U.S. patent application number 16/775424 was filed with the patent office on 2021-02-04 for non-volatile memory device and operating method of the same.
This patent application is currently assigned to Samsung Electronics Co. Ltd.. The applicant listed for this patent is Samsung Electronics Co. Ltd.. Invention is credited to Youngjin CHO, Jinhong KIM, Seyun KIM, Soichiro MIZUSAKI, Jungho YOON.
Application Number | 20210035641 16/775424 |
Document ID | / |
Family ID | 1000004640796 |
Filed Date | 2021-02-04 |







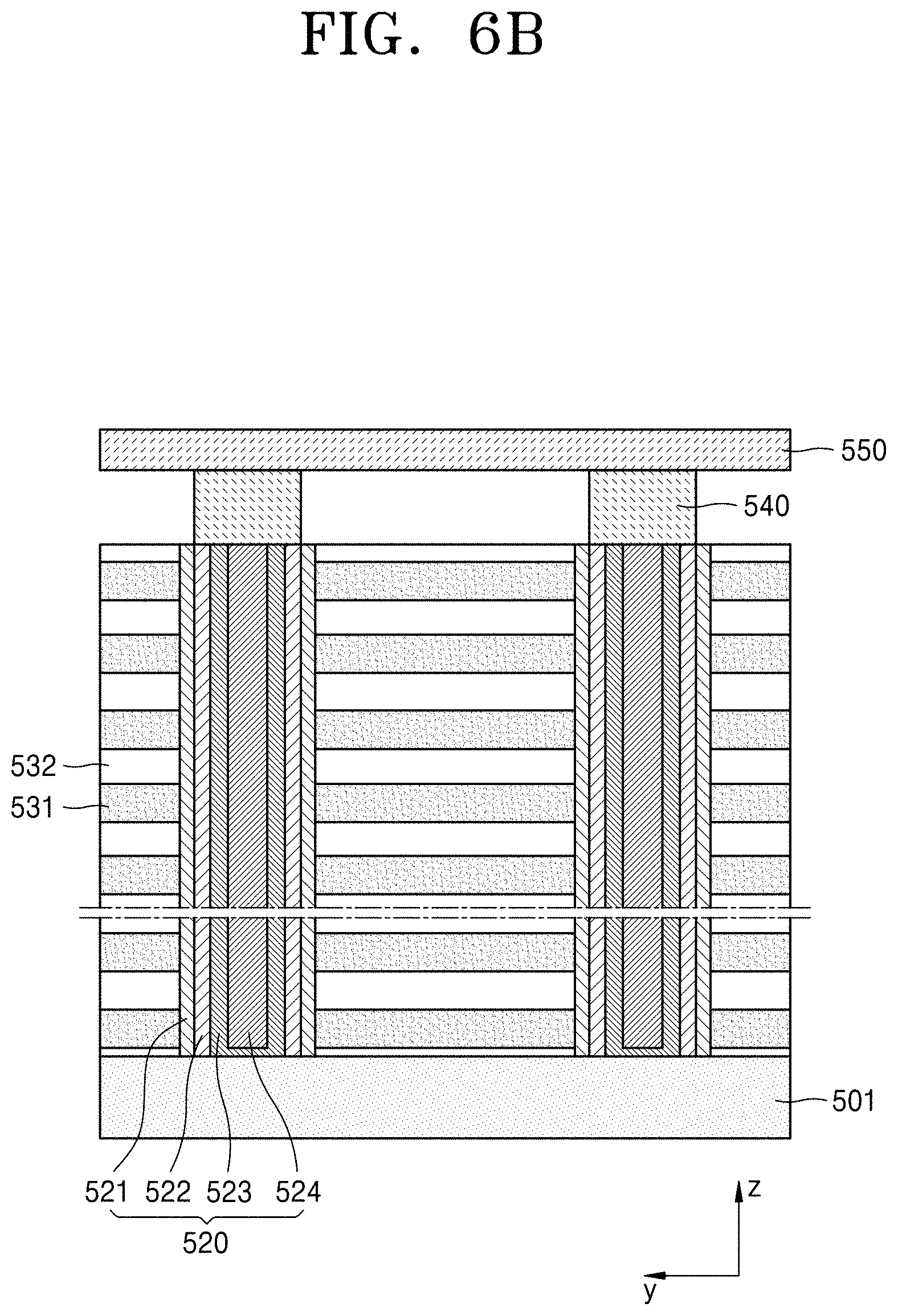



View All Diagrams
United States Patent
Application |
20210035641 |
Kind Code |
A1 |
YOON; Jungho ; et
al. |
February 4, 2021 |
NON-VOLATILE MEMORY DEVICE AND OPERATING METHOD OF THE SAME
Abstract
Provided are a non-volatile memory device and an operating
method thereof. The non-volatile memory device includes a memory
cell array having a vertically stacked structure, a bit line for
applying a programming voltage to the memory cell array, and a
control logic. The memory cell array includes memory cells that
each include a corresponding portion of a semiconductor layer and a
corresponding portion of a resistance layer. The memory cells
include a non-selected memory cell, a compensation memory cell, and
a selected memory cell. The control logic is configured to apply an
adjusted program voltage to the selected memory cell, based on
applying a first voltage to the compensation memory cell, a second
voltage to the selected memory cell, and a third voltage to the
non-selected memory cell. The adjusted program voltage may be
dropped compared to the programming voltage due to the compensation
memory cell.
Inventors: |
YOON; Jungho; (Yongin-si,
KR) ; KIM; Seyun; (Seoul, KR) ; KIM;
Jinhong; (Seoul, KR) ; MIZUSAKI; Soichiro;
(Suwon-si, KR) ; CHO; Youngjin; (Suwon-si,
KR) |
|
Applicant: |
Name |
City |
State |
Country |
Type |
Samsung Electronics Co. Ltd. |
Suwon-si |
|
KR |
|
|
Assignee: |
Samsung Electronics Co.
Ltd.
Suwon-si
KR
|
Family ID: |
1000004640796 |
Appl. No.: |
16/775424 |
Filed: |
January 29, 2020 |
Current U.S.
Class: |
1/1 |
Current CPC
Class: |
G11C 16/0483 20130101;
G11C 16/26 20130101; G11C 16/24 20130101; G11C 16/10 20130101; H01L
27/11582 20130101 |
International
Class: |
G11C 16/10 20060101
G11C016/10; H01L 27/11582 20170101 H01L027/11582; G11C 16/24
20060101 G11C016/24 |
Foreign Application Data
Date |
Code |
Application Number |
Jul 30, 2019 |
KR |
10-2019-0092660 |
Claims
1. A non-volatile memory device comprising: a memory cell array
having a vertically stacked structure, the memory cell array
including a semiconductor layer and a variable resistance layer,
the memory cell array including a plurality of memory cells that
each include a corresponding portion of the semiconductor layer and
a corresponding portion of the variable resistance layer, and the
plurality of memory cells including a non-selected memory cell, a
compensation memory cell, and a selected memory cell; a bit line
configured to apply a programming voltage to the memory cell array;
and a control logic configured to apply an adjusted program voltage
to the selected memory cell, the adjusted program voltage being
dropped compared to the programming voltage due to the compensation
memory cell, based on applying a first voltage to the compensation
memory cell, a second voltage to the selected memory cell, and a
third voltage to the non-selected memory cell, the first voltage
allowing an electric current of a certain magnitude to flow to the
compensation memory cell, the second voltage allowing the electric
current to flow only to the corresponding portion of the variable
resistance layer of the selected memory cell, and the third voltage
allowing the electric current to flow only to the semiconductor
layer of the non-selected memory cell.
2. The non-volatile memory device of claim 1, wherein an absolute
value of the first voltage is greater than an absolute value of the
second voltage.
3. The non-volatile memory device of claim 1, wherein an absolute
value of the first voltage is less than an absolute value of the
third voltage.
4. The non-volatile memory device of claim 1, wherein a magnitude
of the first voltage allows a resistance of the corresponding
portion of the semiconductor layer in the compensation memory cell
to be less than a resistance of the corresponding portion of the
variable resistance layer in the compensation memory cell.
5. The non-volatile memory device of claim 1, wherein a resistance
of the corresponding portion of the semiconductor layer in the
compensation memory cell is less than or equal to 1/10 of a
resistance of the corresponding portion of the variable resistance
layer in the compensation memory cell.
6. The non-volatile memory device of claim 1, wherein a magnitude
of the first voltage allows a resistance of the corresponding
portion of the semiconductor layer in the compensation memory cell
to be within a range of 10.sup.5 .OMEGA.m.sup.-1 to 10.sup.7
.OMEGA.m.sup.-1, based on the control logic applying the first
voltage to the compensation memory cell.
