U.S. patent application number 17/029739 was filed with the patent office on 2021-01-14 for method of producing sic-si composite component and sic-si composite component.
This patent application is currently assigned to AGC Inc.. The applicant listed for this patent is AGC Inc.. Invention is credited to Shinya KIKUGAWA, Nobuhiro SHINOHARA, Yasuo SHINOZAKI, Shunsuke SUSA, Kenichiro TERADA, Kenji YAMADA.
Application Number | 20210009473 17/029739 |
Document ID | / |
Family ID | 1000005163498 |
Filed Date | 2021-01-14 |
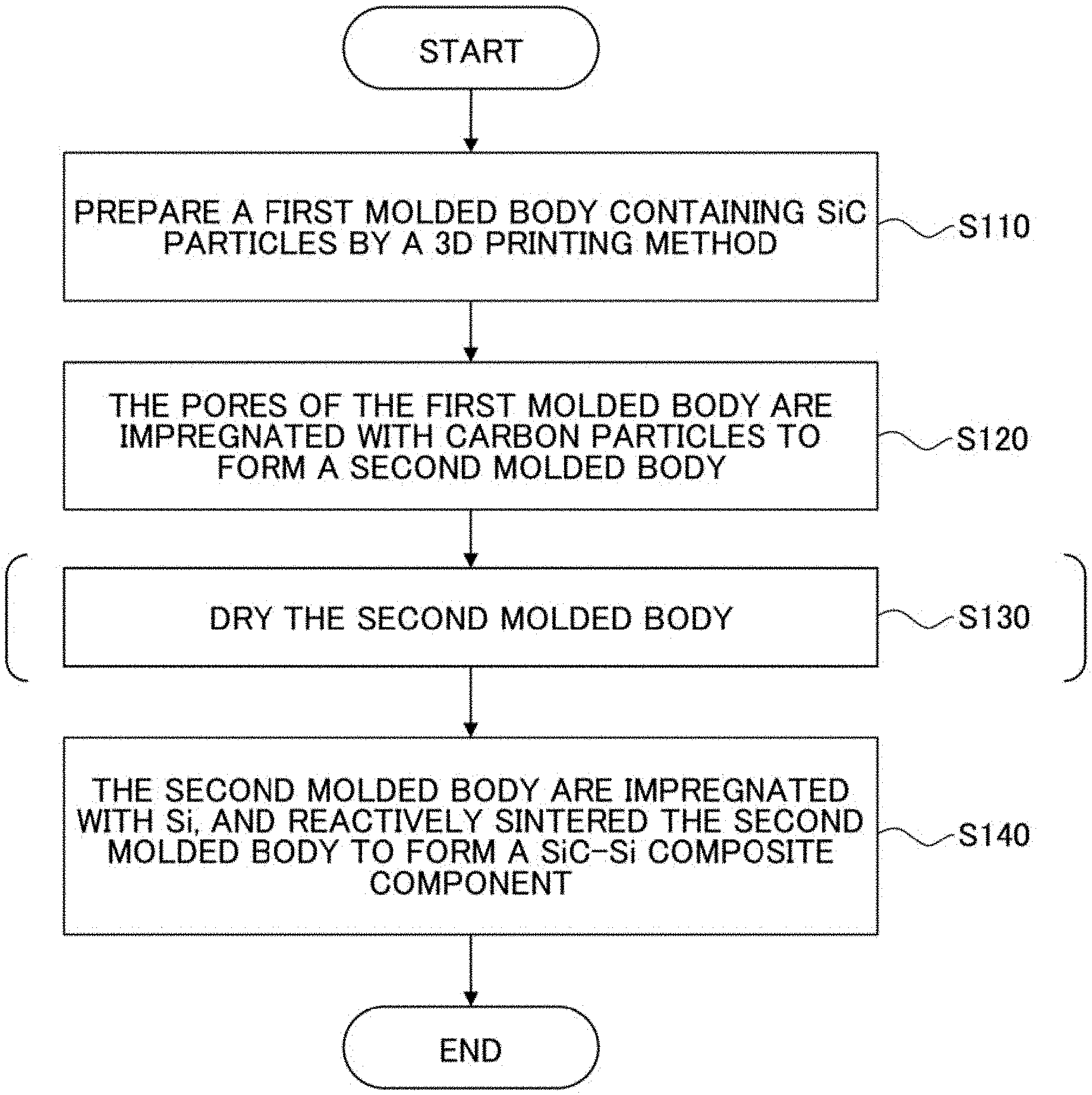





United States Patent
Application |
20210009473 |
Kind Code |
A1 |
YAMADA; Kenji ; et
al. |
January 14, 2021 |
METHOD OF PRODUCING SiC-Si COMPOSITE COMPONENT AND SiC-Si COMPOSITE
COMPONENT
Abstract
The present invention relates to a method of producing a SiC--Si
composite component. The method includes preparing a first molded
body containing SiC particles by a 3D printing method, wherein the
first molded body has a first average pore diameter M.sub.1;
forming a second molded body, in which the first molded body and a
dispersion containing carbon particles are brought into contact so
that the pores are impregnated with the carbon particles, wherein
the carbon particles have a secondary particle having an average
particle diameter M.sub.2, and the carbon particles satisfy the
following formula: M.sub.2.ltoreq.M.sub.1/10; and forming a SiC--Si
composite component by carrying out that the second molded body is
impregnated with a metallic Si and is reactively sintered; wherein
the content of Si is in the range of 5% by mass to 40% by mass in
the SiC--Si composite component.
Inventors: |
YAMADA; Kenji; (Tokyo,
JP) ; TERADA; Kenichiro; (Tokyo, JP) ;
SHINOHARA; Nobuhiro; (Tokyo, JP) ; KIKUGAWA;
Shinya; (Tokyo, JP) ; SHINOZAKI; Yasuo;
(Tokyo, JP) ; SUSA; Shunsuke; (Tokyo, JP) |
|
Applicant: |
Name |
City |
State |
Country |
Type |
AGC Inc. |
Tokyo |
|
JP |
|
|
Assignee: |
AGC Inc.
Tokyo
JP
|
Family ID: |
1000005163498 |
Appl. No.: |
17/029739 |
Filed: |
September 23, 2020 |
Related U.S. Patent Documents
|
|
|
|
|
|
Application
Number |
Filing Date |
Patent Number |
|
|
PCT/JP2019/014492 |
Apr 1, 2019 |
|
|
|
17029739 |
|
|
|
|
Current U.S.
Class: |
1/1 |
Current CPC
Class: |
C04B 2235/428 20130101;
C04B 35/573 20130101; C04B 2235/383 20130101; C04B 2235/6026
20130101; C04B 2235/5436 20130101; C09K 5/14 20130101; C04B
2235/3834 20130101; C04B 2235/422 20130101; C04B 35/657 20130101;
C04B 35/62839 20130101; C04B 2235/9607 20130101; C04B 35/62218
20130101; C04B 2235/5445 20130101; C04B 2235/616 20130101; C04B
2235/77 20130101 |
International
Class: |
C04B 35/573 20060101
C04B035/573; C04B 35/657 20060101 C04B035/657; C04B 35/622 20060101
C04B035/622; C04B 35/628 20060101 C04B035/628; C09K 5/14 20060101
C09K005/14 |
Foreign Application Data
Date |
Code |
Application Number |
Apr 3, 2018 |
JP |
2018-071771 |
Mar 27, 2019 |
JP |
2019-061755 |
Claims
1. A method of producing a SiC--Si composite component comprising:
preparing a first molded body containing SiC particles by a 3D
printing method, wherein the first molded body has a first average
pore diameter M.sub.1; forming a second molded body, in which the
first molded body and a dispersion containing carbon particles are
brought into contact so that the pores are impregnated with the
carbon particles, wherein the carbon particles have a secondary
particle having an average particle diameter M.sub.2, and the
carbon particles satisfy the following formula:
M.sub.2.ltoreq.M.sub.1/10; and forming a SiC--Si composite
component by carrying out that the second molded body is
impregnated with a metallic Si and is reactively sintered; wherein
the content of Si is in the range of 5% by mass to 40% by mass in
the SiC--Si composite component.
