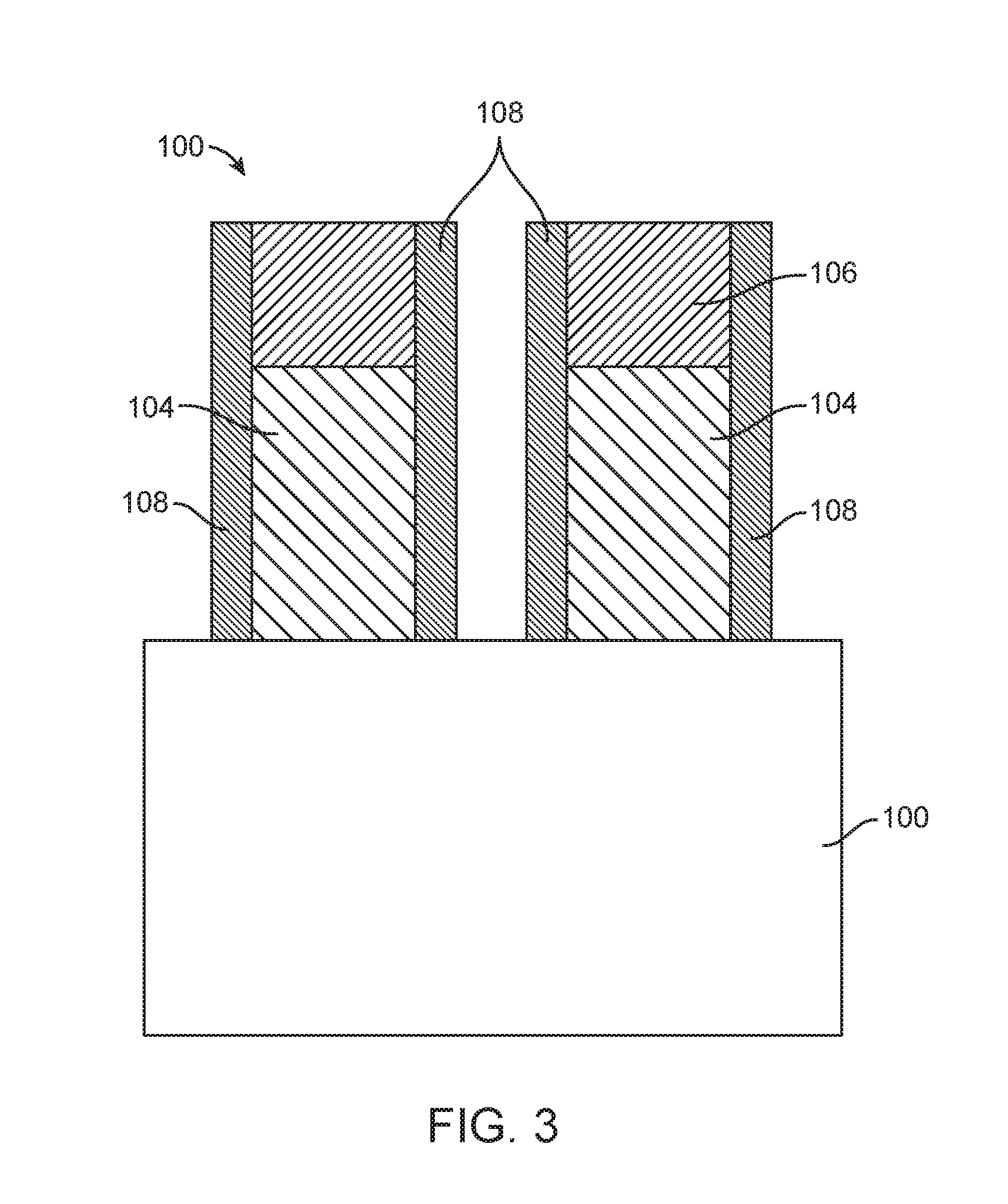

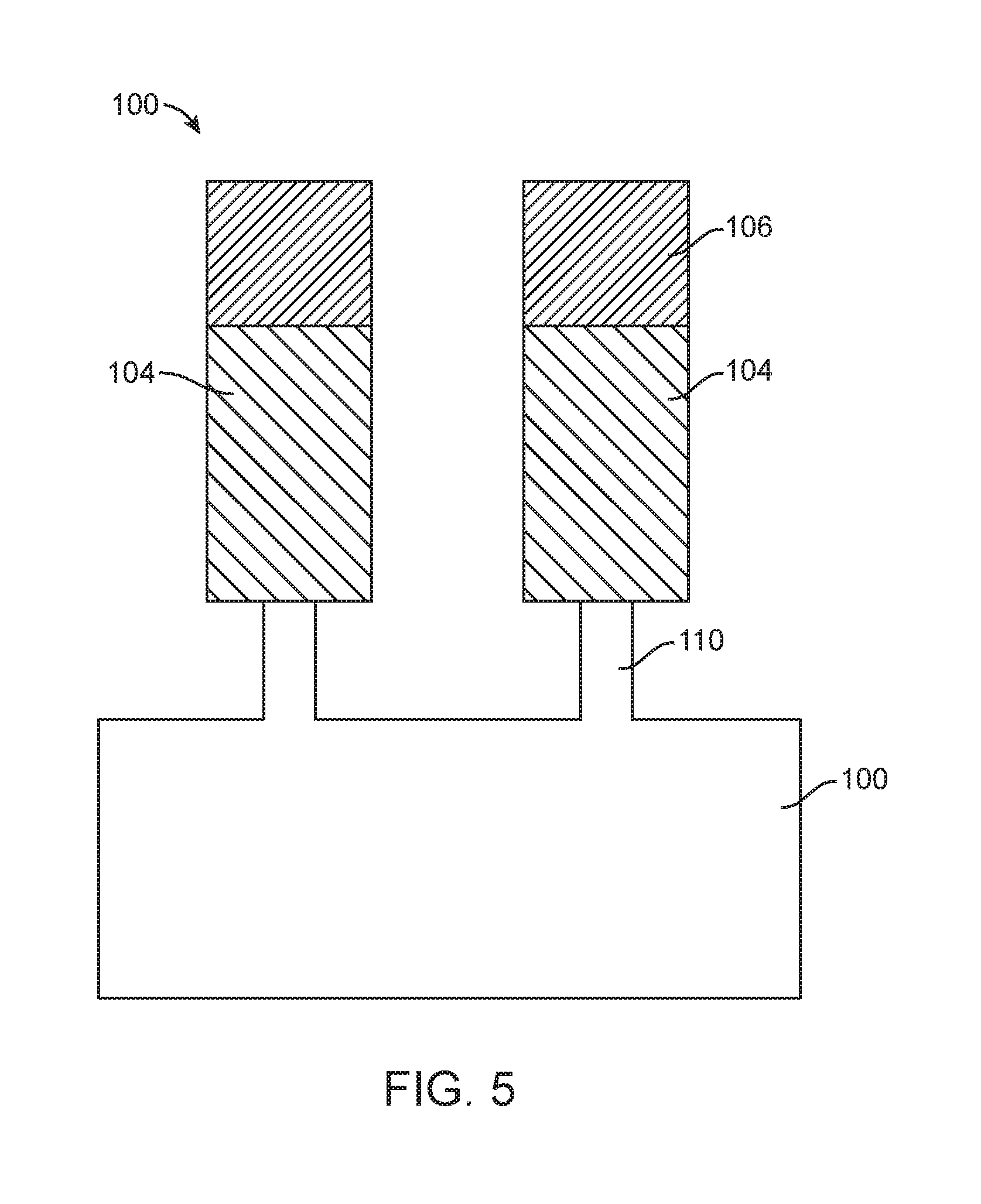
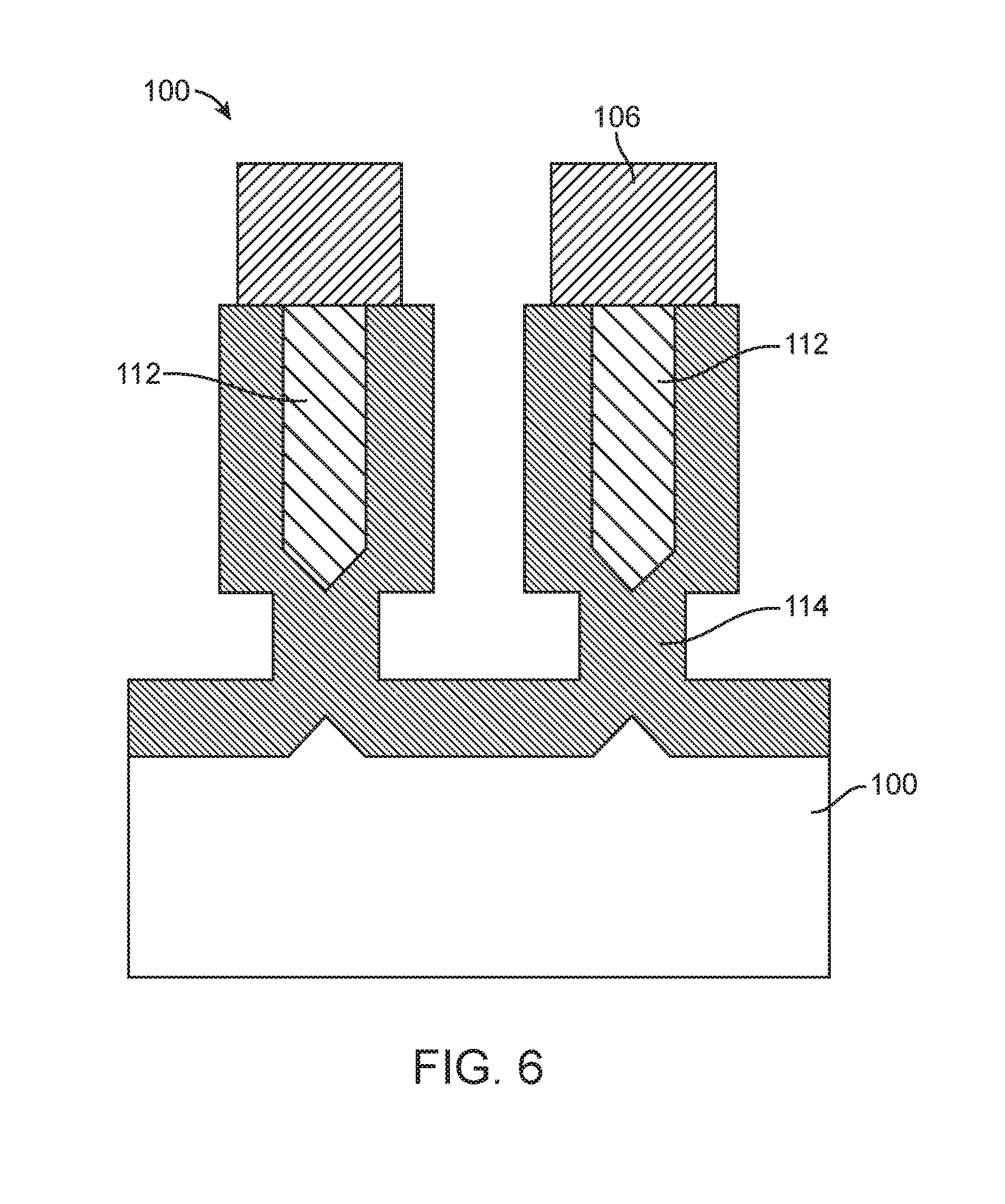
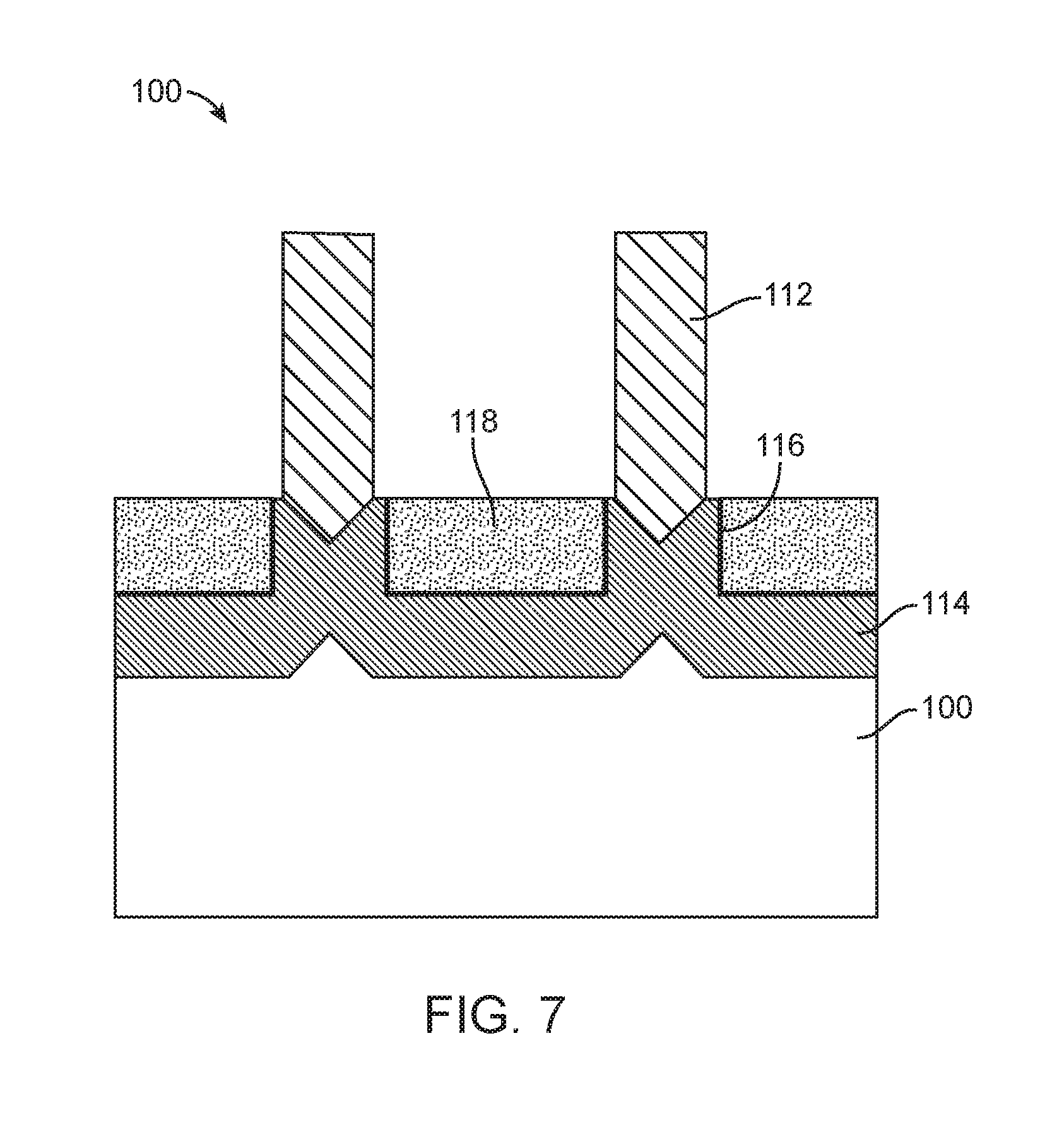

United States Patent
Application |
20190214253 |
Kind Code |
A1 |
Basker; Veeraraghavan S. ;
et al. |
July 11, 2019 |
SiGe FINS FORMED ON A SUBSTRATE
Abstract
A semiconductor structure includes at least one
silicon-germanium (SiGe) fin with a first width. The semiconductor
structure includes at least one silicon (Si) fin with a second
width. An oxide layer isolates the at least one SiGe fin with the
first width from a substrate. The at least one SiGe fin with the
first width, the at least one Si fin with the second width and a
surface of the substrate below the at least one Si fin are each
oxidized.
Inventors: |
