U.S. patent application number 16/855381 was filed with the patent office on 2020-11-05 for microfluidic devices and methods of making the same.
The applicant listed for this patent is E INK CORPORATION. Invention is credited to Timothy J. O'MALLEY, Richard J. PAOLINI, JR., David ZHITOMIRSKY.
Application Number | 20200347840 16/855381 |
Document ID | / |
Family ID | 1000004812301 |
Filed Date | 2020-11-05 |
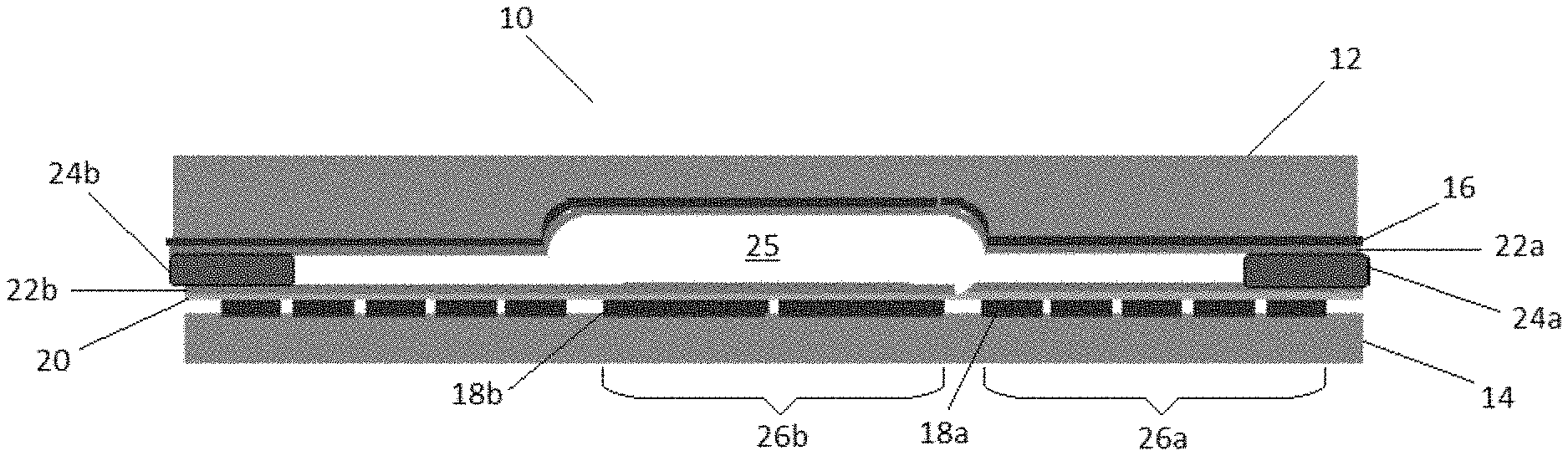

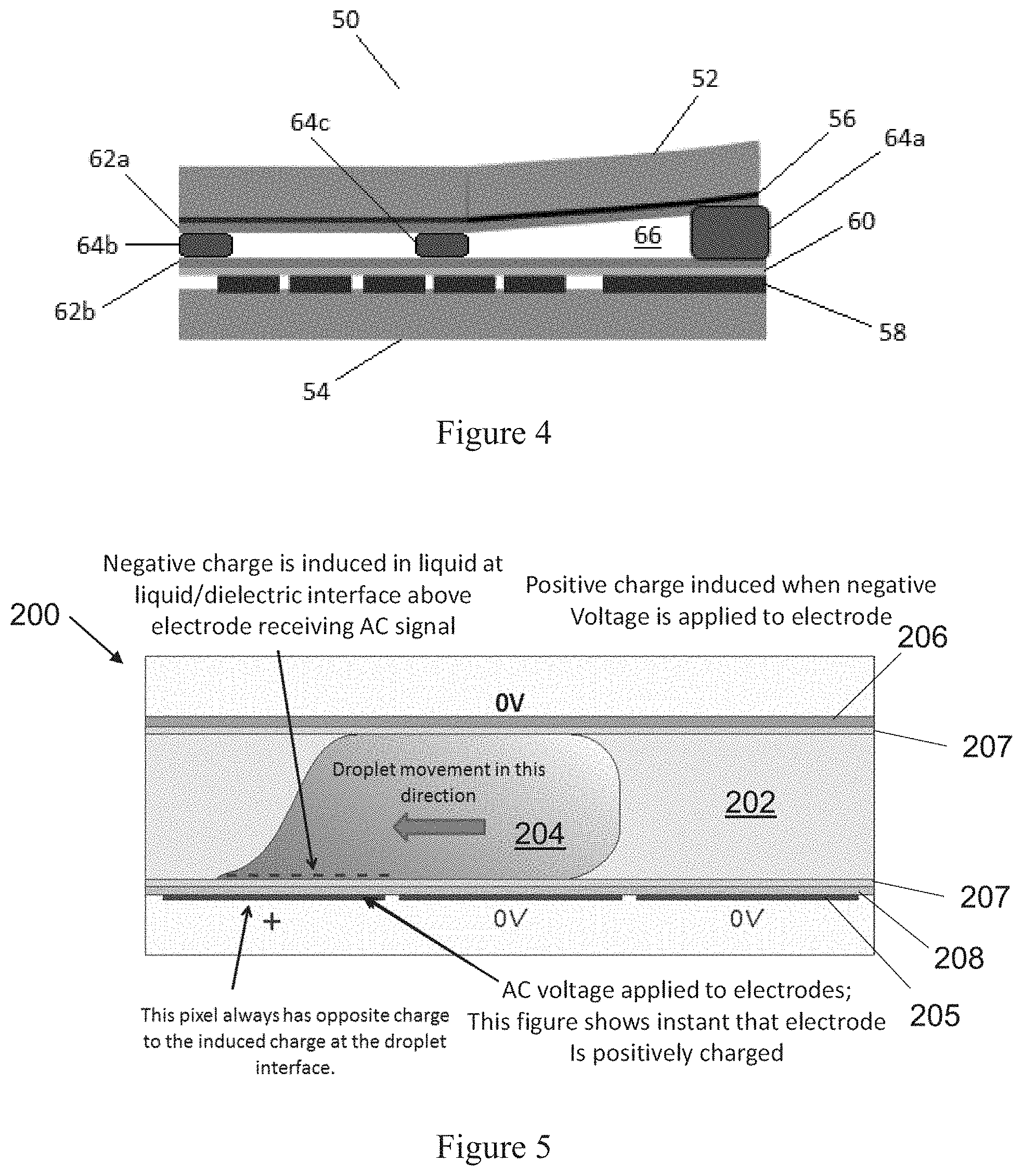
United States Patent
Application |
20200347840 |
Kind Code |
A1 |
PAOLINI, JR.; Richard J. ;
et al. |
November 5, 2020 |
MICROFLUIDIC DEVICES AND METHODS OF MAKING THE SAME
Abstract
A microfluidic device including: (a) top plate, including: a top
substrate; a first layer of hydrophobic material coupled to a
surface of the top substrate; a continuous electrode between the
first layer of hydrophobic material and the top substrate; (b) a
bottom plate, comprising: a bottom substrate; a plurality of
electrodes coupled to the bottom substrate; a second layer of
hydrophobic material coupled to the second substrate and atop the
plurality of electrodes. The top plate and the bottom plate are
placed in a spaced relationship, thereby defining a gap between the
first and second layers of hydrophobic material to permit droplet
motion within the gap under application of propulsion voltages. At
least one of the top substrate, the first layer of hydrophobic
material, the second layer of hydrophobic material, the bottom
substrate, and the plurality of electrodes have a non-uniform
thickness, and the gap has a plurality of heights.
Inventors: |
PAOLINI, JR.; Richard J.;
(Framingham, MA) ; ZHITOMIRSKY; David; (Woburn,