7. The non-volatile memory device of claim 1, wherein a magnitude
of the first voltage allows a resistance of the corresponding
portion of the variable resistance layer in the compensation memory
cell to be within a range of 10.sup.8 .OMEGA.m.sup.-1 to 10.sup.11
.OMEGA.m.sup.-1, based on the control logic applying the first
voltage to the compensation memory cell.
8. The non-volatile memory device of claim 1, wherein the
compensation memory cell and the selected memory cell are connected
to each other in series.
9. The non-volatile memory device of claim 1, wherein the control
logic is configured to make the electric current of the certain
magnitude flow to the corresponding portion of the variable
resistance layer in the selected memory cell during a program
operation.
10. The non-volatile memory device of claim 1, wherein the
corresponding portion of the semiconductor layer in the
compensation memory cell and the corresponding portion of the
variable resistance layer in the compensation memory cell are
connected to each other in parallel.
11. The non-volatile memory device of claim 1, wherein the memory
cell array includes: the semiconductor layer extending in a first
direction; a plurality of gates and a plurality of insulators
extending in a second direction perpendicular to the first
direction, the plurality of gates and the plurality of insulators
being alternately arranged; a gate insulating layer extending in
the first direction among the plurality of gates, the plurality of
insulators, and the semiconductor layer; and the variable
resistance layer extending in the first direction on the
semiconductor layer.
12. The non-volatile memory device of claim 11, wherein the
variable resistance layer is in contact with the semiconductor
layer.
13. The non-volatile memory device of claim 11, wherein the
variable resistance layer is spaced apart from the gate insulating
layer with the semiconductor layer therebetween.
14. The non-volatile memory device of claim 1, wherein the variable
resistance layer has a hysteresis characteristic.
15. The non-volatile memory device of claim 1, wherein the variable
resistance layer includes a transition metal oxide, a transition
metal nitride, or both the transition metal oxide and the
transition metal nitride.
16. The non-volatile memory device of claim 1, wherein the
compensation memory cell is a first compensation memory cell, the
plurality of memory cells further include a second compensation
memory cell, and the first compensation memory cell and the second
compensation memory cell that are each connected to the selected
memory cell in series.
17. An operating method of a non-volatile memory device including a
memory cell array having a vertically stacked structure, the memory
cell array including a semiconductor layer and a variable
resistance layer, the memory cell array including a plurality of
memory cells that each include a corresponding portion of the
semiconductor layer and a corresponding portion of the variable
resistance layer, the method comprising: applying a first voltage
to a compensation memory cell among the plurality of memory cells,
the first voltage allowing an electric current of a certain
magnitude to flow to the corresponding portion of the compensation
memory cell in the memory cell array; applying a second voltage to
a selected memory cell among the plurality of memory cells, the
second voltage allowing the electric current to flow only to the
corresponding portion of the variable resistance layer in the
selected memory cell of the memory cell array; applying a third
voltage to a non-selected memory cell among the plurality of memory
cells, the third voltage allowing the electric current to flow only
to the semiconductor layer of the non-selected memory cell of the
memory cell array; and applying a programming voltage, which has
dropped due to the compensation memory cell, to the selected memory
cell of the memory cell array.
18. The operating method of claim 17, wherein an absolute value of
the first voltage is greater than an absolute value of the second
voltage.
19. The operating method of claim 17, wherein an absolute value of
the first voltage is less than an absolute value of the third
voltage.
20. The operating method of claim 17, wherein a magnitude of the
first voltage allows a resistance of the corresponding portion of
the semiconductor layer in the compensation memory cell to be less
than a resistance of the corresponding portion of the variable
resistance layer in the compensation memory cell.
21. The operating method of claim 17, wherein a resistance of the
corresponding portion of the semiconductor layer in the
compensation memory cell is less than or equal to 1/10 of the
resistance of corresponding portion of the variable resistance
layer in the compensation memory cell.
22. The operating method of claim 17, wherein a magnitude of the
first voltage allows a resistance of the corresponding portion of
the semiconductor layer in the compensation memory cell to be
within a range of 10.sup.5 .OMEGA.m.sup.-1 to 10.sup.7
.OMEGA.m.sup.-1.
23. The operating method of claim 17, wherein the compensation
memory cell and the selected memory cell are connected to each
other in series.
24. The operating method of claim 17, wherein the corresponding
portion of the semiconductor layer and the corresponding portion of
the variable resistance layer in the compensation memory cell are
connected to each other in parallel.
25. A non-volatile memory device comprising: a substrate; a cell
string on the substrate, the cell string including a plurality of
memory cells stacked on top of each other, a semiconductor layer,
and a resistance change layer, each memory cell, of the plurality
of memory cells in the cell string, including a corresponding
portion of the semiconductor layer connected to a corresponding
portion of a variable resistance layer, the plurality of memory
cells including a non-selected memory cell, a compensation memory
cell, and a selected memory cell; a bit line connected to the cell
string, the bit line configured to apply a programing voltage to
the cell string during a program operation; a plurality of word
lines on the substrate, the plurality of word lines including a
non-selected word line connected to the corresponding portion of
the semiconductor layer of the non-selected memory cell, a
compensation word line connected to the corresponding portion of
the semiconductor layer of the compensation memory cell, and a
selected word line connected to the corresponding portion of the
semiconductor layer of the selected memory cell; and a control
logic, during the program operation, being configured to apply an
adjusted program voltage to the selected memory cell, based on
applying a turn-on voltage to the non-selected word line, applying
a turn-off voltage to the selected word line, and applying a
compensation voltage to the compensation word line, a magnitude of
the adjusted program voltage being less than a magnitude of the
programming voltage, and magnitude of the compensation voltage
being between a magnitude of the turn-on voltage and a magnitude of
the turn-off voltage.
Description
CROSS-REFERENCE TO RELATED APPLICATION
[0001] This application claims the benefit of Korean Patent
Application No. 10-2019-0092660, filed on Jul. 30, 2019, in the
Korean Intellectual Property Office, the disclosure of which is
incorporated herein in its entirety by reference.
BACKGROUND
1. Field
[0002] The present disclosure relates to a non-volatile memory
device and an operating method thereof.
2. Description of Related Art
[0003] Non-volatile memory is a semiconductor memory device and
includes a plurality of memory cells each retaining information
even when supply of electric power thereto is blocked and being
capable of using stored information whenever the electric power is
supplied thereto again. As an example of use of the non-volatile
memory device, the non-volatile memory device may be used in a
mobile phone, a digital camera, a personal digital assistant (PDA),
a mobile computing device, a fixed computing device, and other
devices.
[0004] Recently, research on using of a three-dimensional (or
vertical) NAND (VNAND) in a chip forming a next-generation
neuromorphic computing platform or a neural network has been
conducted.
[0005] In particular, a technique allowing random access to memory
cells with highly integrated and low power characteristics is
demanded.
SUMMARY
[0006] Provided are non-volatile memory devices capable of inducing
resistance states of various levels and operating methods
thereof.
[0007] Provided are non-volatile memory devices that output a
resistance state of a linear scale type and operating methods
thereof.
[0008] Additional aspects will be set forth in part in the
description which follows and, in part, will be apparent from the
description, or may be learned by practice of the presented
embodiments of the disclosure.
[0009] According to an embodiment, a non-volatile memory device
includes a memory cell array having a vertically stacked structure,
a bit line configured to apply a programming voltage to the memory
cell array, and a control logic. The memory cell array may include
a semiconductor layer and a variable resistance layer. The memory
cell array may include a plurality of memory cells that each
include a corresponding portion of the semiconductor layer and a
corresponding portion of the variable resistance layer. The
plurality of memory cells may include a non-selected memory cell, a
compensation memory cell, and a selected memory cell. The control
logic may be configured to apply an adjusted program voltage to the
selected memory cell, the adjusted program voltage being dropped
compared to the programming voltage due to the compensation memory
cell, based on applying a first voltage to the compensation memory
cell, a second voltage to the selected memory cell, and a third
voltage to the non-selected memory cell. The first voltage may
allow an electric current of a certain magnitude to flow to the
compensation memory cell. The second voltage may allow the electric
current to flow only to the corresponding portion of the variable
resistance layer of the selected memory cell. The third voltage may
allow the electric current to flow only to the semiconductor layer
of the non-selected memory cell.
[0010] In some embodiments, an absolute value of the first voltage
may be greater than an absolute value of the second voltage.
[0011] In some embodiments, an absolute value of the first voltage
may be less than an absolute value of the third voltage.
[0012] In some embodiments, a magnitude of the first voltage may
allow a resistance of the corresponding portion of the
semiconductor layer in the compensation memory cell to be less than
a resistance of the corresponding portion of the variable
resistance layer in the compensation memory cell.