Basker; Veeraraghavan S.;
(Schenectady, NY) ; Cheng; Kangguo; (Schenectady,
NY) ; Standaert; Theodorus E.; (Clifton Park, NY)
; Wang; Junli; (Slingerlands, NY) |
|
Applicant: |
Name |
City |
State |
Country |
Type |
INTERNATIONAL BUSINESS MACHINES CORPORATION |
Armonk |
NY |
US |
|
|
Family ID: |
58777308 |
Appl. No.: |
16/358227 |
Filed: |
March 19, 2019 |
Related U.S. Patent Documents
|
|
|
|
|
|
Application
Number |
Filing Date |
Patent Number |
|
|
14954581 |
Nov 30, 2015 |
10297448 |
|
|
16358227 |
|
|
|
|
Current U.S.
Class: |
1/1 |
Current CPC
Class: |
H01L 21/02634 20130101;
H01L 29/66795 20130101; H01L 21/02532 20130101; H01L 29/785
20130101 |
International
Class: |
H01L 21/02 20060101
H01L021/02; H01L 29/78 20060101 H01L029/78; H01L 29/66 20060101
H01L029/66 |
Claims
1. A semiconductor structure, comprising: at least one
silicon-germanium (SiGe) fin with a first width; at least one
silicon (Si) fin with a second width; an oxide layer isolating the
at least one SiGe fin with the first width from a substrate,
wherein the at least one SiGe fin with the first width, the at
least one Si fin with the second width and a surface of the
substrate below the at least one Si fin are each oxidized.
2. The semiconductor structure of claim 1, wherein the oxide layer
is formed based on oxidizing a top surface of the substrate, the
SiGe fin with the first width and the Si fin with the second
width.
3. The semiconductor structure of claim 2, wherein the Si fin with
the second width is formed based on etching the Si fin with the
second width from the substrate.
4. The semiconductor structure of claim 3, wherein the SiGe fin
with a first width is formed based on etching the at least one SiGe
fin with the first width from an SiGe layer.
5. The semiconductor structure of claim 4, wherein the SiGe layer
resides on the substrate.
6. The semiconductor structure of claim 5, wherein a height of the
at least one SiGe fin with the first width is reduced after
oxidizing and the first width is reduced after oxidizing.
7. The semiconductor structure of claim 6, wherein a percentage of
Ge in the at least one SiGe fin with the reduced first width is
greater than a percentage of Ge in the SiGe layer.
8. The semiconductor structure of claim 2, wherein the first width
of the at least one SiGe fin is greater than the second width of
the at least one Si fin.