MA) ; O'MALLEY; Timothy J.; (Westford, MA) |
|
Applicant: |
Name |
City |
State |
Country |
Type |
E INK CORPORATION |
Billerica |
MA |
US |
|
|
Family ID: |
1000004812301 |
Appl. No.: |
16/855381 |
Filed: |
April 22, 2020 |
Related U.S. Patent Documents
|
|
|
|
|
|
Application
Number |
Filing Date |
Patent Number |
|
|
62840443 |
Apr 30, 2019 |
|
|
|
Current U.S.
Class: |
1/1 |
Current CPC
Class: |
F04B 19/006
20130101 |
International
Class: |
F04B 19/00 20060101
F04B019/00 |
Claims
1. A microfluidic device comprising: (a) top plate, comprising: a
top substrate; a first layer of hydrophobic material coupled to a
surface of the top substrate; a continuous electrode between the
first layer of hydrophobic material and the top substrate; (b) a
bottom plate, comprising: a bottom substrate; a plurality of
electrodes coupled to the bottom substrate; a second layer of
hydrophobic material coupled to the second substrate and atop the
plurality of electrodes; wherein the top plate and the bottom plate
are placed in a spaced relationship, thereby defining a gap between
the first and second layers of hydrophobic material to permit
droplet motion within the gap under application of propulsion
voltages, and wherein at least one of the top substrate, the first
layer of hydrophobic material, the second layer of hydrophobic
material, the bottom substrate, and the plurality of electrodes has
a non-uniform thickness, and the gap has a plurality of
heights.
2. The microfluidic device of claim 1, wherein the top substrate
has a uniform thickness and the microfluidic device further
includes an additive material between the top substrate and the
first layer of hydrophobic material.