2. The method according to claim 1, wherein the first molded body
is brought into contact with the dispersion containing carbon
particles when the second molded body is formed.
3. The method according to claim 1, wherein the first average pore
diameter M.sub.1is in the range of 20 .mu.m to 100 .mu.m.
4. The method according to claim 1, wherein the SiC particles have
an average particle size in the range of 30 .mu.m to 200 .mu.m.
5. The method according to claim 1, wherein the average particle
diameter M2 is in the range of 100 nm to 200 nm.
6. The method according to claim 1, wherein a content of the carbon
particles in the dispersion is in the range of 20% by mass to 60%
by mass.
7. The method according to claim 1, wherein the first molded body
is prepared by inkjet printing a binder on a powder layer
containing the SiC particles.
8. The method according to claim 7, wherein the powder layer
further contains a curing agent.
9. The method according to claim 1, wherein drying the second
molded body after the second molded body is formed.
10. The method according to claim 9, wherein the second molded body
is vacuum freeze-dried.
11. The method according to claim 1, wherein the second molded body
is impregnated with a molten metallic Si when SiC--Si composite
component is formed.
12. The method according to claim 1, wherein forming the SiC--Si
composite component under the coexistence of the metallic Si and
the second molded body at a temperature equal to or higher than a
melting point of the metallic Si.
13. The method according to claim 1, wherein the SiC--Si composite
component obtained has a bulk density of 2.79 g/cm.sup.3 or
more.
14. A SiC--Si composite component comprising: a sea-island
structure containing .alpha.-SiC particles having an average
particle size of 30 .mu.m to 200 .mu.m, .beta.-SiC particles having
an average particle size of 1 .mu.m to 20 .mu.m, and a metallic Si,
wherein the metallic Si is configured as a sea portion of the
sea-island structure, and a first island portion formed from the
.alpha.-SiC particles and a second island portion formed from the
.beta.-SiC particles are placed in the sea portion configured by
the metallic Si.
15. The SiC--Si composite component according to claim 14, wherein
at least a part of the second island portion is configured by
connecting a plurality of the .beta.-SiC particles to each
other.
16. The SiC--Si composite component according to claim 15, wherein
when a cross-section of the SiC--Si composite component is
photographed at magnification of 20 times, a ratio P obtained by
the following procedure is 3 or more: drawing a circle having a
diameter of 200 .mu.m with any of the .beta.-SiC particles as a
center in the second island portion formed by connecting the
plurality of .beta.-SiC particles to each other; drawing a first
straight line having a length of 200 .mu.m and passing through the
center of the circle; drawing a second straight line having a
length of 200 .mu.m and having a rotation angle of 1.degree. with
respect to the first straight line with the center as a central
axis in the circle; drawing total 180 straight lines in the circle
in the same manner as the above until the rotation angle reaches
180.degree.; measuring each length of a line segment of one or a
plurality of straight line which across the sea portion, and
calculating an average value S.sub.ave of the length of the line
segment in each straight line; selecting a straight line in which
the average value S.sub.ave becomes maximum from the 180 straight
lines and set as maximum S.sub.max, selecting a straight line in
which the average value S.sub.ave becomes minimum from the 180
straight lines and set as minimum S.sub.min, and calculating the
ratio P by the following formula: P=S.sub.max/S.sub.min (1).
17. The SiC--Si composite component according to claim 14, wherein
a total content of the .alpha.-SiC particles and the .beta.-SiC
particles is 60% by mass or more and 95% by mass or less.
18. The SiC--Si composite component according to claim 14, wherein
a ratio of a volume of the .alpha.-SiC particles to a volume of the
.beta.-SiC particles (.alpha.-SiC/.beta.-SiC) is 0.5 to 10.
19. The SiC--Si composite component according to claim 14, wherein
a ratio of a volume of the .beta.-SiC particles to a volume of the
metallic Si (.beta.-SiC/metallic Si) is 0.4 to 12.
20. The SiC--Si composite component according to claim 14, wherein
a thermal conductivity is 200 W/mK or more.
Description
CROSS-REFERENCE TO RELATED APPLICATIONS
[0001] This U.S. non-provisional application is a continuation
application of and claims the benefit of priority under 35 U.S.C.
.sctn. 365(c) from PCT International Application PCT/JP2019/014492
filed on Apr. 1, 2019, which is designated the U.S., and is based
upon and claims the benefit of priority of Japanese Patent
Applications No. 2018-071771 filed on Apr. 3, 2018 and No.
2019-061755 filed on Mar. 27, 2019, the entire contents of which
are incorporated herein by reference.
TECHNICAL FIELD
[0002] The present invention relates to a method of producing a
SiC--Si composite component and a SiC--Si composite component.
BACKGROUND OF THE INVENTION
[0003] Sintered components formed by a composite material
containing silicon carbide (SiC) and silicon (Si) (hereinafter
referred to as "SiC--Si composite component") have a low
coefficient of thermal expansion, excellent heat resistance, wear
resistance and thermal conductivity, and has demands in many
fields.
[0004] SiC--Si composite components can be produced by applying,
for example, a casting method (for example, Patent Document 1 and
Non-Patent Document 1).
[0005] For example, in the method of Non-Patent Document 1, a
molded body containing silicon carbide powder and carbon powder is
prepared by applying a pressure casting method, followed by
impregnating molten metallic Si into the molded body so that the
molded body is reactively sintered to produce a SiC--Si composite
component.
RELATED-ART DOCUMENT
Patent Documents
[0006] Patent document 1: Japanese Unexamined Patent Publication
No. 2001-19552
Non-Patent Documents
[0007] Non-Patent Document 1: Shoko Suyama et al., Journal of the
ceramic society of Japan, 109 [4], pp. 315-321, (2001)
SUMMARY OF THE INVENTION
Problems to be Solved by the Invention
[0008] In recent years, there has been increasing demand for
SiC--Si composite components having complex shapes. However, it is
difficult to produce a SiC--Si composite component having a complex
shape using the above-described cast molding method.
[0009] Therefore, it has been proposed to produce a SiC--Si
composite component having a complex shape by a powder lamination
molding method (so-called "3D printing method").
[0010] In this method, for example, a first powder layer containing
SiC particles and a binder is irradiated with a laser. As a result,
the particles are bound to each other via the binder activated by
the heat of the laser. Then, the same process is performed on the
second powder layer laminated on the first powder layer. By
repeating such steps, a three-dimensional shaped molded body is
formed.
[0011] Then, the molded body thus formed is impregnated with Si,
whereby a SiC--Si composite component can be produced.
[0012] However, generally, a sintered component produced by the 3D
printing method has a problem that mechanical properties are not
favorable.
[0013] Therefore, there is still a demand for a technique for
producing a SiC--Si composite component having a complex shape
without sacrificing mechanical properties.