[0013] In some embodiments, a resistance of the corresponding
portion of the semiconductor layer in the compensation memory cell
is less than or equal to 1/10 of a resistance of the corresponding
portion of the variable resistance layer in the compensation memory
cell.
[0014] In some embodiments, a magnitude of the first voltage may
allow a resistance of the corresponding portion of the
semiconductor layer in the compensation memory cell to be within a
range of 10.sup.5 .OMEGA.m.sup.-1 to 10.sup.7 .OMEGA.m.sup.-1,
based on the control logic applying the first voltage to the
compensation memory cell.
[0015] In some embodiments, a magnitude of the first voltage allows
a resistance of the corresponding portion of the variable
resistance layer in the compensation memory cell to be within a
range of 10.sup.8 .OMEGA.m.sup.-1 to 10.sup.11 .OMEGA.m.sup.-1,
based on the control logic applying the first voltage to the
compensation memory cell.
[0016] In some embodiments, the compensation memory cell and the
selected memory cell may be connected to each other in series.
[0017] In some embodiments, the control logic may be configured to
make the electric current of the certain magnitude flow to the
corresponding portion of the variable resistance layer in the
selected memory cell during a program operation.
[0018] In some embodiments, the corresponding portion of the
semiconductor layer in the compensation memory cell and the
corresponding portion of the variable resistance layer in the
compensation memory cell may be connected to each other in
parallel.
[0019] In some embodiments, the memory cell array may include the
semiconductor layer extending in a first direction; a plurality of
gates and a plurality of insulators extending in a second direction
perpendicular to the first direction, the plurality of gates and
the plurality of insulators being alternately arranged; a gate
insulating layer extending in the first direction among the
plurality of gates, the plurality of insulators, and the
semiconductor layer; and the variable resistance layer extending in
the first direction on the semiconductor layer.
[0020] In some embodiments, the variable resistance layer may be in
contact with the semiconductor layer.
[0021] In some embodiments, the variable resistance layer may be
spaced apart from the gate insulating layer with the semiconductor
layer therebetween.
[0022] In some embodiments, the variable resistance layer may have
a hysteresis characteristic.
[0023] In some embodiments, the variable resistance layer may
include a transition metal oxide, a transition metal nitride, or
both the transition metal oxide and the transition metal
nitride.
[0024] In some embodiments, the compensation memory cell may be a
first compensation memory cell, the plurality of memory cells may
further include a second compensation memory cell, and the first
compensation memory cell and the second compensation memory cell
each may be connected to the selected memory cell in series.
[0025] According to an embodiment, an operating method of a
non-volatile memory device is provided. The non-volatile memory
device includes a memory cell array having a vertically stacked
structure and including a semiconductor layer and a variable
resistance layer. The memory cell array includes a plurality of
memory cells that each include a corresponding portion of the
semiconductor layer and a corresponding portion of the variable
resistance layer. The method includes: applying a first voltage to
a compensation memory cell among the plurality of memory cells, the
first voltage allowing an electric current of a certain magnitude
to flow to the corresponding portion of the compensation memory
cell in the memory cell array; applying a second voltage to a
selected memory cell among the plurality of memory cells, the
second voltage allowing the electric current to flow only to the
corresponding portion of the variable resistance layer in the
selected memory cell of the memory cell array; applying a third
voltage to a non-selected memory cell among the plurality of memory
cells, the third voltage allowing the electric current to flow only
to the semiconductor layer of the non-selected memory cell of the
memory cell array; and applying a programming voltage, which has
dropped due to the compensation memory cell, to the selected memory
cell of the memory cell array.
[0026] In some embodiments, an absolute value of the first voltage
may be greater than an absolute value of the second voltage.
[0027] In some embodiments, an absolute value of the first voltage
may be less than an absolute value of the third voltage.
[0028] In some embodiments, a magnitude of the first voltage may
allow a resistance of the corresponding portion of the
semiconductor layer in the compensation memory cell to be less than
a resistance of the corresponding portion of the variable
resistance layer in the compensation memory cell.
[0029] In some embodiments, a resistance of the corresponding
portion of the semiconductor layer in the compensation memory cell
may be less than or equal to 1/10 of the resistance of
corresponding portion of the variable resistance layer in the
compensation memory cell.
[0030] In some embodiments, a magnitude of the first voltage may
allow a resistance of the corresponding portion of the
semiconductor layer in the compensation memory cell to be within a
range of 10.sup.5 .OMEGA.m.sup.-1 to 10.sup.7 .OMEGA.m.sup.-1.
[0031] In some embodiments, the compensation memory cell and the
selected memory cell may be connected to each other in series.
[0032] In some embodiments, the corresponding portion of the
semiconductor layer and the corresponding portion of the variable
resistance layer in the compensation memory cell may be connected
to each other in parallel
[0033] According to an embodiment, a non-volatile memory device
includes a substrate, a cell string on the substrate, a bit line
connected to the cell string, a plurality of word lines on the
substrate, and a control logic. The cell string may include a
plurality of memory cells stacked on top of each other, a
semiconductor layer, and a resistance change layer. Each memory
cell, of the plurality of memory cells in the cell string, may
include a corresponding portion of the semiconductor layer
connected to a corresponding portion of a variable resistance
layer. The plurality of memory cells may include a non-selected
memory cell, a compensation memory cell, and a selected memory
cell. The bit line may be configured to apply a programing voltage
to the cell string during a program operation. The plurality of
word lines may include a non-selected word line connected to the
corresponding portion of the semiconductor layer of the
non-selected memory cell, a compensation word line connected to the
corresponding portion of the semiconductor layer of the
compensation memory cell, and a selected word line connected to the
corresponding portion of the semiconductor layer of the selected
memory cell. The control logic, during the program operation, may
be configured to apply an adjusted program voltage to the selected
memory cell, based on applying a turn-on voltage to the unselected
word line, applying a turn-off voltage to the selected word line,
and applying a compensation voltage to the compensation word line.
A magnitude of the adjusted program voltage may be less than a
magnitude of the programming voltage. A magnitude of the
compensation voltage may be between a magnitude of the turn-on
voltage and a magnitude of the turn-off voltage.
[0034] In some embodiments, an absolute value of the compensation
voltage may be greater than an absolute value of the turn-off
voltage, and an absolute value of the compensation voltage may be
less than an absolute value of the turn-on voltage.
[0035] In some embodiments, the compensation memory cell and the
selected memory cell may be connected to each other in series, and
the variable resistance layer may directly contact the
semiconductor layer.
[0036] In some embodiments, the selected memory cell may be between
the non-selected memory cell and the compensation memory cell.
[0037] In some embodiments, the variable resistance layer may
include a transition metal oxide, a transition metal nitride, or
both the transition metal oxide and the transition metal
nitride.
BRIEF DESCRIPTION OF THE DRAWINGS
[0038] The above and other aspects, features, and advantages of
certain embodiments of the disclosure will be more apparent from
the following description taken in conjunction with the
accompanying drawings, in which:
[0039] FIG. 1 is a block diagram of a memory system according to an
embodiment;
[0040] FIG. 2 is a block diagram of an implemented example of the
memory device of FIG. 1;
[0041] FIG. 3 is a block diagram of a memory cell array of FIG.
1;
[0042] FIG. 4 is a diagram of an equivalent circuit corresponding
to a memory block according to an embodiment;
[0043] FIG. 5 is a diagram showing a physical structure
corresponding to a memory block according to an embodiment;
[0044] FIG. 6A is an XZ cross-sectional view of the memory block of
FIG. 5;
[0045] FIG. 6B is a YZ cross-sectional view of the memory block of
FIG. 5;
[0046] FIG. 7 is a diagram of the equivalent circuit of the memory
block of FIG. 4 operating in a programming mode of the non-volatile
memory device, according to the embodiment;
[0047] FIG. 8 is a diagram illustrating relationship between
resistances of a compensation memory cell and a selected memory
cell in a programming mode according to an embodiment;
[0048] FIGS. 9A and 9B are diagrams regarding a current movement in
a resistance variable layer in a programming mode according to an
embodiment;
[0049] FIG. 10 is a reference diagram illustrating the concept of a
resistance variable layer capable of ensuring a multi-resistance
state according to an embodiment;
[0050] FIG. 11 is a diagram of an equivalent circuit in a
programming mode of a memory block according to another
embodiment;
[0051] FIG. 12 is a diagram of a memory block including a
compensation memory cell having no resistance variable layer
according to another embodiment;
[0052] FIG. 13 is a diagram of an equivalent circuit of a memory
block in a reading mode according to an embodiment;
[0053] FIG. 14 is a diagram regarding a current movement in a
selected memory cell in the reading mode of FIG. 13;
[0054] FIG. 15 is a diagram showing a circuit during a reading mode
of a memory block according to another embodiment; and
[0055] FIG. 16 is a diagram regarding a current movement in a
selected memory cell during the reading mode of FIG. 15.