3. The microfluidic device of claim 1 wherein the top plate is
attached to the bottom plate, such that the top plate is fixed
relative to the bottom plate.
4. The microfluidic device of claim 1 further comprising a layer of
dielectric material between the plurality of electrodes and the
second layer of hydrophobic material.
5. The microfluidic device of claim 1, wherein the first and second
layers of hydrophobic material are located on opposing sides of the
gap.
6. The microfluidic device of claim 1 further comprising one or
more spacers between the top and bottom substrate.
7. The microfluidic device of claim 1, wherein the plurality of
electrodes comprises two or more sets of electrodes and a side
length of each electrode of a first set differs from the side
length of each electrode of a second set.
8. The microfluidic device of claim 1, wherein the microfluidic
device includes a plurality of operation-specific zones comprising
a transport zone, a splitting zone, a dispensing zone, and a mixing
zone.
9. The microfluidic device of claim 8, wherein the height of the
gap within one of the operation-specific zones differs from the
height of the gap within at least one of the other
operation-specific zones.
10. A method of making a microfluidic device comprising: providing
a first substrate and a second substrate, at least one of the first
and second substrates having a plurality of thicknesses; applying a
first layer of hydrophobic material to a surface of the first
substrate, to form a top plate; applying a plurality of electrodes
to a surface of the second substrate and a second layer of
hydrophobic material on the plurality of electrodes, to form a
bottom plate; and placing the bottom plate in a spaced relationship
defining a gap between the first and second layers of hydrophobic
material to permit droplet motion within the gap under application
of propulsion voltages.
11. The method of claim 10, wherein the plurality of electrodes
comprises two or more sets of electrodes and a side length of each
electrode of a first set differs from the side length of each
electrode of a second set.
12. The method of claim 10, wherein the plurality of thicknesses is
formed by etching at least one of the first and second substrates,
the etching step comprising applying a pattern of a resist to the
surface of at least one of the first and second substrates and
exposing the surface to a chemical etchant.
13. The method of claim 10, wherein the plurality of thicknesses is
formed by applying additive material to the surface of at least one
of the first and second substrates.
14. The method of claim 10, wherein the plurality of thicknesses is
provided by molding at least one of the first and second substrates
into a pre-selected form prior to providing the first and second
substrates.
15. A method of making a microfluidic device comprising: providing
a first substrate and a second substrates; applying a first layer
of hydrophobic material to a surface of the first substrate, to
form a top plate; applying a plurality of electrodes to a surface
of the second substrate and a second layer of hydrophobic material
on the plurality of electrodes, to form a bottom plate; and placing
the bottom plate in a spaced relationship defining a gap having a
plurality of heights between the first and second layers of
hydrophobic material to permit droplet motion within the gap under
application of propulsion voltages.
16. The method of claim 15, wherein the plurality of heights is
provided by deforming at least one of the first and second
substrates during or after the placing step.
17. The method of claim 15, wherein the plurality of heights is
provided by applying a plurality of electrodes having more than one
thickness.
18. The method of claim 15, wherein the plurality of electrodes
comprises two or more sets of electrodes and a side length of each
electrode of a first_set differs from the side length of each
electrode of a second set.
Description
REFERENCE TO RELATED APPLICATIONS
[0001] This application claims priority to U.S. Patent Application
No. 62/840,443 filed on Apr. 30, 2019, which is incorporated by
reference herein in its entirety.
BACKGROUND
[0002] Digital microfluidic devices use independent electrodes to
move droplets in a confined environment, thereby providing a
"lab-on-a-chip." Digital microfluidic devices are alternatively
referred to as electrowetting on dielectric, or "EWoD," to further
differentiate the method from competing microfluidic systems that
rely on electrophoretic flow and/or micropumps. A 2012 review of
the electrowetting technology was provided by Wheeler in "Digital
Microfluidics," Annu. Rev. Anal. Chem. 2012, 5:413-40, which is
incorporated herein by reference in its entirety. The technique
allows sample preparation, assays, and synthetic chemistry to be
performed with tiny quantities of both samples and reagents. In
recent years, controlled droplet manipulation in microfluidic cells
using electrowetting has become commercially-viable; and there are
now products available from large life science companies, such as
Oxford Nanopore.
[0003] Most of the literature reports on EWoD involve so-called
"passive matrix" devices (a.k.a. "segmented" devices), whereby ten
to twenty electrodes are directly driven with a controller. While
segmented devices are easy to fabricate, the number of electrodes
is limited by space and driving constraints. Accordingly, it is not
possible to perform massive parallel assays, reactions, etc. in
passive matrix devices. In comparison, "active matrix" devices
(a.k.a. active matrix EWoD, a.k.a. AM-EWoD) devices can have many
thousands, hundreds of thousands or even millions of addressable
electrodes. The electrodes are typically switched by thin-film
transistors (TFTs) and droplet motion is programmable so that
AM-EWoD arrays can be used as general purpose devices that allow
great freedom for controlling multiple droplets and executing
simultaneous analytical processes.