[0014] The present invention has been made in view of such a
background, and the present invention can be applied to the
production of a sintered component having a complex shape. An
object of the present invention is to provide a method of producing
a SiC--Si composite component capable of obtaining a sintered
component having a favorable mechanical strength. In addition, an
object of the present invention is to provide a SiC--Si composite
component having a favorable mechanical strength.
Means for Solving the Problems
[0015] The present invention provides a method of producing a
SiC--Si composite component including:
[0016] preparing a first molded body containing SiC particles by a
3D printing method, wherein the first molded body has a first
average pore diameter M.sub.1;
[0017] forming a second molded body, in which the first molded body
and a dispersion containing carbon particles are brought into
contact so that the pores are impregnated with the carbon
particles, wherein the carbon particles have a secondary particle
having an average particle diameter M.sub.2, and the carbon
particles satisfy the following formula:
M.sub.2.ltoreq.M.sub.1/10; and
[0018] forming a SiC--Si composite component by carrying out that
the second molded body is impregnated with a metallic Si and is
reactively sintered;
[0019] wherein the content of Si is in the range of 5% by mass to
40% by mass in the SiC--Si composite component.
[0020] In addition, the present invention provides a SiC--Si
composite component including:
[0021] a sea-island structure containing .alpha.-SiC particles
having an average particle size of 30 .mu.m to 200 .mu.m,
.beta.-SiC particles having an average particle size of 1 .mu.m to
20 .mu.m, and a metallic Si,
[0022] wherein the metallic Si is configured as a sea portion of
the sea-island structure, and a first island portion formed from
the .alpha.-SiC particles and a second island portion formed from
the .beta.-SiC particles are placed in the sea portion configured
by the metallic Si.
Effect of the Invention
[0023] The present invention can be applied to the production of a
sintered component having a complex shape, and the present
invention is able to provide a method of producing a SiC--Si
composite component that yields a sintered component having
favorable mechanical strength. In addition, the present invention
is able to provide a SiC--Si composite component having a favorable
mechanical strength.
BRIEF DESCRIPTION OF THE DRAWINGS
[0024] FIG. 1 is a diagram schematically showing a flow of an
example of a method of producing a SiC--Si composite component
according to an embodiment of the present invention.
[0025] FIG. 2 is a diagram showing an example of a micrograph of a
SiC--Si composite component according to an embodiment of the
present invention.
[0026] FIG. 3 is a diagram showing an example of a circle having a
diameter of 200 .mu.m drawn on the microstructure of the SiC--Si
composite component according to the embodiment of the present
invention.
[0027] FIG. 4 is a diagram showing an example of a first straight
line having a length of 200 .mu.m drawn in the circle shown in FIG.
3.
[0028] FIG. 5 is a diagram showing an example of an optical
microscope photograph of a cross-section of a SiC--Si composite
component according to an embodiment of the present invention.
[0029] FIG. 6 is a diagram showing an example of a Si mapping
pattern in the cross-section shown in FIG. 5.
[0030] FIG. 7 is a diagram showing an example of an .alpha.-SiC
mapping pattern in the cross-section shown in FIG. 5.
[0031] FIG. 8 is a diagram showing an example of a .beta.-SiC
mapping pattern in the cross-section shown in FIG. 5.
[0032] FIG. 9 is a graph showing a plot of an average value
S.sub.ave of line segments that across the Si portion, measured on
each of 180 straight lines drawn in the circle shown in FIG. 4.
DETAILED DESCRIPTION
[0033] An embodiment of the present invention will be described
below with reference to the drawings.
[0034] One aspect of the present invention is a method of producing
a SiC--Si composite component including:
[0035] (Step I) preparing a first molded body containing SiC
particles by a 3D printing method, wherein the first molded body
has a first average pore diameter M.sub.1;
[0036] (Step II) forming a second molded body, in which the first
molded body and a dispersion containing carbon particles are
brought into contact so that the pores are impregnated with the
carbon particles, wherein the carbon particles have a secondary
particle having an average particle diameter M.sub.2, and the
carbon particles satisfy the following formula:
M.sub.2.ltoreq.M.sub.1/10; and
[0037] (Step III) forming a SiC--Si composite component by carrying
out that the second molded body is impregnated with a metallic Si
and is reactively sintered;
[0038] wherein the content of Si is in the range of 5% by mass to
40% by mass in the SiC--Si composite component.
[0039] Because such a method of producing a SiC--Si composite
component uses the 3D printing method, it is possible to produce a
composite component with a complex shape and/or a large-sized
shape, which is difficult by the casting method.
[0040] Here, as described above, in the 3D printing method, a
molded body including many pores is formed. Therefore, there is a
problem in that the sintered body produced through such a molded
body does not exhibit favorable mechanical strength.
[0041] However, in the above production method, the first molded
body is prepared by using the 3D printing method in step (I), then
pores of the first molded body are impregnated with carbon
particles in step (II), so that a second molded body is formed.
[0042] Particularly, in step (II), the carbon particles contained
in the dispersion are selected so that the average particle
diameter M.sub.2of secondary particles is smaller than 1/10 of the
first average pore diameter M.sub.1 of the first molded body.
Therefore, the carbon particles can move into the pores of the
first molded body relatively easily, and the pores can be
impregnated with a relatively large number of carbon particles.
[0043] In the present application, the average pore diameter of the
pores of the molded body is defined as the pore diameter when the
volume of the cumulative pore becomes 50% in the cumulative volume
distribution chart of the pore diameter measured by the mercury
porosimeter.
[0044] The average particle size of the secondary particles
(aggregated particles) can be measured using a laser
diffraction/scattering particle size distribution measuring device
(MT3300EXII manufactured by MicrotracBEL Corp.).
[0045] Further, according to one embodiment of the present
invention, in step (III), the second molded body is impregnated
with Si, then the second molded body is reactively sintered. During
the reactive sintering, SiC is generated in the pores of the second
molded body by the reaction between the introduced Si and the
carbon particles. Because the pores are filled with the newly
generated SiC, a SiC--Si composite component having low porosity
and high bulk density can be produced.
[0046] As a result, although the 3D printing method is used in the
production method according to the embodiment of the present
invention, the mechanical strength of the produced SiC--Si
composite component can be significantly improved.
(Method of Producing SiC--Si Composite Component According to One
Embodiment of the Present Invention)
[0047] Hereinafter, an example of a method of producing a SiC--Si
composite component according to an embodiment of the present
invention will be described in detail with reference to FIG. 1.
[0048] FIG. 1 schematically shows a flow of an example of a method
of producing a SiC--Si composite component according to an
embodiment of the present invention.
[0049] As shown in FIG. 1, this production method (hereinafter,
referred to as "first production method") has the following steps:
[0050] (1) a step (step S110) of preparing a first molded body
containing SiC particles by a 3D printing method, [0051] (2) a step
of impregnating the pores of the first molded body with carbon
particles to form a second molded body (step S120), [0052] (3) a
step of drying the second molded body (step S130), and [0053] (4) a
step of impregnating the second molded body with Si and subjecting
the second molded body to reaction sintering to obtain a SiC--Si
composite member (step S140).
[0054] However, of these, step S130 is a step that is performed as
needed, and is not an essential step in the first production
method.
[0055] Hereinafter, each step will be described in more detail.
(Step S110)
[0056] In step S110, a first molded body containing SiC particles
is formed using a 3D printing method.
(3D Printing Method)
[0057] First, the 3D printing method used in the first production
method will be described.
[0058] The 3D printing method used in this step S110 is not
particularly limited. In step S110, for example, a 3D printing
method such as a "laser irradiation modeling method" or a "binder
jet modeling method" may be used.