9. The semiconductor structure of claim 2, wherein a portion of Si
from the at least one SiGe fin with the first width and the at
least one Si fin with the second width as a result of forming the
oxide layer.
10. A method, comprising: selectively forming a silicon-germanium
(SiGe) layer on a substrate by epitaxially growing the SiGe layer
on a top surface of the substrate; forming at least one fin with a
first width from the SiGe layer by forming a masking layer on the
top surface of the SiGe layer, etching the at least one fin with
the first width, and forming a spacer layer on the at least one fin
with the first width; forming at least one other fin with a second
width from the substrate by etching the substrate to form the at
least one other fin with the second width, wherein the second width
is less than the first width, and selectively removing the spacer
layer from the at least one fin with the first width; and oxidizing
the at least one fin with the first width, the at least one other
fin with the second width and a surface of the substrate below the
at least one other fin with the second width, wherein the first
width is condensed in width to a target width, the at least one
other fin is completely oxidized forming an oxide layer between the
at least one fin with the first width and the substrate.
11. The method of claim 10, wherein oxidizing the at least one
other fin with the second width creates the oxide layer that
isolates the at least one fin with the first width from the
substrate; a height of the at least one fin with the first width is
reduced after oxidizing; the first width is reduced after
oxidizing; and a percentage of Ge in the at least one fin with the
reduced first width is greater than a percentage of Ge in the SiGe
layer.
Description
BACKGROUND
[0001] SiGe Fin field-effect transistor (FinFET) semiconductor
structures are a viable option for continued scaling of FinFET to
10 nm and beyond, however, there are two major issues with SiGe fin
fabrication. SiGe fins formed by growing a SiGe layer on a bulk Si
substrate is limited by the so-called critical thickness. When SiGe
is grown on Si, beyond the critical thickness, dislocations start
to generate in SiGe films, resulting in defective SiGe fins.
Isolation of SiGe fin from the bulk Si is not trivial. N-type
dopants (e.g., phosphorus or arsenic) are used for a punchthrough
stopping region under SiGe fins to suppress source/drain
punchthrough. Unfortunately, N-type dopants have a greater
diffusion rate in SiGe than in Si, resulting in undesired
encroachment of punchthrough Si (PTS) dopants into SiGe channel,
resulting in degradation of device performance and increase of
device variability.
SUMMARY
[0002] Embodiments relate to semiconductor structures, in
particular, for defect-free silicon-germanium (SiGe)-on-insulator
fins formed on a bulk silicon (Si) substrate and a method of
manufacturing the same. In one embodiment, a semiconductor
structure includes at least one SiGe fin with a first width. The
semiconductor structure includes at least one Si fin with a second
width. An oxide layer isolates the at least one SiGe fin with the
first width from a substrate. The at least one SiGe fin with the
first width, the at least one Si fin with the second width and a
surface of the substrate below the at least one Si fin are each
oxidized.
[0003] In one embodiment, a method includes selectively forming a
SiGe layer on a substrate by epitaxially growing the SiGe layer on
a top surface of the substrate. At least one fin with a first width
is formed from the SiGe layer by forming a masking layer on the top
surface of the SiGe layer, etching the at least one fin with the
first width, and forming a spacer layer on the at least one fin
with the first width. At least one other fin with a second width is
formed from the substrate by etching the substrate to form the at
least one other fin with the second width. The second width is less
than the first width. The spacer layer is selectively removed from
the at least one fin with the first width. The at least one fin
with the first width, the at least one other fin with the second
width and a surface of the substrate below the at least one other
fin with the second width are oxidized. The first width is
condensed in width to a target width. The at least one other fin is
completely oxidized forming an oxide layer between the at least one
fin with the first width and the substrate.
[0004] These and other features, aspects and advantages of the
embodiments will become understood with reference to the following
description, appended claims and accompanying figures.
BRIEF DESCRIPTION OF THE DRAWINGS
[0005] FIG. 1 is a cross-sectional view of an exemplary
semiconductor structure with a silicon-germanium (SiGe) layer
formed on a substrate, according to one embodiment;
[0006] FIG. 2 is a cross-sectional view of a result of the
exemplary structure of FIG. 1 after selective formation of at least
one SiGe fin with a first width from the SiGe layer, according to
an embodiment;
[0007] FIG. 3 is a cross-sectional view of a result of the
exemplary structure of FIG. 2 after forming a spacer layer,
according to an embodiment;
[0008] FIG. 4 is a cross-sectional view of the result of the
exemplary structure of FIG. 3 after formation of at least one
silicon (Si) fin with a second width from the substrate, according
to an embodiment;
[0009] FIG. 5 is a cross-sectional view of the result of the
exemplary structure of FIG. 4 after selectively removing the spacer
layer from the SiGe fins, according to an embodiment;
[0010] FIG. 6 is a cross-sectional view of the result of the
exemplary structure of FIG. 5 after condensing the at least one
SiGe fin with the first width and the at least one Si fin with the
second width, according to an embodiment;
[0011] FIG. 7 is a cross-sectional view of the result of the
exemplary structure of FIG. 6 after selectively removing an oxide
layer from the at least one SiGe fin with a first width, according
to an embodiment; and
[0012] FIG. 8 illustrates a block diagram for a process for forming
defect-free silicon-germanium (SiGe)-on-insulator fins on a bulk Si
substrate, according to one embodiment.