[0004] The fundamental operation of a traditional EWoD device is
illustrated in the sectional image of FIG. 5. The EWoD 200 includes
a cell filled with an oil 202 and at least one aqueous droplet 204.
In a basic configuration, as shown in FIG. 5, a plurality of
propulsion electrodes 205 are disposed on one substrate and a
singular top electrode 206 is disposed on the opposing surface. The
cell additionally includes hydrophobic coatings 207 on the surfaces
contacting the oil layer, as well as a dielectric layer 208 between
the propulsion electrodes 205 and the hydrophobic coating 207. (The
upper substrate may also include a dielectric layer, but it is not
shown in FIG. 5). The hydrophobic layer prevents the droplet from
wetting the surface. When no voltage differential is applied
between adjacent electrodes, the droplet will maintain a spheroidal
shape to minimize contact with the hydrophobic surfaces (oil and
hydrophobic layer). Because the droplets do not wet the surface,
they are less likely to contaminate the surface or interact with
other droplets except when that behavior is desired. When a voltage
differential is applied between adjacent electrodes, the voltage on
one electrode attracts opposite charges in the droplet at the
dielectric-to-droplet interface, and the droplet moves toward this
electrode.
[0005] As illustrated in FIG. 5, EWoD devices typically comprise
two parallel substrates. The operations for moving the droplets
within the gap between the two substrates generally fall under one
of four main operations: transport (i.e. laterally moving a droplet
through the gap), splitting (i.e. dividing a droplet into two or
more droplets of lesser volume), dispensing (i.e. extracting a
droplet from a large reservoir of fluid), and mixing/merging (i.e.
the combination of two droplets into one drop of larger volume).
The efficiency of each of the operations is dependent on several
factors. For example, the voltages needed for acceptable droplet
propulsion depend on the properties of the dielectric and
hydrophobic layers. However, another critical factor is the aspect
ratio (h/L) of the droplet. The height of the droplet ("h") will
equal the gap height between the two parallel substrates because
the droplet will span this entire dimension. The length ("L")
equals the width or the dimension of the droplet perpendicular to
the height. There is an optimal aspect ratio for a droplet
depending on the operation to be performed. For example, if the
aspect ratio of the droplet within a zone of the device dedicated
for a transport operation is too low (i.e. the length of the
droplet is too great), a higher driving voltage may be required to
move the droplet due to the large contact area between the droplet
and the hydrophobic layers. Splitting operations may be impossible
to perform for droplets having too high of an aspect ratio (i.e.
the length of the droplet is too small). Due to manufacturing
constraints, the design of EWoD devices generally have a uniform
gap height and include electrodes having uniform dimensions;
thereby, providing a limit on the achievable aspect ratios for
various regions of the device. Thus, there is a need for improved
EWoD devices capable of more efficient droplet propulsion
operations and cost-effective methods for their manufacture.
SUMMARY OF INVENTION
[0006] In a first aspect, the present application provides a
microfluidic device comprising: (a) top plate, comprising: a top
substrate; a first layer of hydrophobic material coupled to a
surface of the top substrate; a continuous electrode between the
first layer of hydrophobic material and the top substrate; (b) a
bottom plate, comprising: a bottom substrate; a plurality of
electrodes coupled to the bottom substrate; a second layer of
hydrophobic material coupled to the second substrate and atop the
plurality of electrodes. The top plate and the bottom plate are
placed in a spaced relationship, thereby defining a gap between the
first and second layers of hydrophobic material to permit droplet
motion within the gap under application of propulsion voltages. At
least one of the top substrate, the first layer of hydrophobic
material, the second layer of hydrophobic material, the bottom
substrate, and the plurality of electrodes has a non-uniform
thickness, and the gap has a plurality of heights.
[0007] In a second aspect, the present application provides a
method of making a microfluidic device comprising: providing a
first substrate and a second substrate, at least one of the first
and second substrates having a plurality of thicknesses; applying a
first layer of hydrophobic material to a surface of the first
substrate, to form a top plate; applying a plurality of electrodes
to a surface of the second substrate and a second layer of
hydrophobic material on the plurality of electrodes, to form a
bottom plate; and placing the bottom plate in a spaced relationship
defining a gap between the first and second layers of hydrophobic
material to permit droplet motion within the gap under application
of propulsion voltages.
[0008] In a third aspect, the present application provides a method
of making a microfluidic device comprising: providing a first
substrate and a second substrate; applying a first layer of
hydrophobic material to a surface of the first substrate, to form a
top plate; applying a plurality of electrodes to a surface of the
second substrate and a second layer of hydrophobic material on the
plurality of electrodes, to form a bottom plate; and placing the
bottom plate in a spaced relationship defining a gap having a
plurality of heights between the first and second layers of
hydrophobic material to permit droplet motion within the gap under
application of propulsion voltages.