[0059] Among these, in the laser irradiation modeling method, a
powder layer containing SiC particles and a binder is irradiated
with a laser. The binder in the irradiated area is melted by the
heat of the laser, then solidifies. As a result, each SiC particles
is bonded. Such procedure is applied to each powder layer which is
successively laminated so that a first molded body having a
three-dimensional structure is formed.
[0060] Alternatively, in the binder jet molding method, the binder
is sprayed from the inkjet nozzle to the powder layer. In the area
where the binder is sprayed, the SiC particles are bound each
other. This operation is repeated every time the powder layers are
laminated, so that the first molded body having a three-dimensional
structure is formed.
[0061] In the binder jet molding method, the powder layer may
contain a curing agent in advance, and the binder may be reacted
and cured only in an area where the sprayed binder and the curing
agent come into contact with each other. The content of the curing
agent may be, for example, about 0.1% by mass to 1% by mass with
respect to the powder layer.
[0062] In either molding method, a thermosetting resin such as a
phenol resin and a self-curing resin such as a furan resin can be
used as the binder.
[0063] The thickness of one powder layer used in the 3D printing
method is usually in the range of 200 .mu.m to 300 .mu.m.
(SiC Particles)
[0064] The average particle size of the SiC particles contained in
the powder layer may be, for example, in the range of 30 .mu.m to
200 .mu.m. The average particle diameter of the SiC particles is
preferably in the range of 50 .mu.m to 180 .mu.m.
[0065] The SiC particles are preferably .alpha.-SiC.
[0066] In general, the larger the average particle diameter of the
SiC particles becomes, the larger the average pore diameter
M.sub.1of the pores contained in the first molded body is formed.
Therefore, when the average pore diameter M.sub.1of the pores is
desired to be relatively small, SiC particles having a relatively
small average particle diameter may be selected. When the average
pore diameter M.sub.1 of the pores is desired to be relatively
large, SiC particles having a large average particle diameter may
be selected.
[0067] However, if the average particle size of the SiC particles
is too small, in the later step of S120, the pores of the first
molded body may not be sufficiently impregnated with the carbon
particles. Therefore, the average particle diameter of the SiC
particles is preferably 30 .mu.m or more.
[0068] Any powder such as granulated powder or spherical powder can
be used as the SiC particles.
[0069] The average particle size of the SiC particles can be
measured by a laser diffraction/scattering particle size
distribution measuring device (MT3300EXII manufactured by
MicrotracBEL Corp.).
(First Molded Body)
[0070] The first molded body produced by the 3D printing method is
usually a porous body and has a large number of pores.
[0071] The volume occupied by the SiC particles in the first molded
body is, for example, in the range of 30 vol % to 50 vol %. In
other words, the volume of the pores in the first molded body, that
is, the porosity may be in the range of 50 vol % to 70 vol %.
[0072] The bulk density of the first molded body is, for example,
in the range of 0.97 g/cm.sup.3 to 1.61 g/cm.sup.3.
[0073] The average pore diameter M.sub.1 of the pores of the first
molded body may be, for example, 20 .mu.m to 100 .mu.m and
particularly 30 .mu.m to 100 .mu.m. However, the average pore
diameter M.sub.1 of the pores of the first molded body may be
varied depending on the particle diameter of the SiC particles
used, the degree of compression of the powder layer, and the
like.
[0074] The porosity of the first molded body and the average pore
diameter M.sub.1 of the pores can be measured using the mercury
porosimeter as described above.
[0075] The size and shape of the first molded body produced by the
3D printing method are not particularly limited.
[0076] However, it should be noted that the first production method
can be applied to a large-sized molded body and/or a complex molded
body. In particular, the first production method can also produce a
molded body having a complex shape, which is difficult to produce
by the casting method.
(Step S120)
[0077] Next, in step S120, the pores of the first molded body are
impregnated with carbon particles to form a second molded body.
[0078] In this step, a dispersion in which carbon particles are
dispersed is used. That is, the pores can be impregnated with the
carbon particles contained in the dispersion by immersing the first
molded body in the dispersion or injecting the dispersion into the
first molded body.
[0079] In the former case, the impregnation treatment is preferably
carried out under reduced pressure. Pores can be efficiently
impregnated with a dispersion under reduced pressure environment.
Further, it is preferable to hold the first molded body in a
pressurized environment after once the pores of the first molded
body are impregnated with dispersion. This allows the dispersion to
be introduced even more into the pores of the first molded
body.
[0080] The concentration of carbon particles contained in the
dispersion is, for example, in the range of 20% by mass to 60% by
mass. The concentration of carbon particles is preferably high as
long as the carbon particles do not precipitate. Therefore, the
concentration of carbon particles is, for example, preferably in
the range of 30% by mass to 55% by mass.
[0081] The carbon particles are preferably nanoparticles or
aggregates of nanoparticles. For example, the average particle size
M.sub.2 of aggregated particles (secondary particles) of carbon
particles is in the range of 100 nm to 200 nm and preferably in the
range of 110 nm to 150 nm. Reducing the average particle size
M.sub.2 of the aggregated particles of carbon particles to less
than 100 nm is not practical because it is necessary to
significantly increase the content of the dispersant, and the
viscosity of the dispersion tends to increase. The average particle
size M.sub.2 of the aggregated particles of carbon particles of
larger than 200 nm is not preferably used, because the
dispersibility is deteriorated and the particles are apt to
precipitate.
[0082] The dispersion medium contained in the dispersion is not
particularly limited. The dispersion medium may include, for
example, water and/or alcohol.
[0083] Here, the carbon particles are selected so that the average
particle size M.sub.2 is 1/10 or less of the average pore size of
the pores present in the first molded body. That is,
M.sub.2.ltoreq.M.sub.1/10.
[0084] The above particle size, ensures that the carbon. particles
are introduced into the pores of the first molded. body. For
example, when the average particle diameter M.sub.2 of the carbon
particles is approximately the same as the average pore diameter
M.sub.1 of the pores present in the first molded body, it becomes
difficult to impregnate a sufficient amount of carbon into the
pores.
[0085] As a further example, the average particle diameter M.sub.2
of the carbon particles is preferably selected so that the average
particle diameter M.sub.2 of the carbon particles with respect to
the average pore diameter M.sub.1 of the pores of the first molded
body is M.sub.2.ltoreq.M.sub.1/30, M.sub.2.ltoreq.M.sub.1/50,
M.sub.2.ltoreq.M.sub.1/100, and M.sub.2.ltoreq.M.sub.1/200.
[0086] Instead of using the above-mentioned dispersion, the first
molded body may be impregnated with a carbon source such. as a
phenol resin or an epoxy resin and then heat-treated to generate
(precipitate) carbon from the carbon source.
[0087] However, such a method requires an additional reaction step
for producing (precipitating) carbon from the carbon source.
Further, in order to generate (precipitate) a sufficient amount of
carbon, it is necessary to repeat the process of impregnating the
carbon source and the process of carbon precipitation several
times. As a result, the production method becomes complicated.
Furthermore, especially when the carbon source is a solid, it is
difficult to introduce carbon into the pores of the first molded
body as intended by the carbon deposition reaction.
[0088] Thus, the method of using carbon sources and depositing
carbon from the carbon sources is problematic and, thus, is not
practical.
[0089] However, in the first production method, the pores of the
first molded body are impregnated with the carbon particles using a
dispersion containing the carbon particles. Therefore, in the first
production method, steps such as carbon deposition reaction can be
omitted, and the production method can be simplified.