DETAILED DESCRIPTION
[0056] Reference will now be made in detail to embodiments,
examples of which are illustrated in the accompanying drawings,
wherein like reference numerals refer to like elements throughout.
In this regard, the present embodiments may have different forms
and should not be construed as being limited to the descriptions
set forth herein. Accordingly, the embodiments are merely described
below, by referring to the figures, to explain aspects. As used
herein, the term "and/or" includes any and all combinations of one
or more of the associated listed items. Expressions such as "at
least one of," when preceding a list of elements, modify the entire
list of elements and do not modify the individual elements of the
list.
[0057] Phrases such as "in some embodiments of the disclosure" or
"in one embodiment of the disclosure" throughout the specification
may not necessarily denote the same embodiment of the
disclosure.
[0058] Some embodiments of the disclosure may be represented as
functional block structures, various processing stages and/or
various processing operations. Some or all of the functional blocks
may be realized by any number of hardware and/or software
components configured to perform the specified functions. For
example, the functional blocks of the disclosure may be realized by
one or more microprocessors or circuit structures for performing a
desired and/or alternatively predetermined function. In addition,
for example, the functional blocks of the disclosure may be
implemented with any programming or scripting language. The
functional blocks may be implemented in algorithms that are
executed on one or more processors. Also, the disclosure may employ
any number of conventional techniques for electronics
configuration, signal processing and/or, data processing and the
like. The words "mechanism," "element," "means," and
"configuration" are used broadly and are not limited to mechanical
or physical components.
[0059] In addition, the connecting lines, or connectors shown in
the various figures presented are intended to represent example
functional relationships and/or physical or circuit couplings
between the various elements. It should be noted that connections
between elements by many alternative or additional functional
relationships, physical connections or circuit connections may be
present in a practical device.
[0060] The terms "consist(s) of" or "include(s) (or comprise(s))"
should not be interpreted or understood as including, without
exception, all of the plurality of elements or the plurality of
steps disclosed in the description. In other words, it should be
understood that some of the elements or some of the steps may not
be included, or that additional elements or steps may be further
included.
[0061] When a layer, a film, a region, or a panel is referred to as
being "on" another element, it may be directly on/under/at
left/right sides of the other layer or substrate, or intervening
layers may also be present. Hereinafter, one or more embodiments of
the disclosure will be described in detail with reference to
accompanying drawings.
[0062] It will be understood that although the terms "first" and
"second" are used herein to describe various elements, these
elements should not be limited by these terms. Terms are only used
to distinguish one element from other elements.
[0063] The disclosure will be described in detail below with
reference to accompanying drawings.
[0064] FIG. 1 is a block diagram of a memory system 10 according to
an embodiment.
[0065] Referring to FIG. 1, the memory system 10 may include a
memory controller 100 and a memory device 200. The memory
controller 100 performs a control operation with respect to the
memory device 200, for example, the memory controller 100 provides
the memory device 200 with an address ADD and a command CMD to
perform a programming (or writing), a reading, and an erasing
operation with respect to the memory device 200. Also, data for the
programming operation and read data may be transmitted between the
memory controller 100 and the memory device 200.
[0066] The memory device 200 may include a memory cell array 210
and a voltage generator 220. The memory cell array 210 may include
a plurality of memory cells that are arranged on regions where a
plurality of word lines and a plurality of bit lines intersect with
each other. The memory cell array 210 includes non-volatile memory
cells that store data in a non-volatile way, and as the
non-volatile memory cells, the memory cell array 210 may include
flash memory cells such as NAND flash memory cells or NOR flash
memory cells. Hereinafter, one or more embodiments of the
disclosure will be described under an assumption that the memory
cell array 210 includes the flash memory cell array 210 and the
memory device 200 is a non-volatile memory device.
[0067] The memory controller 100 may include a record/read
controller 110, a voltage controller 120, and a data determiner
130. In example embodiments, the memory controller 100 may include
processing circuitry such as hardware including logic circuits; a
hardware/software combination such as a processor executing
software; or a combination thereof. For example, the processing
circuitry more specifically may include, but is not limited to, a
central processing unit (CPU), an arithmetic logic unit (ALU), a
digital signal processor, a microcomputer, a field programmable
gate array (FPGA), a System-on-Chip (SoC), a programmable logic
unit, a microprocessor, application-specific integrated circuit
(ASIC), etc. The memory controller 100 may operate in response to
requests from a host (not shown) and may be configured to access
the memory device 200 and control operations discussed below,
thereby transforming the memory controller 100 into a special
purpose controller. As discussed below, the memory controller 100
may improve the functioning of the memory device 200 by reducing
and/or suppressing a dielectric breakdown and current overshoot of
a selected memory cell and/or by allowing multi-resistance states
to be recorded in memory cells of the memory device 200.
[0068] The record/read controller 110 may generate an address ADD
and a command CMD for performing programming/reading/erasing
operations on the memory cell array 210. Also, the voltage
controller 120 may generate a voltage control signal for
controlling at least one voltage level used in the non-volatile
memory device 200. As an example, the voltage controller 120 may
generate the voltage control signal for controlling a voltage level
of a word line for reading data from or programming data on the
memory cell array 210.
[0069] In addition, the data determiner 130 may perform a
determination operation on the data read from the non-volatile
memory device 200. For example, by determining the data read from
the memory cells, the number of on-cells and/or off-cells from
among the memory cells may be determined. As an operating example,
when programming is performed on a plurality of memory cells,
states of the data from the memory cells are determined by using a
desired and/or alternatively predetermined reading voltage to
determine whether the programming is normally finished on every
cell. Alternatively or additionally, the memory device 200 may
provide the memory controller 100 a pass/fail signal P/F according
to a read result with respect to the read data. The memory
controller 100 may refer to the pass/fail signal P/F and thus
control write and read operations of the memory cell array 210.
[0070] As described above, the memory cell array 210 may include
non-volatile memory cells, for example, the memory cell array 210
may include flash memory cells. Also, the flash memory cells may be
implemented in various types, for example, the memory cell array
210 may include three-dimensional (or vertical) NAND (VNAND) memory
cells.
[0071] FIG. 2 is a block diagram of an implemented example of the
memory device 200 of FIG. 1.
[0072] As shown in FIG. 2, the memory device 200 may further
include a row decoder 230, an input/output circuit 240, and a
control logic 250.
[0073] The memory cell array 210 may be connected to one or more
string selection lines SSL, a plurality of word lines WL (WL1 to
WLm, including normal word lines WL and dummy word lines WL), and
one or more common source lines CSL, and may be also connected to a
plurality of bit lines BL1 to BLn. The voltage generator 220 may
generate one or more word line voltages V1 to Vi that may be
provided to the row decoder 230. Signals for
programming/reading/erasing operations may be applied to the memory
cell array 210 via the bit lines BL1 to BLn.
[0074] Also, data to be programmed may be provided to the memory
cell array 210 via the input/output circuit 240 and the read data
may be provided to outside (e.g., memory controller) via the
input/output circuit 240. In example embodiments, the control logic
250 may include processing circuitry such as hardware including
logic circuits; a hardware/software combination such as a processor
executing software; or a combination thereof. For example, the
processing circuitry more specifically may include, but is not
limited to, a central processing unit (CPU), an arithmetic logic
unit (ALU), a digital signal processor, a microcomputer, a field
programmable gate array (FPGA), a System-on-Chip (SoC), a
programmable logic unit, a microprocessor, application-specific
integrated circuit (ASIC), etc. The control logic 250 may operate
in response to requests from memory controller 100 (see FIG. 1) and
the control logic 250 may provide the row decoder 230 and the
voltage generator 220 with various control signals related to the
memory operations under the control of the memory controller 100.
As discussed below, the control logic 250 may improve the
functioning of the memory device 200 by reducing and/or suppressing
a dielectric breakdown and current overshoot of a selected memory
cell and/or by allowing multi-resistance states to be recorded in
memory cells of the memory device 200.
[0075] According to a decoding operation of the row decoder 230,
the word line voltages V1 to Vi may be provided to various lines
SSL, WL1 to WLm, and CSL. For example, the word line voltages V1 to
Vi may include a string selection voltage, word line voltages, and
ground selection voltages. The string selection voltage may be
provided to one or more string selection lines SSL, the word line
voltages may be provided to one or more word lines WL1 to WLm, and
the ground selection voltages may be provided to one or more common
source lines CSL.
[0076] FIG. 3 is a block diagram of the memory cell array 210 of
FIG. 1.