[0009] These and other aspects of the present invention will be
apparent in view of the following description.
BRIEF DESCRIPTION OF DRAWINGS
[0010] The drawing Figures depict one or more implementations in
accord with the present concepts, by way of example only, not by
way of limitations. The drawings are not to scale. In the figures,
like reference numerals refer to the same or similar elements.
[0011] FIG. 1 is a schematic cross-sectional side view of an EWoD
device according to a first embodiment of the present
invention.
[0012] FIG. 2 is a schematic cross-sectional side view of an EWoD
device according to a second embodiment of the present
invention.
[0013] FIG. 3 is a schematic cross-sectional side view of an EWoD
device according to a third embodiment of the present
invention.
[0014] FIG. 4 is a schematic cross-sectional side view of an EWoD
device according to a fourth embodiment of the present
invention.
[0015] FIG. 5 is a schematic cross-sectional side view of a
traditional EWoD device.
DETAILED DESCRIPTION
[0016] In the following detailed description, numerous specific
details are set forth by way of examples in order to provide a
thorough understanding of the relevant teachings. However, it
should be apparent to those skilled in the art that the present
teachings may be practiced without such details.
[0017] The various embodiments of the present invention provide an
EWoD device including dual substrates. As depicted herein the
"bottom" substrate includes a plurality of electrodes to propel
various droplets through a microfluidic region. The "top" substrate
includes layer of conductive material, which serves as a common
conductor. The use of "top" and "bottom" is merely a convention as
the locations of the two substrates can be switched, and the
devices can be oriented in a variety of ways, for example, the top
and bottom substrates can be roughly parallel while the overall
device is oriented so that the substrates are normal to a work
surface (as opposed to parallel to the work surface as shown in the
figures). The top or the bottom substrate may include additional
functionality, such as resistive heating and/or temperature
sensing. Various materials that may be used to form the top and/or
bottom substrate include, but are not limited to, glass and other
oxides, semiconductor materials (e.g. silicon), plastics (e.g.
acrylics), and combinations thereof.
[0018] The term "coupled", refers to any director indirect
structural coupling or connection between two or more parts of a
device, where the parts may either be in direct physical contact or
coupled through an intermediate part, for example and intermediate
layer, member or adhesive. By way of example, a layer "coupled" to
a substrate may in certain instances refer to a configuration where
the layer is directly adjacent to and in physical contact with the
substrate. However, depending on the context, one or more
additional layers or other parts may be interposed between the
layer and substrate.
[0019] Referring now to FIG. 1, a microfluidic device 10 comprises
a top plate and bottom plate. The top plate includes a top
substrate 12, and the bottom plate includes bottom substrate 14.
The two plates are generally parallel to each other, as well as
being fixed relative to each other. As used herein, "fixed` means
that the device 10 does not include any mechanical or
electro-mechanical parts that would allow the distance between the
top and bottom plates to be variably adjusted. In one embodiment,
the top and bottom plates are pressed together to form a reversible
attachment. Alternatively, the attachment may be secured with
removable or permanent fasteners.
[0020] The top substrate 12 includes an interior surface to which a
continuous layer of conductive material 16 may be applied to most,
if not all, of the interior surface. A layer of hydrophobic
material 22a may then be applied over the continuous electrode 16.
The entire area of the continuous electrode 16 is preferably coated
with the layer of hydrophobic material 22a. The conductive material
of the continuous electrode includes, but is not limited to, metal
oxides (e.g. indium tin oxide) and conductive polymers (PEDOT:PSS).
The hydrophobic layer 22a may be manufactured from hydrophobic
materials formed into coatings by deposition onto a surface via
suitable techniques. Depending on the hydrophobic material to
be_applied, example deposition techniques include spin coating,
molecular vapor deposition, and chemical vapor deposition.
Hydrophobic layers may be more or less wettable as usually defined
by their respective contact angles. Unless otherwise specified,
angles are herein measured in degrees (.degree.) or radians (rad),
according to context. For the purpose of measuring the
hydrophobicity of a surface, the term "contact angle" is understood
to refer to the contact angle of the surface in relation to
deionized (DI) water. If water has a contact angle between
0.degree.<.theta.<90.degree., then the surface is classed as
hydrophilic, whereas a surface producing a contact angle between
90.degree.<.theta.<180.degree. is considered hydrophobic.
Usually, moderate contact angles are considered to fall in the
range from about 90.degree. to about 120.degree., while high
contact angles are typically considered to fall in the range from
about 120.degree. to about 150.degree.. In instances where the
contact angle is 150.degree.<.theta. then the surface is
commonly known as superhydrophobic or ultrahydrophobic. Surface
wettabilities may be measured by analytical methods well known in
the art, for instance by dispensing a droplet on the surface and
performing contact angle measurements using a contact angle
goniometer. Anisotropic hydrophobicity may be examined by tilting
substrates with gradient surface wettability along the transverse
axis of the pattern and examining the minimal tilting angle that
can move a droplet.