[0090] The concentration of carbon particles contained in the
dispersion can be adjusted as appropriate. Therefore, the number of
impregnation steps with the dispersion can be minimized, and the
production method can be simplified. For example, when a dispersion
having a carbon particle concentration of 30% by mass or more is
used, one impregnation step is sufficient.
[0091] Further, in the first production method, a dispersion
containing carbon particles has a relationship between the average
pore diameter M.sub.1 and the average particle diameter M.sub.2 of
M.sub.2.ltoreq.M.sub.1/10. Therefore, many carbon particles can be
relatively easily introduced into each pore of the first molded
body.
[0092] Furthermore, in the first production method, carbon
particles having a relatively small average particle size are
introduced into the pores of the molded body. Such small carbon
particles have an advantage that the reaction surface area is
significantly increased and the reactivity can be increased in the
reaction step S140 of carbon particles and Si.
[0093] By the method as described above, a molded body in which
carbon particles are introduced into the pores, that is, a second
molded body can be formed.
(Step S130)
[0094] Next, if necessary, the second molded body is dried.
[0095] This step S130 is performed to ensure removal of the
dispersion medium contained in the second molded body.
[0096] The method of drying treatment is roughly classified into
two types, that is, "heat drying method" and "vacuum freeze-drying
method".
[0097] The heating and drying method is a drying method in which
the second molded body is heated to volatilize and remove the
dispersion medium. The heating temperature changes depending on the
type of dispersion medium. When the dispersion medium is water, the
heating temperature may be in the range of 100.degree. C. to
120.degree. C.
[0098] On the other hand, in the vacuum freeze-drying method,
first, the second molded body placed in the drying chamber is
cooled and frozen. As a result, the dispersion contained in the
second molded body is also frozen. The cooling temperature is set
to a temperature equal to or lower than the temperature at which
the dispersion medium freezes. For example, when the dispersion
medium contains water, the cooling temperature may be in the range
of -5.degree. C. to -50.degree. C.
[0099] Then, the dispersion medium is sublimated and removed by
vacuum-exhausting the drying chamber to dry the second molded
body.
[0100] In the heating and drying method, when the vaporization of
the dispersion medium in the pores gradually progresses, the
remaining dispersion medium moves to the pore walls forming the
pores, passes through the state of adhering to the pore walls, and
is then completely vaporized. Since the carbon particles also
follow such a liquid flow, the carbon particles tend to be
agglomerated and segregated on the pore walls after the drying
treatment.
[0101] On the other hand, in the vacuum freeze-drying method, the
dispersion medium is sublimated and removed in a small movement
within the pores. Therefore, in the vacuum freeze-drying method,
the dispersion medium can be removed while maintaining the
distribution state of the carbon particles in the pores before the
drying treatment. Therefore, aggregation of carbon particles is
unlikely to occur.
[0102] As described above, step S130 is a step that is performed as
necessary and does not necessarily need to be actively performed.
For example, the second molded body may be naturally dried.
(Step S140)
[0103] Next, in step S140, a SiC--Si composite component is
produced from the dried second molded body.
[0104] In addition, before this step S140, a process of sintering
the second molded body (hereinafter, referred to as "pre-sintering
process") may be performed.
[0105] By performing the pre-sintering treatment, at least some of
the SiC particles are sintered and bonded to each other in the
second molded body, and the shape can be further stabilized.
Further, it seems that the mechanical strength of the SiC--Si
composite component finally obtained (that is, after step S140) can
be further increased.
[0106] The conditions of the pre-sintering treatment, that is, the
treatment temperature, the treatment time, the treatment
environment and the like are not particularly limited as long as at
least a part of the SiC particles is sintered and bonded.
[0107] The pre-sintering process is not always a necessary process
and may be omitted.
[0108] Next, the second molded body is impregnated with metallic
silicon (Si).
[0109] Further, a part of the impregnated metallic Si reacts with
the carbon particles contained in the pores of the second molded
body generates silicon carbide (SiC). Further, the SiC particles
included in the second molded body and the SiC newly generated in
this step S140 are mutually sintered to form a sintered body.
Hereinafter, these processes will be collectively referred to as
"reactively sintering process".
[0110] Note that, the portion that did not react with the carbon
particles among the introduced metal Si remains as it is.
Therefore, the SiC--Si composite component is formed after the
reaction sintering process.
[0111] Here, as described above, the pores of the second molded
body are impregnated with carbon particles having a relatively
small average particle size. Therefore, the reaction between the
carbon particles and Si, that is, the reaction for producing SiC,
proceeds relatively quickly in the reaction sintering process. As a
result, the sintering reaction between some SiC is also
accelerated.
[0112] The method of impregnating the second molded body with
metallic Si in step S140 is not particularly limited. For example,
the second molded body and the metallic Si are heated and the
metallic Si may be molten in a state where the second molded body
and the metallic Si are in contact with each other. In this case,
the pores of the second molded body are impregnated with the molten
metallic Si by the capillary phenomenon. Further, when the metallic
is molten in a state where the metallic Si is placed on the upper
part of the second molded body, the effect of gravity is further
utilized to impregnate the pores of the second molded body with the
molten metallic Si.
[0113] When such a method is adopted, the heating temperature of
the second molded body and the metallic Si (hereinafter
collectively referred to as "body to be heated") may be equal to or
higher than the melting point of the metallic Si. For example, the
heating temperature is preferably in the range of the melting point
of metallic Si to 1650.degree. C. The melting point of metallic Si
is approximately 1410.degree. C. to 1414.degree. C., although the
melting point of metallic Si varies slightly depending on the
measuring method.
[0114] When the heating temperature of the body to be heated is
adjusted in the range of the melting point of metallic Si to
1650.degree. C., the reaction sintering process can be performed
simultaneously with the impregnation of the molten metallic Si into
the second molded body.
[0115] Tn the above method, the processing environment of the body
to be heated is preferably a reduced pressure environment.
[0116] The second molded body may be impregnated with metallic Si
using a different method.
[0117] The amount of metallic Si introduced into the second molded
body is determined based on the amount of carbon particles
contained in the second molded body and the Si concentration
contained in the finally obtained SiC--Si composite component.
[0118] For example, the amount of Si required for the sintering
reaction of SiC, which is calculated from the amount of carbon
particles contained in the second compact, is R.sub.1. The amount
of required for the sintering reaction of SiC, which is calculated
from the Si concentration in the metallic state in the SiC--Si
composite component is R.sub.2. The amount R of metallic Si, which
is introduced into the second molded body, is determined by
R=R.sub.1+R.sub.2.
[0119] Through the above steps, the SiC--Si composite component can
be produced.
[0120] The content of Si contained in the obtained SiC--Si
composite component is in the range of 5% by mass to 40% by mass,
and preferably in the range of 8% by mass to 30% by mass, based on
the total weight of the SiC--Si composite component. In other
words, the content of SiC contained in the SiC--Si composite
component is in the range of 60% by mass to 95% by mass, and in the
range of 70% by mass to 92% by mass, based on the total weight of
the SiC--Si composite component. When the content of Si contained
in the SiC--Si composite component is less than 5% by mass, the
impregnation operation of the dispersion of the carbon particles
into the first molded body is required for several times, thereby
the process becomes complicated and unfavorable. When the content
of Si contained in the SiC--Si composite member exceeds 40% by
mass, the mechanical strength of the SiC--Si composite becomes low
and unfavorable.
[0121] According to the first production method, a SiC--Si
composite component having a small porosity and a high bulk density
can be produced. For example, the porosity of the SiC--Si composite
component is in the range of 0 vol % to 3 vol %. The bulk density
of the SiC--Si composite component is in the rage of 2.79
g/cm.sup.3 to 3.16 g/cm.sup.3.