[0077] Referring to FIG. 3, the memory cell array 210 includes a
plurality of memory blocks BLK (BLK1 to BLKz). Each of the memory
blocks BLK (BLK1 to BLKz) has a three-dimensional (or vertical)
structure. For example, each memory block BLK includes structures
extending in first to third directions. For example, as discussed
with reference to FIG. 4, each memory block BLK includes a
plurality of cell strings CS extending in the second direction. For
example, the plurality of cell strings CS are provided in the first
to third directions.
[0078] Each of the cell strings CS is connected to the bit line BL,
the string selection line SSL, the word lines WL, and the common
source line CSL. That is, each of the memory blocks BLK (BLK1 to
BLKz) is connected to the plurality of bit lines BL, the plurality
of string selection lines SSL, the plurality of word lines WL, and
the plurality of common source lines CSL. The memory blocks BLK
(BLK1 to BLKz) will be described in more detail below with
reference to FIG. 4.
[0079] FIG. 4 is a diagram of an equivalent circuit corresponding
to a memory block BLKi according to an embodiment. As an example,
one of the memory blocks BLK (BLK1 to BLKz) in the memory cell
array 210 of FIG. 3 is shown in FIG. 4.
[0080] Referring to FIG. 3 and FIG. 4, the memory block BLKi
includes a plurality of cell strings CS (for example, CS11 to
CSkn). The plurality of cell strings CSs are arranged in rows and
columns.
[0081] Each of the cell strings CS11 to CSkn includes memory cells
MC and a string selection transistor SST. The memory cells MC in
each of the cell strings CS11 to CSkn and the string selection
transistors SST may be stacked in a height direction.
[0082] The plurality of rows of the cell strings CS11 to CSkn are
respectively connected to different string selection lines SSL1 to
SSLk. For example, the string selection transistors SST in the cell
strings CS11 to CS1n are commonly connected to the string selection
line SSL1. The string selection transistors SST in the cell strings
CSk1 to CSkn are commonly connected to the string selection line
SSLk.
[0083] The plurality of columns of the cell strings CS are
respectively connected to different bit lines BL1 to BLn. For
example, the memory cells MC and the string selection transistors
SST in the cell strings CS11 to CSk1 may be commonly connected to
the bit line BL1, and the memory cells MC and the string selection
transistors SST in the cell strings CS1n to CSkn may be commonly
connected to the bit line BLn.
[0084] The plurality of rows of the cell strings CS may be
respectively connected to different common source lines CSL1 to
CSLk. For example, the string selection transistors SST in the cell
strings CS11 to CS1n may be commonly connected to the common source
line CSL1 and the string selection transistors SST of the cell
strings CSk1 to CSkn may be commonly connected to the common source
line CSLk.
[0085] The memory cells at the same height from the substrate (or
the string selection transistors SST) are commonly connected to a
same word line WL and the memory cells at different heights from
the substrate (or the string selection transistors SST) may be
respectively connected to different word lines WL1 to WLm.
[0086] The memory block BLKi shown in FIG. 4 is an example. One or
more embodiments are not limited to the memory block BLKi shown in
FIG. 4. For example, the number of rows of the cell strings CS may
be increased or decreased. As the number of rows of the cell
strings CS varies, the number of string selection lines connected
to the rows of the cell strings CS and the number of cell strings
CS connected to one bit line may also vary. As the number of rows
of the cell strings CS varies, the number of common source lines
connected to the rows of the cell strings CS may also vary.
[0087] The number of columns of the cell strings CS may be also
increased or decreased. As the number of columns of the cell
strings CS varies, the number of bit lines connected to the columns
of the cell strings CS and the number of cell strings CS connected
to one string selection line may also vary.
[0088] The height of the cell strings CS may increase or decrease.
For example, the number of memory cells stacked in each of the cell
strings CS may increase or decrease. As the number of memory cells
stacked in each of the cell strings CS varies, the number of word
lines WL may also vary. For example, the number of string selection
transistors provided to each of the cell strings CS may increase.
As the number of string selection transistors provided to each of
the cell strings CS varies, the number of the string selection
lines or the common source lines may also vary. As the number of
string selection transistors increases, the string selection
transistors may be stacked like the memory cells MC.
[0089] For example, writing and reading operations may be performed
in units of rows of the cell strings CS. The cell strings CS are
selected by the common source lines CSL in units of one row and may
be selected by the string selection lines SSL in units of one row.
Also, the voltage may be applied in units of at least two common
source lines CSL. The voltage may be applied in units of total
common source lines CSL.
[0090] In a selected row of the cell strings CS, the programming
and reading operations may be performed in units of pages. A page
may denote one row of memory cells MCconnected to one word line WL.
In the selected row of the cell strings CS, the memory cells MC may
be selected by the word lines WL in units of pages.
[0091] In addition, each of the memory cells MC may correspond to a
circuit in which a transistor and a resistor are connected in
parallel.
[0092] FIG. 5 is a diagram showing a physical structure
corresponding to a memory block according to an embodiment, FIG. 6A
is a cross-sectional diagram of the memory block of FIG. 5 in an XZ
plane, and FIG. 6B is a cross-sectional view of the memory block of
FIG. 5 in a YZ plane.
[0093] Referring to the drawings, a substrate 501 is prepared. The
substrate 501 may include a silicon material doped with first type
impurities. For example, the substrate 501 may include a silicon
material doped with p-type impurities. For example, the substrate
501 may include a p-type well (e.g., a pocket p-well). Hereinafter,
it will be assumed that the substrate 501 includes p-type silicon.
However, the substrate 501 is not limited to the p-type
silicon.
[0094] A doping region 510 is provided in the substrate 501. For
example, the doping region 510 may be of a second type that is
different from that of the substrate 501. For example, the doping
region 510 may be of an n-type. Hereinafter, it will be assumed
that the doping region 510 is of the n-type. However, the doping
region 510 is not limited to the n-type. The doping region 510 may
be a common source line.
[0095] On the substrate 501, a plurality of gates 531 and a
plurality of insulators 532 extending in a horizontal direction may
be alternately arranged. That is, the plurality of gates 531 and
the plurality of insulators 532 may be stacked alternately with
each other along a vertical direction that is perpendicular to the
horizontal direction. For example, the gate 531 may include a metal
material (e.g., copper, silver, etc.) and the insulator 532 may
include silicon oxide, but are not limited thereto. Each of the
gates 531 is connected to one of the word line WL and the string
selection line SSL.
[0096] A pillar 520 is provided to penetrate through the plurality
of gates 531 and the plurality of insulators 532 in the vertical
direction, wherein the plurality of gates 531 and the plurality of
insulators 532 are alternately arranged.
[0097] The pillar 520 may include a plurality of layers. In an
embodiment, an outermost layer of the pillar 520 may be a gate
insulating layer 521. For example, the gate insulating layer 521
may include silicon oxide. The gate insulating layer 521 may be
conformally stacked on the pillar 520.
[0098] Also, a semiconductor layer 522 may be conformally deposited
on an internal surface of the gate insulating layer 521. In an
embodiment, the semiconductor layer 522 may include a silicon
material doped with the first type impurities. The semiconductor
layer 522 may include a silicon material doped with the same type
as that of the substrate 501. For example, when the substrate 501
includes the silicon material doped with p-type impurities, the
semiconductor layer 522 may also include the silicon material doped
with the p-type impurities. Alternatively, the semiconductor layer
522 may include a material such as Ge, IGZO, GaAs, etc.
[0099] A variable resistance layer 523 may be arranged along the
internal surface of the semiconductor layer 522. The variable
resistance layer 523 may be arranged to be in contact with the
semiconductor layer 522 and may be conformally stacked on the
semiconductor layer 522. In an embodiment, the variable resistance
layer 523 may include a material having a resistance that varies
depending on an applied voltage. The variable resistance layer 523
may switch from a high resistance state to a low resistance state
or from the low resistance state to the high resistance state,
according to the voltage applied to the gate 531. The above
variable resistance may be caused by oxygen vacancies in the
variable resistance layer 523 or may be caused by the change in a
current conducting mechanism due to trapping/de-trapping of
electrons in the variable resistance layer 523.
[0100] The variable resistance layer 523 may include a material
having a hysteresis characteristic. For example, the variable
resistance layer 523 may include a transition metal oxide and/or a
transition metal nitride. In detail, the variable resistance layer
523 may include oxide of at least one element selected from the
group consisting of zirconium (Zr), hafnium (Hf), aluminum (Al),
nickel (Ni), copper (Cu), molybdenum (Mo), tantalum (Ta), titanium
(Ti), tungsten (W), chromium (Cr), strontium (Sr), lanthanum (La),
manganese (Mn), calcium (Ca), praseodymium (Pr), and silicon (Si).