DETAILED DESCRIPTION
[0013] The descriptions of the various embodiments have been
presented for purposes of illustration, but are not intended to be
exhaustive or limited to the embodiments disclosed. Many
modifications and variations will be apparent to those of ordinary
skill in the art without departing from the scope and spirit of the
described embodiments. The terminology used herein was chosen to
best explain the principles of the embodiments, the practical
application or technical improvement over technologies found in the
marketplace, or to enable others of ordinary skill in the art to
understand the embodiments disclosed herein.
[0014] As used herein, a "lengthwise" element is an element that
extends along a corresponding lengthwise direction, and a
"widthwise" element is an element that extends along a
corresponding widthwise direction.
[0015] FIG. 1 is a cross-sectional view of an exemplary
semiconductor structure 100 with a silicon-germanium (SiGe) layer
102 formed on a substrate 100, according to one embodiment. In one
embodiment, the substrate 100 is a commercial silicon (Si)
substrate atop which the SiGe layer 102 is formed. In one
embodiment, the SiGe layer 102 is epitaxially grown atop the
substrate 100. In one embodiment, the SiGe layer 102 has a
relatively low Ge percentage (e.g., 5-15 percent) as compared to
the overall makeup of the SiGe layer 102. The low Ge percentage in
the SiGe layer 102 allows for the manufacture of taller SiGe fins
without defects. Further, the SiGe layer 102 is taller than the
desired SiGe fins (112, FIG. 6), taking into account the height and
width reduction in the condensing step (208, FIG. 8). In one
embodiment, the SiGe layer may be gown to a height of at least 50
nm. It is appreciated that the taller the SiGe layer, the taller
the resultant SiGe fins can be manufactured.
[0016] FIG. 2 is a cross-sectional view of a result of the
exemplary structure 100 of FIG. 1 after selective formation of at
least one SiGe fin 104 with a first width from the SiGe layer 102
(FIG. 1), according to an embodiment. In this embodiment, prior to
formation of the SiGe fins 104 a hardmask layer 106 is applied
and/or formed on a top surface of the SiGe layer 102 (FIG. 1). In
one embodiment, the hardmask layer is silicon nitride and applied
in the conventional spacer image and transfer (STI) process. The
SiGe fins 104 are formed in a conventional process (e.g., dry
etching, wet etching, etc.). At this point in the process, the SiGe
fins 104 have a first width which is greater than the width of the
SiGe fins after the condensing/oxidation step (208, FIG. 8). In one
embodiment, the first width of the SiGe fins 104 is 20 nm, where
the target/final width may be 8 nm.
[0017] FIG. 3 is a cross-sectional view of a result of the
exemplary structure 100 of FIG. 2 after forming a spacer layer 108,
according to an embodiment. In this embodiment, the spacer layer
108 is applied only to the sidewalls of the SiGe fins 104. The
spacer layer 108 may be made up of the same material as the
hardmask 106 (e.g., silicon-nitride) or distinct from the hardmask
106 (e.g., oxide). In one embodiment, the thickness of the spacer
layer 108 is less than the thickness of the hardmask layer 106 to
allow for subsequent removal (e.g., etching) of the spacer layer
106 from the SiGe fins 104 while maintaining the hardmask layer
106.
[0018] FIG. 4 is a cross-sectional view of the result of the
exemplary structure 100 of FIG. 3 after formation of at least one
Si fin 110 with a second width from the substrate 100, according to
an embodiment. In this embodiment, only portions of the substrate
100 is selective removed (e.g., dry etching, wet etching, etc.) to
form Si fins 110. It is appreciated that for each SiGe fin 104,
there is a corresponding Si fin 110. Further, the Si fins 110 have
a width which is less than the width of the SiGe fins 104. This
allows for complete oxidation of the Si fins 110 without complete
oxidation of the SiGe fins 104 at the condensing/oxidation step
(208, FIG. 8). In one embodiment the Si fins 104 have a width of 10
nm.
[0019] FIG. 5 is a cross-sectional view of the result of the
exemplary structure 100 of FIG. 4 after selectively removing the
spacer layer 108 from the SiGe fins 104, according to an
embodiment. In one embodiment, the spacer layer 108 (FIG. 4) is
selectively removed from the structure 100 by etching (e.g., wet
etching, dry etching, time etching, etc.).