[0122] Therefore, according to the first production method, a
SiC--Si composite component having good mechanical strength can be
obtained. The 4-point bending strength of the SiC--Si composite
component may be 100 MPa or more, for example 190 MPa or more.
[0123] In the present application, the 4-point bending strength is
a value measured by the method specified in JIS R1601 (test method
for bending strength of fine ceramics).
[0124] Further, a SiC--Si composite component having a complex
shape and/or a large-sized shape can be produced in the first
production method.
[0125] Heretofore, one embodiment of the present invention has been
described by referencing the first production method as an example.
However, it is obvious to a person skilled in the art that the
production method according to the present invention is not limited
to the first production method. For example, each step included in
the first production method may be modified within the scope of the
present invention.
(SiC--Si Composite Component According to One Embodiment of the
Present Invention)
[0126] Hereinafter, an example of the SiC--Si composite component
according to one embodiment of the present invention will be
described in detail with reference to FIG. 2.
[0127] FIG. 2 shows an example of a micrograph of a SiC--Si
composite component (hereinafter referred to as "first composite
component") according to one embodiment of the present
invention.
[0128] As shown in FIG. 2, the microstructure of the first
composite component has a so-called "sea-island structure" in which
metallic Si as "sea portion" and .alpha.-SiC particles and
.beta.-SiC particles as "island portion" are mixed.
[0129] More specifically, in the microstructure of FIG. 2, the "sea
portion" that appears white is metallic Si, and the large "island
portion" that appears black is .alpha.-SiC particles. In addition,
small black "island portion" dispersed in the "sea portion" of
metallic Si are .beta.-SiC particles.
[0130] It should be noted that it can be determined by Raman
microspectroscopy whether each part of the microstructure is
.alpha.-SiC particles, .beta.-SiC particles or metallic Si. That
is, in the case of .alpha.-SiC particles, large peaks occur at the
respective positions of 766 cm.sup.-1, 788 cm.sup.-1 and 797
cm.sup.-1 in the Raman spectrum. Further, in the case of .beta.-SiC
particles, a large peak appears at the position of 796 cm.sup.-1 in
the Raman spectrum. In contrast, a large peak occurs at the
position of 521 cm.sup.-1 in the case of metallic Si.
[0131] In this way, each part of the microstructure can be
identified.
[0132] Hereinafter, the portion of .alpha.-SiC particles is
referred to as a "first island portion", and the portion of
.beta.-SiC particle is also referred to as a "second island
portion" in the microstructure of the first composite
component.
[0133] The first island portion, that is, the .alpha.-SiC
particles, has an average particle diameter in the range of 30
.mu.m to 200 .mu.m. The .alpha.-SiC particles, for example, may
have an average particle size in the range of 50 .mu.m to 180
.mu.m.
[0134] In contrast, the second island portion, that is, .beta.-SiC
particles, has an average particle size in the range of 1 .mu.m to
20 .mu.m. However, when the second island portion is observed in
detail in FIG. 2, the .beta.-SiC particles are not necessarily
separated from each other in the first composite component. That
is, at least some of the second island portions in FIG. 2 are
connected to each other.
[0135] The average particle size of .alpha.-SiC particles in the
microstructure can be obtained from a micrograph. That is, 200 or
more of .alpha.-SiC particles having a major axis of 30 .mu.m or
more are selected, and the average value of the major axis and the
minor axis of each particle is calculated. Next, the average
particle size of the .alpha.-SiC particles is obtained by averaging
the obtained average values for all the selected .alpha.-SiC
particles. Image analysis software WinROOF2015 is used for the
measurement.
[0136] The average particle size of p-sic particles can be
similarly obtained from the micrograph. In this case, 200 or more
of .beta.-SiC particles having a major axis of 30 .mu.m or less are
selected from the micrograph. After that, the equivalent circle
diameter of each particle is calculated using the image analysis
software WinROOF 2015, and the average particle diameter of the
.beta.-SiC particles is obtained by averaging all the selected
.beta.-SiC particles.
[0137] In the first composite component, the total content of
.alpha.-SiC particles and .beta.-SiC particles is, for example, 60%
by mass or more and 95% by mass or less. In contrast, the content
of metallic Si is, for example, 5% by mass or more and 40% by mass
or less in the first composite component. In this case, the first
composite component can be produced easily and the component with
high strength can be easily obtained.
[0138] The total content of .alpha.-SiC particles and .beta.-SiC
particles is preferably 70% by mass or more and 90% by mass or
less. The content of metallic Si is preferably 10% by mass or more
and 30% by mass or less.
[0139] In the first composite component, the ratio A of the volume
of the .alpha.-SiC particles to the volume of the .beta.-SiC
particles, that is, A=(volume of .alpha.-SiC)/(volume of
.beta.-SiC) is 0.5 to 10.
[0140] In addition, in order to reduce ratio A to 0.5 or less, the
concentration of carbon particles in the dispersion needs to be
high. However, in such a case, the viscosity of the dispersion
increases. As a result, the pores of the first molded body may be
hardly impregnated with the carbon particles. Further, in order to
increase the ratio A to 10 or more, the porosity of the first
molded body is need to be small, but it is not practical because
the porosity of the molded body is controlled by the bulk density
of .alpha.-SiC particles.
[0141] The ratio A is preferably in the range of 0.7 to 3.
[0142] In contrast, in the first composite member, the ratio B of
the volume of .beta.-SiC particles to the volume of metallic Si,
that is, B=(volume of .beta.-SiC)/(volume of metallic Si) is 0.4 to
12. In this case, the dispersibility of the carbon particles is
stabilized during the production of the first composite component.
The ratio B is preferably in the range of 0.4 to 4.
[0143] It should be noted that both the ratio A and the ratio B can
be obtained by performing image processing on the microstructure in
the cross-section of the first composite component to obtain the
area ratio. That is, because the first composite component has a
relatively homogeneous structure in the three-dimensional
direction, the volume ratio can be directly calculated from the
obtained area ratio.
[0144] In the present application, the above area ratios were
measured in the cross-sections of five different microstructures,
and the ratio A and the ratio B were calculated from the five
averages obtained from the measurements.
[0145] Image analysis software WinROOF2015 was used for the image
processing.
[0146] Specifically, this software was used to perform monochrome
processing of the microstructure photograph image and then perform
automatic binarization processing to calculate the SiC area and the
Si area.
[0147] In addition, particles having a particle size of 30 .mu.m or
more were selected from the structure photograph image, the
calculated area was taken as the .alpha.-SiC area, and the value
obtained by subtracting the .alpha.-SiC area from the
above-mentioned SiC area was taken as the .beta.-SiC area. Using
these areas, the ratio A and the ratio B were calculated.
[0148] Further, when the microstructure of the cross-section of the
first composite component is photographed at a magnification of 20
times, the P value obtained by the following procedure is
preferably 3 or more.