Also, the variable resistance layer 523 may include silicon nitride
and aluminum nitride.
[0101] An insulating layer 524 may be filled in the variable
resistance layer 523. For example, the insulating layer 524 may
include silicon oxide.
[0102] The semiconductor layer 522 and the variable resistance
layer 523 may be in contact with the doping region 510, e.g., a
common source region.
[0103] A drain 540 may be provided on the pillar 520. The drain 540
may include a silicon material doped with the second type
impurities. For example, the drain 540 may include the silicon
material doped with n-type impurities.
[0104] A bit line 550 may be provided on the drain 540. The drain
540 and the bit line 550 may be connected to each other via contact
plugs. The bit line 550 may include a metal material and/or a
semiconductor material, e.g., polysilicon. The bit line may be
formed of conductive material.
[0105] As compared with FIG. 4, the plurality of gates 531, the
plurality of insulators 532, the gate insulating layer 521, the
semiconductor layer 522, and the variable resistance layer 523 are
elements of the cell strings CS. In detail, the gates 531, the gate
insulating layer 521, and the semiconductor layer 522 are elements
of the transistor and the variable resistance layer 523 may be a
resistor.
[0106] As shown in the drawings, since the semiconductor layer 522
of the transistor and the variable resistance layer 523 is directly
bonded to each other, the variable resistance layer 523 may have
the high resistance state or the low resistance state to record
data on the memory cells MC. The semiconductor layer 522 of the
transistor and the variable resistance layer 523 is connected in
parallel in each of the memory cells MC, and the parallel
connecting structures are successively arranged in the vertical
direction to form the cell string CS. In addition, the common
source line 510 and the bit line 550 may be connected to opposite
ends of the cell string CS. When the voltage is applied to the
common source line 510 and the bit line 550, the programming,
reading, and erasing operations may be performed on the plurality
of memory cells MC.
[0107] According to inventive concepts, the memory block includes
the variable resistance layer 523 instead of using a phase change
material, and thus issues such as heat generation and stress
(pressure) caused when the phase change material is used may be
addressed. Also, since the memory block is configured as described
above and operated, even when the memory cells included in the
memory block are repeatedly operated, ion transfer between adjacent
memory cells and leakage current and operation failure due to the
ion transfer may be prevented. Density in the memory block may
increase because a scaling issue between the memory cells in a
next-generation VNAND may be addressed.
[0108] The memory block according to the embodiment of the
disclosure may be implemented as a chip to be used as a
neuromorphic computing platform. In addition, the memory block
according to the embodiment of the disclosure may be implemented as
a chip to be used to configure a neural network.
[0109] The memory controller 100 may control the memory device 200
to operate in a programming mode.
[0110] FIG. 7 is a diagram of the equivalent circuit of a memory
block 700 according to FIG. 4, in a programming mode of the
non-volatile memory device according to the embodiment.
[0111] In FIG. 7, each of a plurality of memory cells 710, 720, and
730 may include the gate 531, gate insulating layer 521, the
variable resistance layer 523, and the semiconductor layer 522 of
FIG. 5.
[0112] The plurality of memory cells 710, 720, and 730 in the
memory block 700 may include a compensation memory cell 710, a
selected memory cell 720, and a non-selected memory cell 730. A
programming mode of a non-volatile memory device denotes a mode in
which a programming operation is performed on the memory cell MC
and the memory cell MC that is a target of the programming
operation may be referred to as the selected memory cell 720. In
addition, the memory cell MC that is not a target of the
programming operation may be referred to as the non-selected memory
cell 730.
[0113] The compensation memory cell 710 may denote the memory cell
MC that adjusts a magnitude of electric current flowing on the
selected memory cell 720. Since the compensation memory cell 710
and the selected memory cell 720 are connected in series, when the
magnitude of the electric current flowing in the compensation
memory cell 710 is controlled, a magnitude of electric current
flowing in the selected memory cell 720 may be controlled.
[0114] The control logic 250 may control a turn-on voltage Von to
be applied to a string selection line SSL connected to the selected
memory cell 720, from among the plurality of string selection lines
SSL. In addition, from among the plurality of word lines WL, the
control logic 250 applies a compensation voltage Vcc to a word line
WL connected to the compensation memory cell 710, applies a
turn-off voltage Voff to a word line WL connected to the selected
memory cell 720, and applies the turn-on voltage Von to the word
line WL connected to the non-selected memory cell 730.
[0115] The compensation voltage Vcc may be a voltage allowing an
electric current of a certain magnitude to flow on the compensation
memory cell 710, for example, the electric current of a certain
magnitude may flow on the semiconductor layer 522 of the
compensation memory cell 710. The turn-off voltage Voff is a
voltage of a magnitude for turning off the transistor and may
prevent the electric current from flowing on the semiconductor
layer 522 of the transistor included in the selected memory cell
720. The turn-on voltage Von is a voltage of a magnitude for
turning on the transistor and may allow the electric current to
flow only on the semiconductor layer 522 of the transistor included
in the non-selected memory cell 730.
[0116] Magnitudes of the compensation voltage Vcc, the turn-off
voltage Voff, and the turn-on voltage Von may vary depending on
kinds, thickness, etc. of the materials included in the gate 531,
the gate insulating layer 521, the semiconductor layer 522, and the
variable resistance layer 523 in the memory cell MC. An absolute
value of the turn-on voltage Von is generally greater than an
absolute value of the turn-off voltage Voff.
[0117] An absolute value of the compensation voltage Vcc may be
less than that of the turn-on voltage Von and greater than that of
the turn-off voltage Voff. Also, the compensation voltage Vcc may
have a magnitude making the resistance of the semiconductor layer
522 less than that of variable resistance layers 523 and 523c in
the compensation memory cell 710. For example, the compensation
voltage Vcc may have a magnitude that makes the resistance of the
semiconductor layer 522 in the compensation memory cell 710 equal
to or less than 1/10 of the resistance of the variable resistance
layer 523 in the compensation memory cell 710.
[0118] Alternatively, the compensation voltage Vcc may be in a
range in which the resistance of the semiconductor layer 522 in the
compensation memory cell 710 is 10.sup.5 .OMEGA.m.sup.-1 to
10.sup.7 .OMEGA.m.sup.-1 or the resistance of the variable
resistance layer 523 of the compensation memory cell 710 is
10.sup.8 .OMEGA.m.sup.-1 to 10.sup.11 .OMEGA.m.sup.-1.
[0119] Since the semiconductor layer 522 and the variable
resistance layer 523 in the compensation memory cell 710 are
connected in parallel and the resistance of the semiconductor layer
522 is relatively less than the resistance of the variable
resistance layer 523, the magnitude of the electric current flowing
on the compensation memory cell 710 may be determined according to
the magnitude of the electric current flowing on the semiconductor
layer 522 of the compensation memory cell 710. Thus, the magnitude
of the electric current flowing on the compensation memory cell 710
may be determined according to the magnitude of the compensation
voltage Vcc applied to the transistor of the compensation memory
cell 710.
[0120] In addition, a programming voltage Vprogram may be applied
to a bit line BL connected to the selected memory cell 720, from
among the plurality of bit lines BL. The programming voltage
Vprogram may be provided from outside, e.g., the memory controller
100, via the input/output circuit 240. The programming voltage
Vprogram is a voltage for recording data on the memory cell MC and
the magnitude of the programming voltage Vprogram may vary
depending on the data.
[0121] The bit line BL that is not connected to the selected memory
cell 720, from among the plurality of bit lines BL, may be grounded
or floated. Since the bit line that is not connected to the
selected memory cell 720 is grounded or floated, electric power
loss because of the leakage current may be prevented. Thus, the
control logic 250 may perform the programming operation on the
selected memory cell 720.
[0122] In the programming mode, because the turn-off voltage Voff
is applied to the selected memory cell 720, the semiconductor layer
522 of the selected memory cell 720 may have an insulating
property. In addition, since the turn-on voltage Von is applied to
the non-selected memory cell 730, the semiconductor layer 522 of
the non-selected memory cell 730 may have a conductive property.
Therefore, there is a voltage difference between the compensation
memory cell 710 and the selected memory cell 720 due to the
programming voltage Vprogram.
[0123] Since the electric current of a certain magnitude flows on
the compensation memory cell 710, a programming voltage Vprogram'
that is voltage-dropped by the compensation memory cell 710 is
applied to the selected memory cell 720. The programming voltage
Vprogram' (also referred to as adjusted program voltage) is less
than the programming voltage Vprogram. Thus, dielectric breakdown
and current overshoot of the selected memory cell 720 due to the
programming voltage Vprogram may be limited and/or suppressed.