[0020] FIG. 6 is a cross-sectional view of the result of the
exemplary structure 100 of FIG. 5 after condensing the at least one
SiGe fin 104 with the first width and the at least one Si fin 110
with the second width, according to one embodiment. In this
embodiment, condensing the structure 100 includes performing an
oxidation to each of the SiGe fins 104, the Si fins 110 (FIG. 5)
and the top surface of the substrate 100. In one embodiment, the Si
fins 110 are completely oxidized by the condensing/oxidization
process, forming an oxide layer (e.g. barrier) between the SiGe
fins 104 and the substrate 100. Oxidization of the SiGe fins 104 to
remove a portion of the Si from the SiGe fins results in SiGe fins
112 with a higher Ge percentage as compared to the percentage of Ge
in the SiGe layer 102 (FIG. 1). Oxidization also has the effect of
condensing the width of the SiGe fins. In this embodiment, the
oxide layer (e.g., barrier) 114 isolates the SiGe fins 112 from the
substrate 100. The result is that in this configuration, there is
no need to employ a punchthrough, thus reducing the risk of leakage
into the substrate.
[0021] For example, with SiGe fins 104 having a 20 nm width and the
Si fins 110 having a 10 nm width, a 20 nm oxidization of the SiGe
fins 104 and Si fins 110 will result in fully oxidized Si fins
oxidize 12 nm oxidization of the SiGe fin 112. As a result, the
percentage of Ge in the oxidized SiGe fins 112 is higher than the
percentage of Ge to Si in the SiGe layer 102 (e.g., 25 percent
compared to 10 percent). Moreover, the higher Ge SiGe fins 112 have
been condensed in width to their target width (8 nm in this
example).
[0022] FIG. 7 is a cross-sectional view of the result of the
exemplary structure 100 of FIG. 6 after selectively removing an
oxide layer 114 (FIG. 6) from the at least one SiGe fin with a
first width (112), according to one embodiment. In this embodiment,
the oxide layer 114 (FIG. 6) is selectively removed from the side
surfaces of the SiGe fins 102 having a higher concentration of Ge
112. Here, the oxide layer 114 may be thermal oxide. Further, a
nitride liner 116 may be deposited on the surface of the oxide
layer 114 and then a flowable oxide layer 118 may be deposited on
the nitride liner 116. It will be appreciated that complete Fin
field-effect transistor (FinFET) fabrication is not detailed in
FIG. 7.
[0023] FIG. 8 illustrates a block diagram for a process 200 for
forming defect-free silicon-germanium (SiGe)-on-insulator fins 112
(FIG. 7) on a bulk silicon (Si) substrate 100 (FIG. 7), according
to one embodiment. In one embodiment, in block 202 a SiGe layer is
formed on a top surface of a substrate. The SiGe layer may be
formed by epitaxially growing the SiGe on a Si substrate. In one
embodiment, the SiGe layer will have a percentage of Ge of between
5-15% compared to the percentage of Si. Moreover, the SiGe layer
will have an optimal height greater than a height of formed SiGe
fins to account for condensing of the fin in block 208.
[0024] In one embodiment, in block 204 formation of SiGe fins may
comprise forming a hardmask layer (106, FIG. 2) on a top surface of
the SiGe layer (102, FIG. 2), then etching the SiGe fins from the
SiGe layer. In one embodiment, the hardmask layer is silicon
nitride and applied in the conventional spacer image and transfer
(STI) process. The resultant SiGe fins have a first width which is
greater than the width of the SiGe fins after the condensing step
208. For example, with a target width of 8 nm, the SiGe fins formed
in block 204 may have a thickness of 20 nm.
[0025] In one embodiment, block 206 comprises applying a spacer
layer 108 (FIG. 4) to the side surfaces of the SiGe fins 104 (FIG.
4). Thereafter, the Si fins 110 (FIG. 4) are formed (e.g., etched)
from the substrate 100 (FIG. 4). In one embodiment, after forming
the Si fins 110, the spacer layer 108 is removed from the SiGe fins
104 (FIG. 5). In one embodiment, the spacer layer 108 (FIG. 4) is
selectively removed from the structure 100 by etching (e.g., wet
etching, dry etching, time etching, etc.).
[0026] In one embodiment, step 208 involves condensing the SiGe
fins 104 (FIG. 6) by means of oxidizing the SiGe fins 104, Si fins
110 and the top surface of the substrate 100 (FIG. 5). By oxidizing
Si from the SiGe fins, the resultant fin has a higher percentage of
Ge as compared to the percentage of Ge in the SiGe layer. Further,
the resultant fin has a width more narrow than the fabricated
(pre-oxidized) SiGe fins in block 204.