[0021] Hydrophobic layers of moderate contact angle typically
include one or a blend of fluoropolymers, such as PTFE
(polytetrafluoroethylene), FEP (fluorinated ethylene propylene),
PVF (polyvinylfluoride), PVDF (polyvinylidene fluoride), PCTFE
(polychlorotrifluoroethylene), PFA (perfluoroalkoxy polymer), FEP
(fluorinated ethylenepropylene), ETFE
(polyethylenetetrafluoroethylene), and ECTFE
(polyethylenechlorotrifluoroethylene). Commercially available
fluoropolymers include Cytop.RTM. (AGC Chemicals, Exton, Pa.) and
Teflon.RTM. AF (Chemours, Wilmington, Del.). An advantage of
fluoropolymer films is that they can be highly inert and can remain
hydrophobic even after exposure to oxidizing treatments such as
corona treatment and plasma oxidation.
[0022] The bottom substrate 14 has an interior surface to which a
plurality of electrodes 18a, 18b may be applied. The electrodes may
be a passive matrix or an active matrix, such as a TFT array. A
layer of dielectric material 20 is coated over the plurality of
electrodes, preferably over the entire area of the electrodes, as
well as a layer of hydrophobic material 22b that may the same or
similar to the composition of the hydrophobic material layer 22a
applied to the top substrate. The dielectric layer may comprise a
layer of approximately 20-40 nm SiO.sub.2 topped over-coated with
200-400 nm plasma-deposited silicon nitride. Alternatively, the
dielectric may comprise atomic-layer-deposited Al.sub.2O.sub.3
between 2 and 100 nm thick, preferably between 20 and 60 nm thick.
While it is possible to have a single layer for both the dielectric
and hydrophobic functions, such layers typically require thick
inorganic layers (to prevent pinholes) with resulting low
dielectric constants, thereby requiring more than 100V for droplet
movement. To achieve low voltage actuation, it is better to have a
thin dielectric inorganic layer for high capacitance and to be
pinhole free, topped by a thin organic hydrophobic layer. With this
combination it is possible to have electrowetting operation with
voltages in the range +/-10 to +/-50V, which is in the range that
can be supplied by conventional TFT arrays. AC driving is used to
reduce degradation of the droplets, dielectrics, and electrodes by
various electrochemistries. Operational frequencies for EWoD can be
in the range 100 Hz to 1 MHz, but lower frequencies of 1 kHz or
lower are preferred for use with TFTs that have limited speed of
operation. Examples of various architectures for the electrodes and
driving methods are disclosed in U.S. patent application Ser. No.
16/161,548, the entire content of which is incorporated by
reference herein.
[0023] As previously mentioned, the top plate and bottom plate may
fixed relative to each other, for example by pressing together
until a reversible attachment is established. The distance and
separation between the top plate and bottom plate may be maintained
by one or more spacers 24a, 24b in order to provide a gap 25
between the substrates 12, 14. The gap 25 is preferably filled with
a gap fluid. The droplet samples propelled within the device should
not be miscible in the gap fluid. For example, if the microfluidic
device is used to perform analyses on aqueous droplet samples, it
is usually preferred that the gap fluid is a hydrophobic fluid,
such as silicone oil, dodecane, or other long-chain, non-polar
hydrocarbon oils.
[0024] As illustrated in FIG. 1, the top substrate 12 does not have
a uniform thickness. Instead, the thickness of the top substrate 12
within one cross-sectional area 26b may be less than the thickness
of the top substrate 12 within an adjacent cross-sectional area
26a. As a result, the height of the gap 25 in area 26a is less than
the gap height in area 26b. Each of the areas 26a, 26b may be an
operation-specific zone. For example, area 26a having a small gap
height may be an operation-specific zone dedicated to a splitting
operation, while the area 26b having the larger gap height may be a
transport zone. Each operation-specific zone (e.g. transport zone,
dispensing zone, splitting zone, and mixing zone) may have a gap
height that is different than the gap height of at least one of the
other operation-specific zones. Each gap height is typically in the
range 50 to 200 .mu.m, but the gap can be larger.
[0025] In order to provide the top substrate 12 with a non-uniform
thickness, the top substrate 12 may be made from an etchable
material, such as glass. An etching process, such as a wet etching
process, may be performed by first applying a resist to a surface
of a glass substrate in a desired pattern. The patterned surface
may then be exposed to a chemical etchant for a specified amount of
time in order to remove a portion of the exposed glass. After a
sufficient amount of material has been chemically etched away, the
patterned surface may be washed to remove the resist and any
residual etchant. Dry etching processes (e.g. plasma or
photo-induced etching) may also be used to provide the top
substrate with a non-uniform thickness.