[0124] FIG. 8 is a diagram illustrating relationship between
resistances of the compensation memory cell 710 and the selected
memory cell 720 in a programming mode according to an
embodiment.
[0125] As shown in FIG. 8, the memory block may include the gates
531, the insulators 532, the gate insulating layer 521, the
semiconductor layer 522, the variable resistance layer 523, and the
insulating layer 524 on a substrate (not shown). The gate
insulating layer 521, the semiconductor layer 522, the variable
resistance layer 523, and the insulating layer 524 may extend in a
first direction. The gates 531 and the insulating layer 524 may
alternately extend in a second direction that is perpendicular to
the first direction.
[0126] In addition, the gates 531, the insulators 532, the gate
insulating layer 521, and the semiconductor layer 522 are elements
of the transistor, and the variable resistance layer 523 may
correspond to a resistor.
[0127] In the programming mode, the control logic 250 may control
the compensation voltage Vcc to be applied to a gate 531a of the
compensation memory cell 710, the turn-off voltage Voff to be
applied to a gate 531b of the selected memory cell 720, and the
turn-on voltage Von to be applied to a gate 531c of the
non-selected memory cell 730. Thus, a semiconductor layer 522b of
the selected memory cell 720 has an insulating property and a
semiconductor layer 522c of the non-selected memory cell 730 may
have a conductive property. When the programming voltage Vprogram
is applied to a bit line that is electrically connected to the
selected memory cell 720, there is a voltage difference between the
compensation memory cell 710 and the selected memory cell 720.
[0128] In addition, since the electric current of a certain
magnitude flows on the compensation memory cell 710 due to the
compensation voltage Vcc and the compensation memory cell 710 and
the selected memory cell 720 are connected in series, the above
voltage difference may be distributed to the compensation memory
cell 710 and the selected memory cell 720. That is, the magnitude
of the electric current flowing on the selected memory cell 720 is
controlled by the compensation voltage Vcc applied to the
compensation memory cell 710, and the programming voltage Vprogram'
that is dropped due to the compensation memory cell 710 may be
applied to the selected memory cell 720.
[0129] Since the magnitude of the electric current flowing on the
selected memory cell 720 is controlled by the compensation memory
cell 710, and the dielectric breakdown and electric current
overshoot of the selected memory cell 720 in the programming mode
may be limited and/or suppressed. Also, since the magnitude of the
electric current flowing on the selected memory cell 720 may be
adjusted by adjusting the magnitude of the compensation voltage Vcc
applied to the compensation memory cell 710, the selected memory
cell 720 may have resistance states of various levels. Thus, more
information may be recorded on the memory cell MC.
[0130] Since oxygen vacancies in the variable resistance layer 523b
of the selected memory cell 720 move towards the semiconductor
layer 522b due to the voltage difference, the variable resistance
layer 523b may be in the low resistance state. The variable
resistance layer 523b of the selected memory cell 720 in the low
resistance state may denote that the value of resistor included in
the selected memory cell 720 is decreased.
[0131] FIGS. 9A and 9B are diagrams regarding a current movement in
the variable resistance layer 523 in a programming mode according
to an embodiment.
[0132] The dropped programming voltage Vprogram' may allow the
oxygen vacancies in the variable resistance layer 523b
corresponding to the selected memory cell 720 to move towards the
semiconductor layer 522b. As shown in FIG. 9A, when a density of
oxygen vacancies is high at a region in the variable resistance
layer 523b, which is adjacent to the semiconductor layer 522b, a
conductive filament is generated. Thus, the variable resistance
layer 523b is in the low resistance state and the selected memory
cell 720 may have an ohmic conductive property.
[0133] Alternatively, as shown in FIG. 9B, when the density of the
oxygen vacancies at the region of the variable resistance layer
523b, which is adjacent to the semiconductor layer 522b, the
dropped programming voltage Vprogram' may allow electrons to be
filled in traps that are spaced apart from one another with
constant intervals in the variable resistance layer 523b. Thus, the
variable resistance layer 523b is in the low resistance state due
to the change in the current conducting type, and the selected
memory cell 720 may have a bulk conductive property such as
Hopping, SCLC, and Poole-Frenkel.
[0134] FIG. 10 is a reference diagram illustrating concept of the
variable resistance layer 523 capable of ensuring multi-resistance
state according to an embodiment.
[0135] In FIG. 10, (i) denotes a current-voltage characteristic of
the transistor in the compensation memory cell 710. The electric
current flowing on the semiconductor layer 522 of the transistor
may vary depending on the voltage applied to the gate 531 of the
transistor, and this denotes that the current applied to the
selected memory cell 720 may be controlled by controlling the
voltage applied to the gate 531 of the transistor.
[0136] In FIG. 10, (ii) denotes a current-voltage characteristic of
the variable resistance layer 523 included in the selected memory
cell 720. The variable resistance layer 523 of the selected memory
cell 720 may have hysteresis characteristic. The variable
resistance layer 523 of the memory cell MC may have different
resistance characteristics before and after the current flows.
[0137] In FIG. 10, (iii) shows a current-voltage characteristic of
the variable resistance layer 523 according to the applied current.
The electric current applied to the selected memory cell 720 may be
determined by the compensation memory cell 710, that is, a voltage
at the gate 531 of the compensation memory cell 710. In addition,
the variable resistance layer 523 of the selected memory cell 720
may have resistance state of various levels according to the
magnitude of the applied current. That is, the variable resistance
layer 523 may have the resistance characteristic that varies
depending on the magnitude of the applied current. Thus, more
information may be recorded on one memory cell MC.
[0138] FIG. 11 is a diagram of an equivalent circuit in a
programming mode of a memory block according to another embodiment.
When FIG. 7 and FIG. 11 are compared with each other, in FIG. 7,
one cell string includes one compensation memory cell 710, whereas
in FIG. 11, one cell string includes a plurality of compensation
memory cells 710, e.g., first and second compensation memory cells
710a and 710b. FIG. 11 shows two compensation memory cells 710a and
710b, but one or more embodiments are not limited thereto. That is,
three or more compensation memory cells may be provided.
[0139] The plurality of first and second compensation memory cells,
for example first and second compensation memory cells 710a and
710b are connected to the selected memory cell 720 in series, and a
magnitude of the electric current flowing on each of the first and
second compensation memory cells 710a and 710b may be determined
according to the compensation voltage Vcc applied to each of the
first and second compensation memory cells 710a and 710b. A
magnitude of the electric current applied to the selected memory
cell 720 may be determined according to a minimum magnitude of the
electric current flowing on the plurality of compensation memory
cells, for example, the first and second compensation memory cells
710a and 710b. The dropped programming voltage Vprogram' applied to
the selected memory cell 720 connected to the plurality of
compensation memory cells 710a and 710b is much lower than that
applied to the selected memory cell 720 connected to one
compensation memory cell.
[0140] The compensation memory cell 710 may include a transistor
and a variable resistance layer like in the selected memory cell
720 and the non-selected memory cell 730. However, one or more
embodiments are not limited thereto. In some embodiments, the
variable resistance layer may be omitted and/or not included in the
compensation memory cell 710.
[0141] FIG. 12 is a diagram of a memory block including the
compensation memory cell 710 that does not include the variable
resistance layer 523, according to another embodiment. As shown in
FIG. 12, a compensation memory cell 710c is at an uppermost portion
of the memory block. The compensation memory cell 710c may include
the gates 531, the gate insulating layer 521, and the semiconductor
layer 522. The semiconductor layer 522 of the compensation memory
cell 710a is directly connected to a drain electrode 540 and may be
connected to a source electrode 510 via the semiconductor layers
522 of a plurality of memory cells MC. In FIG. 12, the compensation
memory cell 710c is at the uppermost portion of the memory block,
but one or more embodiments are not limited thereto. The
compensation memory cell 710c may be arranged at a lowermost
portion of the memory block or at a middle portion of the memory
cells MC.
[0142] FIG. 13 is a diagram of an equivalent circuit of a memory
block 800 in a reading mode according to an embodiment. Referring
to FIG. 13, each of the plurality of memory cells MC in the memory
block 800 may include the gates 531, the gate insulating layer 521,
the semiconductor layer 522, and the variable resistance layer 523
shown in FIG. 5.
[0143] The memory cells MC in the memory block 800 may include the
compensation memory cell 710, the selected memory cell 720, and the
non-selected memory cell 730. A reading mode of the non-volatile
memory device denotes a mode in which a reading operation is
performed on the memory block and the selected memory cell 720 may
denote the memory cell MC that is a target on which the reading
operation is performed.