[0027] Moreover, in one embodiment, as a result of condensing the
SiGe fins and Si fins in block 208, the Si is fully oxidized from
the Si fins, resulting in the formation of an oxide layer 114 (FIG.
6) isolating the SiGe fins from the substrate.
[0028] In one embodiment, by forming the Si fins 204, the Si fins
have a width which is less than the width of the SiGe fins 104
(FIG. 5) to allow for complete oxidation of the Si fins 110 without
complete oxidation of the SiGe fins 104 during condensing 208.
[0029] The exemplary methods and techniques described herein may be
used in the fabrication of IC chips. In one embodiment, the IC
chips may be distributed by a fabricator in raw wafer form (i.e.,
as a single wafer that has multiple unpackaged IC chips), as a bare
die, or in a packaged form. In the latter case, the IC chip is
mounted in a single IC chip package (e.g., a plastic carrier with
leads that are affixed to a motherboard or other higher level
carrier) or in a multiIC chip package (e.g., a ceramic carrier that
has either or both surface interconnections or buried
interconnections). The IC chip is then integrated with other IC
chips, discrete circuit elements and/or other signal processing
devices as part of either (a) an intermediate product, such as a
motherboard, or (b) an end product, such as microprocessors, smart
phones, mobile phones, cellular handsets, set-top boxes, DVD
recorders and players, automotive navigation, printers and
peripherals, networking and telecom equipment, gaming systems, toys
and digital cameras, as non-limiting examples. One or more
embodiments may be applied in any of various highly integrated
semiconductor devices.
[0030] Unless described otherwise or in addition to that described
herein, "depositing" may include any now known or later developed
techniques appropriate for the material to be deposited, including,
but not limited to: CVD, LPCVD, PECVD, semi-atmosphere CVD (SACVD),
high density plasma CVD (HDPCVD), rapid thermal CVD (RTCVD),
ultra-high vacuum CVD (UHVCVD), limited reaction processing CVD
(LRPCVD), metalorganic CVD (MOCVD), sputtering deposition, ion beam
deposition, electron beam deposition, laser assisted deposition,
thermal oxidation, thermal nitridation, spin-on methods, PVD, ALD,
chemical oxidation, MBE, plating or evaporation. Any references to
"poly" or "poly silicon" should be understood to refer to
polycrystalline silicon.
[0031] References herein to terms such as "vertical", "horizontal,"
etc. are made by way of example, and not by way of limitation, to
establish a frame of reference. The term "horizontal" as used
herein is defined as a plane parallel to the conventional plane or
surface of the substrate, regardless of the actual spatial
orientation of the semiconductor substrate. The term "vertical"
refers to a direction perpendicular to the horizontal, as just
defined. Terms, such as "on," "above," "below," "side" (as in
"sidewall"), "higher," "lower," "over," "beneath" and "under," are
defined with respect to the horizontal plane. It is understood that
various other frames of reference may be employed for describing
one or more embodiments without departing from the spirit and scope
of the one or more embodiments.
[0032] References in the claims to an element in the singular is
not intended to mean "one and only" unless explicitly so stated,
but rather "one or more." All structural and functional equivalents
to the elements of the above-described exemplary embodiment that
are currently known or later come to be known to those of ordinary
skill in the art are intended to be encompassed by the present
claims. No claim element herein is to be construed under the
provisions of 35 U.S.C. section 112, sixth paragraph, unless the
element is expressly recited using the phrase "means for" or "step
for."
[0033] The terminology used herein is for the purpose of describing
particular embodiments only and is not intended to be limiting of
the embodiments. As used herein, the singular forms "a", "an" and
"the" are intended to include the plural forms as well, unless the
context clearly indicates otherwise. It will be further understood
that the terms "comprises" and/or "comprising," when used in this
specification, specify the presence of stated features, steps,
operations, elements, materials, and/or components, but do not
preclude the presence or addition of one or more other features,
steps, operations, elements, materials, components, and/or groups
thereof.
[0034] The corresponding structures, materials, acts, and
equivalents of all means or step plus function elements in the
claims below are intended to include any structure, material, or
act for performing the function in combination with other claimed
elements as specifically claimed. The description of the
embodiments has been presented for purposes of illustration and
description, but is not intended to be exhaustive or limited to the
embodiments in the form disclosed. Many modifications and
variations will be apparent to those of ordinary skill in the art
without departing from the scope and spirit of the embodiments. The
embodiment was chosen and described in order to best explain the
principles of the embodiments and the practical application, and to
enable others of ordinary skill in the art to understand the
embodiments with various modifications as are suited to the
particular use contemplated.
* * * * *