[0026] In another method according to an embodiment of the present
invention, one or both substrates of the microfluidic device may be
molded or formed to provide a non-uniform surface. For example, a
stamp having an inverse of the desired pattern for the surface of
the substrate may be applied to one or both substrates, preferably
prior to the application of any of the additional layers. Another
method of providing one of the substrates with a non-uniform
thickness would be by embossing. For example, the substrate may be
made of an embossable material, such as a plastic film, and an
embossing roller having an inverse of the desired pattern may be
applied to the surface of the substrate material. The embossing
process may be a roll-to-roll processes, such as those described in
U.S. Pat. No. 6,930,818, the entire content of which is
incorporated by reference herein. Embossing procedures may be used
to provide the surface of the substrate with the desired surface
topography after being coated (e.g. after the top substrate is
coated with a layer of conductive material or the layer of
hydrophobic material). However, it is preferred that the embossing
is performed prior to application of these layers.
[0027] In some embodiments, both the top and bottom substrate may
be provided with non-uniform thicknesses; however, providing the
bottom substrate with a non-uniform surface topography is less
preferred because applying an array of electrodes to a non-uniform
surface may be more difficult and therefore, more costly, than
coating a continuous layer of conductive material to a non-uniform
surface on the top substrate. Therefore, it is preferred that of
the two substrates, only the substrate carrying the continuous
conductor is provided with a surface topography. In another aspect
of the present invention, the top substrate may be changed with
another top substrate having a different surface topography.
Therefore, various embodiments of the present invention may be
provided in the form of a kit comprising a bottom substrate and a
plurality of top substrates each having a different surface
topography that are configured to be releasably attached to the
bottom substrate, such as with fasteners. This would allow the user
to select from various top substrate options depending on the
desired operations to be performed within the microfluidic
device.
[0028] As illustrated in FIG. 1, the electrodes on the bottom
substrate 14 within one cross-sectional area 26a may have a shorter
side length than the electrodes on the bottom substrate 14 within
another cross-sectional area 26b. Therefore, devices made according
to one embodiment of the invention may have a specific electrode
width depending on the gap height 25 of the cross-sectional area in
which the electrode is located. This may provide more control over
how a desired aspect ratio may be achieved for a droplet within
certain areas of the device 10. Due to the difficulty of forming an
array of electrodes having varying sizes, it is preferred to
provide the bottom substrate 14 with an array of substantially
uniform side lengths. For microfluidic devices having an array of
similarly sized driving electrodes, the cross-sectional areas in
which a larger electrode side length is desired may be achieved by
ganging a group of electrodes together, such that the gang of
electrodes to operate in unison. Generally, an aspect ratio of
approximately 1:2, more preferably about 1:3, should be maintained
for effective performance of each propulsion operation. Therefore,
the width of the droplet formed by the electrode or gang of
electrodes should be approximately three times greater than the
height.
[0029] As an alternative to removing or etching material, a
microfluidic device with a gap having a plurality of heights
according to various embodiments of the present invention may be
made by applying a pattern of additive material to an interior
surface of one or both substrates. For example referring to FIG. 2,
a microfluidic device 30 is illustrated having the same features as
the previously described embodiment. The top plate of device 30 may
comprise a top substrate 32 having a layer of conductive material
forming a continuous electrode 38 and a layer of hydrophobic
material 42a applied to an internal surface of the top substrate
32, as well as a bottom plate comprising bottom substrate 34,
plurality of electrodes 40, a dielectric layer 41, and a layer of
hydrophobic material 42b also applied to an internal surface. One
or more spacers 44a, 44b maintain the top and bottom substrates 32,
34 apart to provide a gap 45 between the layers of hydrophobic
material 42a, 42b. The embodiment illustrated in FIG. 2 differs
from the previously described embodiment in that the top and bottom
substrates 32, 34 have a substantially uniform thickness, but an
additive material 36 has been applied in specific locations on the
interior surface of the top substrate 32 to reduce the height of
the gap 45 that is adjacent to the additive material 36.
[0030] In some embodiments, the additive material may be the same
material as the conductive material or the hydrophobic material.
However, with respect to the top substrate 32, it is less preferred
that the additive material 36 is the same material as the
conductive material of the continuous electrode 38 because a layer
of conductive material having a non-uniform thickness may cause a
variation in electrical properties across the device 30. The
additive material 36 may be applied to the substrate either before
or after application of the layer of conductive material 38,
preferably before. If the additive material 36 is not hydrophobic,
it is also preferred that the additive material 36 is applied prior
to the layer of hydrophobic material 42a. The additive material 36
may also be patterned onto the interior surface of the bottom
substrate 34 or over the plurality of electrodes 40. Using a
pattern of dielectric material 41 to provide the surface topography
however is a less preferred option, as it may cause non-uniform
electrical properties across the interior surface of the bottom
substrate 34. Types of additive materials that may be used to form
the surface topography of the substrates include, but are not
limited to, photoresist materials (e.g. SU-8), polymeric materials
(e.g. PMMA), and dielectric materials (e.g. inorganic oxides).