<How to Obtain P Value>
[0149] P value is obtained by the following: [0150] (i) In the
second island portion of the microstructure, a circle Z is drawn
having a diameter of 200 .mu.m with the center X of any .beta.-SiC
particle in a region formed by connecting a plurality of .beta.-SiC
particles to each other. For example, FIG. 3 shows an example of a
circle Z drawn on the microstructure by such operation. [0151] (ii)
Next, a first straight line having a length of 200 .mu.m and
passing through the center X is drawn in the circle Z. For example,
FIG. 4 shows an example of the first straight line L drawn in the
circle Z by such operation. [0152] (iii) In the circle Z, a second
straight line having a length of 200 .mu.m and having a rotation
angle of 1.degree. with respect to the first straight line L with
the center X as the central axis is drawn. [0153] (iv) The same
operation as (iii) is repeated until the rotation angle is
180.degree., and a total of 180 straight lines are drawn in the
circle Z. [0154] (v) In each straight line, length of the line
segment of one or a plurality of lines which across "the sea
portion" of the metal Si is measured. An average value S.sub.ave of
the length of segments in each straight line is calculated. As a
result, the average value S.sub.ave corresponding to each of the
180 straight lines is obtained. [0155] (vi) The ratio P is
calculated by selecting a straight line in which the average value
S.sub.ave becomes maximum from the 180 straight lines and set as
maximum S.sub.max, selecting a straight line in which the average
value S.sub.ave becomes minimum from the 180 straight lines and set
as minimum S.sub.min, and calculating the ratio P by the following
formula:
[0155] P=S.sub.max/S.sub.min (1).
[0156] The operation (v) can be statistically performed by using
image analysis software (for example, WinROOF2015).
[0157] The P value obtained in this way can be an index showing the
degree of connection between the second island portions in the
first composite component. That is, the higher the P value, the
longer the second island portions are connected in a row.
[0158] Further, when the P value is 3 or more, many .beta.-SiC
particles are connected to each other in the second island portion,
and thus such a first composite component tends to have high
strength. For example, the first composite component has a strength
of 190 MPa or more as determined by a 4-point bending test (JIS
R1601 (test method of bending strength for fine ceramics at room
temperature)).
[0159] Furthermore, the first composite component may have a
thermal conductivity of 200 W/mK or more. The thermal conductivity
is, for example, 220 W/mK or more. In the first composite
component, when many .beta.-SiC particles are interconnected in the
second island portion, high thermal conductivity is obtained.
[0160] The first composite component having the above-described
microstructure can be obtained, for example, when the (trying step
(step S130) is carried out by the freeze-drying method in the
above-mentioned first production method.
[0161] As described above, when the molded body containing the
carbon particles in the pores is dried by the freeze-drying method,
the aggregation of the carbon particles in the pores is
significantly suppressed. Therefore, in the subsequent reaction
sintering process, the carbon particles having a relatively small
average particle diameter are reacted and sintered with the
metallic Si, and fine .beta.-SiC particles can be generated.
EXAMPLES
[0162] Examples will be described below. In the following
description, Examples 1 to 3 and 6 are Examples, and Examples 4 to
5 are Comparative Examples.
Example 1
[0163] The SiC--Si composite component was produced according to
the above-described first production method as follows.
[0164] First, the first molded body was prepared by the 3D printing
method.
[0165] As the 3D printing method, the "binder jet molding method"
was adopted, and the binder was injected from the inkjet nozzle
toward the powder layer. A phenol resin was used as the binder.
[0166] The powder layer was a mixture of SiC particles and a curing
agent. The content of the curing agent was about 0.1% by mass. As
the SiC particles, .alpha.-SiC powder (manufactured by Shinano
Electric Refining Co., Ltd.) having an average particle diameter of
80 .mu.m was used. The thickness of each powder layer was about 200
.mu.m, and the binder injection was repeated each time each powder
layer was laminated.
[0167] Accordingly, a first molded body having a length of 40 mm, a
width of 20 mm, and a height of 10 mm in a shape of rectangular
parallelepiped was prepared.
[0168] The bulk density of the first molded body was 1.14
g/cm.sup.3. The porosity of the first molded body was 64.6%. The
average pore diameter M.sub.1 of the pores of the first molded body
was 46.9 .mu.m.
[0169] Next, a second molded body was formed from the first molded
body.
[0170] First, the first molded body was immersed in the dispersion
containing carbon particles.
[0171] The concentration of carbon particles in the dispersion was
40% by mass, and the carbon particles were nanoparticles. The
average particle diameter M.sub.2 of the secondary particles of the
carbon particles was about 120 nm. Therefore, M.sub.2/M.sub.1 was
about 0.00256. The dispersion medium was water.
[0172] The first molded body was immersed in the dispersion under a
reduced pressure environment. As a result, the pores of the first
molded body were impregnated with the carbon particles, and the
second molded body was formed.
[0173] Then, the second molded body was dried. A vacuum
freeze-drying method was used for drying. Specifically, the second
molded body was allowed to pass through the range of 0.degree. C.
to -10.degree. C. in less than 20 minutes so that the water content
of the second molded body was sublimated by freezing the second
molded body followed by vacuum-exhausting the second molded
body.
[0174] The bulk density of the second molded body was 1.37
g/cm.sup.3 and the porosity was 53.2%.
[0175] Moreover, when the concentration of the carbon particles
contained in the second molded body was measured using a thermal
analysis method, the content of the carbon particles with respect
to SiC in the molded body was 20% by mass.
[0176] Next, the second molded body was impregnated with metallic
Si.
[0177] For the impregnation treatment with metallic Si, the body to
be heated constituted by placing metallic Si on the second molded
body was placed in the reaction furnace. The amount of metallic Si
was 12.7 g.
[0178] Next, the body to be heated was heated to 1550.degree. C. in
a state where the inside of the reaction furnace was in a reduced
pressure environment.
[0179] By this treatment, the metallic Si was molten, and the
second molded body was impregnated with the molten material. At the
same time, the reactive sintering process proceeded. That is, SiC
was formed by the reaction between the carbon in the pores and the
impregnated Si, and a sintering reaction was occurred between the
contained in the second molded body which also containing these
newly generated SiC.
[0180] As a result, a sintered body (SiC--Si composite component)
was formed.
[0181] The sintered body (hereinafter, referred to as "sintered
body according to Example 1") was maintained as a rectangular
parallelepiped having a length of 40 mm, a width of 20 mm, and a
height of 10 mm.
Example 2
[0182] A sintered body (hereinafter referred to as "sintered body
according to Example 2") was produced by the same method as in
Example 1.
[0183] However, in this Example 2, the concentration of carbon
particles in the dispersion was 50% by mass. The average particle
size M.sub.2 of the secondary particles of the carbon particles was
about 130 nm. Other conditions were the same as in the case of
Example 1.
Example 3
[0184] A sintered body (hereinafter referred to as "sintered body
according to Example 3") was produced by the same method as in
Example 1.
[0185] However, in this example 3, the average particle diameter of
the SiC particles was 150 .mu.m. Other conditions were the same as
in the case of Example 1.
Example 4
[0186] A sintered body (hereinafter referred to as "sintered body
according to Example 4") was produced by the same method. as in
Example 1.
[0187] However, in this Example 4, the step of immersing the first
molded body in the dispersion containing carbon particles was not
performed. That is, the steps after the impregnation of the
metallic Si using the first molded body were performed. Further,
the first molded body was naturally dried before being subjected to
the impregnation treatment with metallic Si.
Example 5
[0188] A sintered body (hereinafter referred to as "sintered body
according to Example 5") was produced by the same method as in
Example 1.
[0189] However, in Example 5, the average particle size of the SiC
particles was set to 1 .mu.m. Other conditions were the same as in
the case of Example 1.
[0190] Table 1 below collectively shows the conditions used for
producing the sintered bodies according to the respective
examples.