[0144] During the reading mode, the control logic 250 may apply the
turn-on voltage Von to the string selection line SSL connected to
the selected memory cell 720 and may apply the turn-off voltage
Voff to the string selection line that is not connected to the
selected memory cell 720, from among the plurality of string
selection lines SSL. In addition, the control logic 250 may apply
the turn-on voltage Von to the word line WL, from among the
plurality of word lines WL, connected to the compensation memory
cell 710 and the non-selected memory cell 730 and may apply the
turn-off voltage Voff to the word line WL connected to the selected
memory cell 720.
[0145] Here, the turn-on voltage Von has a magnitude that may turn
on the transistor, e.g., allow the electric current to flow only on
the semiconductor layer 522 of the transistor. The turn-off voltage
Voff has a magnitude that may turn off the transistor, e.g., block
the electric current from flowing on the semiconductor layer 522 of
the transistor. Values of the turn-on voltage Von and the turn-off
voltage Voff may vary depending on the kinds, thickness, etc. of
the materials included in the gates 531, the gate insulating layer
521, the semiconductor layer 522, and the variable resistance layer
523 in the plurality of memory cells MC. An absolute value of the
turn-on voltage Von is generally greater than an absolute value of
the turn-off voltage Voff.
[0146] In addition, from among the plurality of bit lines BL, a
reading voltage Vread is applied to the bit line BL connected to
the selected memory cell 720 to perform the reading operation on
the selected memory cell 720. The reading voltage Vread may be
provided from outside, e.g., the memory controller 100, via the
input/output circuit 240. The reading voltage Vread may be a
voltage for reading data recorded on the selected memory cell 720.
From among the plurality of bit lines BL, the bit lines BL that are
not connected to the selected memory cell 720 may be grounded or
floated. As such, the reading operation may be performed on the
selected memory cell 720.
[0147] FIG. 14 is a diagram regarding a current movement in the
selected memory cell 720 in the reading mode of FIG. 13.
[0148] In the reading mode, the reading voltage Vread is applied to
the bit line BL connected to the selected memory cell 720 and the
turn-on voltage Von is applied to the compensation memory cell 710
and the non-selected memory cell 730, and thus a semiconductor
layer 522a in the compensation memory cell 710 and a semiconductor
layer 522c in the non-selected memory cell 730 have a conductive
property. Thus, a reading current Iread flows through the
semiconductor layer 522a of the compensation memory cell 710 and
the semiconductor layer 522c of the non-selected memory cell 730.
However, since the turn-off voltage Voff is applied to the selected
memory cell 720, the reading current Iread only flows on the
variable resistance layer 523b of the selected memory cell 720 and
the reading operation may be performed on the selected memory cell
720.
[0149] A resistance state of the variable resistance layer 523
generally has a distribution in a log scale (dynamic range). Thus,
a ratio of a maximum resistance with respect to a minimum
resistance of the variable resistance layer 523 may be large and a
deviation may be also large. The variation in the resistance of the
variable resistance layer 523 may exceed a limit of a dynamic range
of a sense amplifier that senses the resistance. Thus, it may be
difficult to bond the sense amplifier to the memory cell MC or the
memory device.
[0150] FIG. 15 is a diagram showing a circuit during a reading mode
of a memory block according to another embodiment. Referring to
FIGS. 2, 6A, and 15, during the reading mode, the control logic 250
may apply the turn-on voltage Von to the string selection line SSL
connected to the selected memory cell 720 and may apply the
turn-off voltage Voff to the string selection line that is not
connected to the selected memory cell 720, from among the plurality
of string selection lines SSL. In addition, the control logic 250
may apply the turn-on voltage Von to the word line WL, from among
the plurality of word lines WL, connected to the compensation
memory cell 710 and the non-selected memory cell 730.
[0151] The control logic 250 may apply a current-on voltage Vion to
the word line WL connected to the selected memory cell 720. The
current-on voltage Vion may have a magnitude that may allow the
electric current to flow on both the semiconductor layer 522 and
the variable resistance layer 523 of the transistor included in the
selected memory cell 720. An absolute value of the current-on
voltage Vion may be greater than that of the turn-off voltage Voff
and less than that of the turn-on voltage Von. The value of the
current-on voltage Vion may vary depending on the kinds, thickness,
etc. of the materials included in the gates 531, the gate
insulating layer 521, the semiconductor layer 522, and the variable
resistance layer 523 in the plurality of memory cells MC. In
particular, the current-on voltage Vion may allow a resistance
distribution of the selected memory cell 720 to have a linear
scale.
[0152] In addition, the reading voltage Vread may be applied to a
bit line BL connected to the selected memory cell 720, from among
the plurality of bit lines BL. The reading voltage Vread may be
provided from outside, e.g., the memory controller 100 (see FIG.
1), via the input/output circuit 240. From among the plurality of
bit lines BL, the bit lines BL that are not connected to the
selected memory cell 720 may be grounded or floated. As such, the
reading operation may be performed on the selected memory cell
720.
[0153] FIG. 16 is a diagram regarding a current movement in the
selected memory cell 720 during the reading mode of FIG. 15.
[0154] In the reading mode, the reading voltage Vread is applied to
the bit line BL connected to the selected memory cell 720 and the
turn-on voltage Von is applied to the non-selected memory cell 730,
and thus the semiconductor layer 522c in the non-selected memory
cell 730 have a conductive property. Therefore, the reading current
Iread flows through the semiconductor layer 522c of the
non-selected memory cell 730. However, since the current-on voltage
Vion is applied to the selected memory cell 720, the reading
current Iread flows on both the semiconductor layer 522b and the
variable resistance layer 523b of the selected memory cell 720.
[0155] The current-on voltage Vion may have a magnitude that allows
the resistance of the semiconductor layer 522b of the selected
memory cell 720 to be in a similar range to that of the variable
resistance layer 523b of the selected memory cell 720. By the
magnitude of the current-on voltage Vion, the resistance of the
semiconductor layer 522b of the selected memory cell 720 is equal
to or greater than a minimum resistance of the variable resistance
layer 523b of the selected memory cell 720 or equal to or less than
a maximum resistance of the variable resistance layer 523c of the
selected memory cell 720. Alternatively, when the resistance of the
variable resistance layer 523b ranges from 10.sup.4 .OMEGA.m.sup.-1
to 10.sup.12 .OMEGA.m.sup.-1, the magnitude of the current-on
voltage Vion may be in a range, in which the resistance of the
semiconductor layer 522b also ranges from 10.sup.4 .OMEGA.m.sup.-1
to 10.sup.12 .OMEGA.m.sup.-1. Alternatively, the magnitude of the
current-on voltage Vion may allow a ratio of a maximum value with
respect to a minimum value of a combined resistance of the
semiconductor layer 522b and the variable resistance layer 523b in
the selected memory cell 720 to be 10 or less. Thus, the total
resistance of the selected memory cell 720 may be determined as a
parallel resistance of the semiconductor layer 522b and the
variable resistance layer 523b.
[0156] Since the total resistance of the selected memory cell 720
is determined as the resistance of the semiconductor layer 522b and
the variable resistance layer 523b connected in parallel, the total
resistance of the selected memory cell 720 may have a linear scale
distribution even when the resistance state of the variable
resistance layer 523b is in the log scale. Therefore, uniformity of
the resistance state with respect to the memory cells MC may be
improved and a current within a certain range may be output from
the selected memory cell 720, and thus the bonding property with
respect to the sense amplifier that senses the selected memory cell
720 may be improved.
[0157] According to the disclosure, the resistance states of
various levels may be generated on the selected memory cell by
controlling the magnitude of the current flowing on the selected
memory cell. Since the memory cell may have the resistance states
of various levels, more information may be stored on the memory
cell. In the reading mode, by applying the voltage within a range,
in which the semiconductor layer, as well as the variable
resistance layer, may become a resistance, to the memory cell of
the non-volatile memory device, the resistance value of the memory
cell may be distributed in the linear scale range. This may improve
the uniformity of the current output from the non-volatile memory
device. In addition, it may be easy to bond to the sense amplifier
that detects the electric current of the non-volatile memory
device.
[0158] The above description of the disclosure is provided for the
purpose of illustration, and it would be understood by those
skilled in the art that various changes and modifications may be
made without changing technical conception and essential features
of the disclosure. Thus, the embodiments of the disclosure set
forth herein or shown above are to be interpreted in an
illustrative and non-limiting sense. For example, each component
described to be of a single type can be implanted in a distributed
manner. Likewise, components described to be distributed can be
implanted in a combined manner.
[0159] It should be understood that embodiments described herein
should be considered in a descriptive sense only and not for
purposes of limitation. Descriptions of features or aspects within
each embodiment should typically be considered as available for
other similar features or aspects in other embodiments. While one
or more embodiments have been described with reference to the
figures, it will be understood by those of ordinary skill in the
art that various changes in form and details may be made therein
without departing from the spirit and scope as defined by the
following claims.
* * * * *