[0031] It may be desired in some applications for the microfluidic
device made according to the various embodiments of the present
invention to have a light transmissive top substrate and/or bottom
substrate, as well as the layers applied thereto, to perform
certain analytical procedures on the droplet samples within the gap
of the device. For example, fluorescent markers may be observed by
illuminating a droplet through the top substrate with a light
source and then using a detector and optionally colored filters to
observe the resulting fluorescence through the top substrate. In
other embodiments, the light may pass through both the top and
bottom substrates to allow absorption measurements in the IR, UV,
or visible wavelengths. Alternatively, attenuated (frustrated)
total-internal reflection spectroscopy can be used to probe the
contents and or location of droplets in the system.
[0032] There is no limitation as to how the pattern of the additive
material may be formed on the surface of one of the substrates of
the microfluidic device. For example if the additive material
comprises hydrophobic material, an initial uniform coating of
hydrophobic material may be applied to the conductive layer
followed by a pattern of additional hydrophobic material or vice
versa. In another method, one or more stencils or masks having the
desired pattern may be applied to the substrate prior to coating
the surface of the substrate with the additive material. The mask
may then be lifted to reveal the desired pattern. If multiple masks
are used, the coating of additive material is preferably dried
between applications. Once the pattern is formed, the layer of
conductive material and hydrophobic material may be applied over
the patterned additive material. In another method, a uniform layer
of additive material may be applied over the entire surface of a
substrate. A laser may be used to etch the additive material to
form the surface topography. Alternatively, one or more masks or a
resist may be applied in a pattern to a uniform coating of additive
material prior to the removal of any exposed material using a
chemical etchant. Again, the layer of conductive material and
hydrophobic material may be applied over the additive material
after etching and rinsing the surface pattern.
[0033] As previously noted, the top and/or bottom substrates may be
modified to include additive material to provide a device with a
plurality of gap heights. For example, as illustrated in FIG. 3, a
less preferred embodiment of the present invention is illustrated.
A microfluidic device 31 includes an array of short electrodes 40a
and tall electrodes 40b on the bottom substrate 34. Additional
conductive material is used to form the tall electrodes 40b and
thereby decrease the height of the gap 45 adjacent to the tall
electrodes 40b relative to the gap height adjacent to the short
electrodes 40a.
[0034] Referring now to FIG. 4, a microfluidic device 50 may be
formed by applying a plurality of spacers 64a, 64b, 64c having
various heights to the bottom substrate 54 after application of a
dielectric material 60 and hydrophobic layer 62b. A top substrate
52 having a common electrode layer 56 and hydrophobic layer 62a may
then be pressed and bonded onto the plurality of spacers 64a, 64b,
64c, such that the varying heights of the gap 66 are maintained by
the spacers 64a, 64b, 64c and the gap heights in any location
within the device 50 are dependent on the dimensions of the spacers
64a, 64b, 64c in the vicinity of the gap 66. In each of the
deformation processes, heat and/or pressure may be used to provide
a more pliable and deformable substrate and to facilitate bonding
of the top substrate to the spacers.
[0035] From the foregoing, it will be seen that the present
invention provides improved methods of fabricating devices to allow
for greater design selection in gap height at specific regions of
the device to match electrode dimensions for greater effective of
control over the propulsion operations of the same droplets within
the microfluidic device. For example, a customized gap height at
the location of reagent input reservoirs change the friction and
capillary forces for dispensing droplets into the device. Larger
gap heights create a more favorable capillary action. Smaller gap
heights with smaller electrodes may enable improved volume control
of droplets. Some operations may also be improved, such as mixing
wherein the droplet may be moved from an area with a large gap
height to an area with a smaller gap height. Smaller effective gap
heights may also enable the use of less reagent volumes. Reagents
for some analyses are very expensive, so the ability to perform
reactions and operations effectively with reduced reagent volumes
offers potential cost advantages.
[0036] While preferred embodiments of the invention have been shown
and described herein, it will be understood that such embodiments
are provided by way of example only. Numerous variations, changes,
and substitutions will occur to those skilled in the art without
departing from the spirit of the invention. Accordingly, it is
intended that the appended claims cover all such variations as fall
within the spirit and scope of the invention.
[0037] All of the contents of the aforementioned patents and
applications are incorporated by reference herein in their
entireties. In the event of any inconsistency between the content
of this application and any of the patents and application
incorporated by reference herein, the content of this application
shall control to the extent necessary to resolve such
inconsistency.
* * * * *