TABLE-US-00001 TABLE 1 Average Content partcle of C Average diamter
particles Bulk pore M.sub.2 of C Bulk based on Average density
Porosity size M.sub.1 particles density Porosity SiC in Drying
particle of first of first of first C partcle (agglomerated of
second of second second method size of molded molded molded
concentration particles) in molded molded molded of second
.alpha.-SiC body body body in dispersion dispersion body body body
(% molded Examples (.mu.m) (g/cm.sup.3) (%) (.mu.m) (% by mass)
(nm) M.sub.2/M.sub.1 (g/cm.sup.3) (%) by mass) body 1 80 1.14 64.6
46.9 40 120 0.00256 1.37 53.2 20 vacuum freeze drying 2 80 1.14
64.6 46.9 50 120 0.00256 1.41 50.9 24 vacuum freeze drying 3 150
1.18 63.4 75.1 40 120 0.0016 1.39 52.7 18 vacuum freeze drying 4 80
1.14 64.6 46.9 -- -- -- -- -- -- Natural drying 5 1 1.06 66.5 0.6
40 120 0.2 1.11 64.8 3 vacuum freeze drying
(Evaluation)
[0191] The following evaluations were performed using the sintered
bodies according to the above-mentioned Examples 1 to 5.
(Physical Property Value)
[0192] The physical properties of each sintered body were measured.
Specifically, the amount of unreacted metallic Si contained in each
sintered body, the bulk density, and the porosity were
measured.
[0193] The measurement results are shown in Table 2 below.
TABLE-US-00002 TABLE 2 Amount of metallic Si Bulk density Porosity
Examples (% by mass) (g/cm.sup.3) (%) 1 33.4 2.86 0.2 2 29.1 2.92
0.8 3 33.8 2.85 0.4 4 56.9 2.65 0.1 5 55.3 -- --
[0194] As a result of the measurement, the sintered body according
to Example 4 had a bulk density of 2.65 g/am.sup.3, which was not
so high.
[0195] On the other hand, the sintered bodies according to Examples
1 to 3 all have a bulk density of 2.85 g/cm.sup.3 or more, and have
a high bulk density. In addition, the porosity was less than 3 vol
%.
[0196] In addition, the sintered body according to Example 5 had
poor unifomity. Therefore, various evaluations were not
performed.
(Strength)
[0197] The strengths of the sintered bodies according to Examples 1
to 3 were evaluated by a 4-point bending test.
[0198] The 4-point bending test was performed by cutting out a
sample from each sintered body. The size of the sample was 36 mm in
length.times.4 mm in width.times.3 mm in thickness. The 4-point
bending test was carried out in accordance with JIS 81601 (test
method of bending strength for fine ceramics at room
temperature).
[0199] The measurement results are shown in Table 3 below.
TABLE-US-00003 TABLE Examples Bending strength (MPa) 1 206 2 259 3
192 4 48 5 --
[0200] As a result of the measurement, the 4-point bending strength
of each of the sintered bodies was 192 MPa or more, and each
sintered body had good strength.
[0201] As described above, the SiC--Si composite produced by using
the method according to the embodiment of the present invention had
high density and had favorable mechanical strength.
Example 6
[0202] A sintered body (hereinafter referred to as "sintered body
according to Example 6") was produced by the same method. as in
Example 2.
[0203] However, in Example 6, the heating temperature of the body
to be heated in the reactive sintering process was 1450.degree. C.
That is, the body to be heated, which was reconfigured by placing
metallic Si on the second molded body, was heated to 1450.degree.
C. in the reaction furnace. Other conditions were the same as in
the case of Example 2.
(Evaluation)
[0204] The following evaluation was performed using the sintered
body according to Example 6.
(Organizational Evaluation)
[0205] The structure was observed on the cross-section obtained by
cutting the sintered body according to Example 6.
[0206] FIG. 2 described above shows a micrograph of the structure
of the cross-section.
[0207] From FIG. 2, the structure of the sintered body according to
Example 6 has a sea-island structure.
[0208] Raman microspectroscopic measurement was performed on the
obtained cross-sectional structure. As a result, .alpha.-SiC,
.beta.-SiC, and metallic Si were contained in the sintered body
according to Example 6.
[0209] FIGS. 5 to 8 show mapping diagrams obtained by Raman
microspectroscopic measurement. Note that FIG. 5 is an optical
micrograph of the evaluation target area. FIG. 6 is a mapping
pattern of Si, FIG. 7 is a mapping pattern of .alpha.-SiC, and FIG.
8 is a mapping pattern of .beta.-SiC.
[0210] From comparison between FIGS. 5 to 8 and FIG. 2, the large
first island portion is .alpha.-Si particles in the sea-island
structure. Further, the small second island portion dispersed. in
the sea portion of metallic Si is formed from .beta.-Si
particles.
[0211] As a result of the measurement, the average particle size of
the .alpha.-Si particles was 56 .mu.m, and the average particle
size of the .beta.-Si particles was 7 .mu.m.
[0212] Next, the P value was calculated by the above operation in
the sintered body according to Example 6. WinROOF2015 was used as
software.
[0213] FIG. 3 and FIG. 4 described above respectively show the
circle Z and the first straight line L obtained in the course of
the operation of calculating the P value.
[0214] FIG. 9 shows a plot of the average value S.sub.ave measured
on each of the 180 straight lines drawn in the circle Z. As
described above, the average value S.sub.ave is the average value
obtained by averaging the respective lengths of the line segments
when the target straight line across the portion of metallic
Si.
[0215] In FIG. 9, the horizontal axis indicates the rotation angle
of each straight line with respect to the first straight line.
Therefore, the range of the horizontal axis is 0.degree. (first
straight line L) to 179.degree.. The vertical axis represents the
average value S.sub.ave.
[0216] From FIG. 9, the maximum S.sub.max was 8.7 .mu.m and the
minimum S.sub.min was 1.9 .mu.m in this operation. Therefore, the P
value obtained from the following formula (1) was 4.6.
P=S.sub.max/S.sub.min (1)
[0217] Next, the above-mentioned ratio A (=volume of
.alpha.-SiC/volume of .beta.-Sic) and ratio B (volume of
.beta.-SiC/volume of metallic Si) were calculated by the image
processing from the cross-section of the obtained
microstructure.
[0218] In Table 4 below, the measurement results of the area ratios
obtained from the five cross-sectional structures are shown.
TABLE-US-00004 TABLE 4 No. 1 No. 2 No. 3 No. 4 No. 5 Average value
Si area ratio 0.25 0.25 0.25 0.24 0.26 -- .alpha.-SiC area 0.37
0.38 0.37 0.33 0.31 -- ratio .beta.-SiC area 0.38 0.37 0.38 0.43
0.43 -- ratio (.alpha.-SiC area 0.96 1.01 1.00 0.77 0.72 0.88
ratio)/(.beta.-SiC area ratio) (.beta.-SiC area 1.53 1.49 1.50 1.79
1.65 1.60 ratio)/(Si area ratio)
[0219] From the five measurement results, the average value of
(area ratio of .alpha.-SiC)/(area ratio of .beta.-SiC), that is,
the ratio A was 0.88. Further, the average value of (.beta.-SiC
area ratio)/(Si area ratio), that is, the ratio B was 1.60.
[0220] In the sintered body of Example 6, the total content of
.alpha.-SiC particles and .beta.-SiC particles was 82% by mass.
(Strength)
[0221] The strength of the sintered body according to Example 6 was
evaluated by a 4-point bending test. As a result, the strength was
280 MPa.
(Thermal Conductivity)
[0222] The thermal conductivity of the sintered body according to
Example 6 was evaluated by the xenon flash method. As a result, the
thermal conductivity at 25.degree. C. was 226 W/mK.
* * * * *