U.S. patent application number 16/833379 was filed with the patent office on 2020-10-01 for high porosity, low tortuosity, variable-pore-size structured topology for capillary wicks.
The applicant listed for this patent is The Government of the United States of America, as represented by the Secretary of the Navy, The Government of the United States of America, as represented by the Secretary of the Navy. Invention is credited to Jesse Maxwell, Austin Phoenix, Charles Tenney.
Application Number | 20200309469 16/833379 |
Document ID | / |
Family ID | 1000004763608 |
Filed Date | 2020-10-01 |


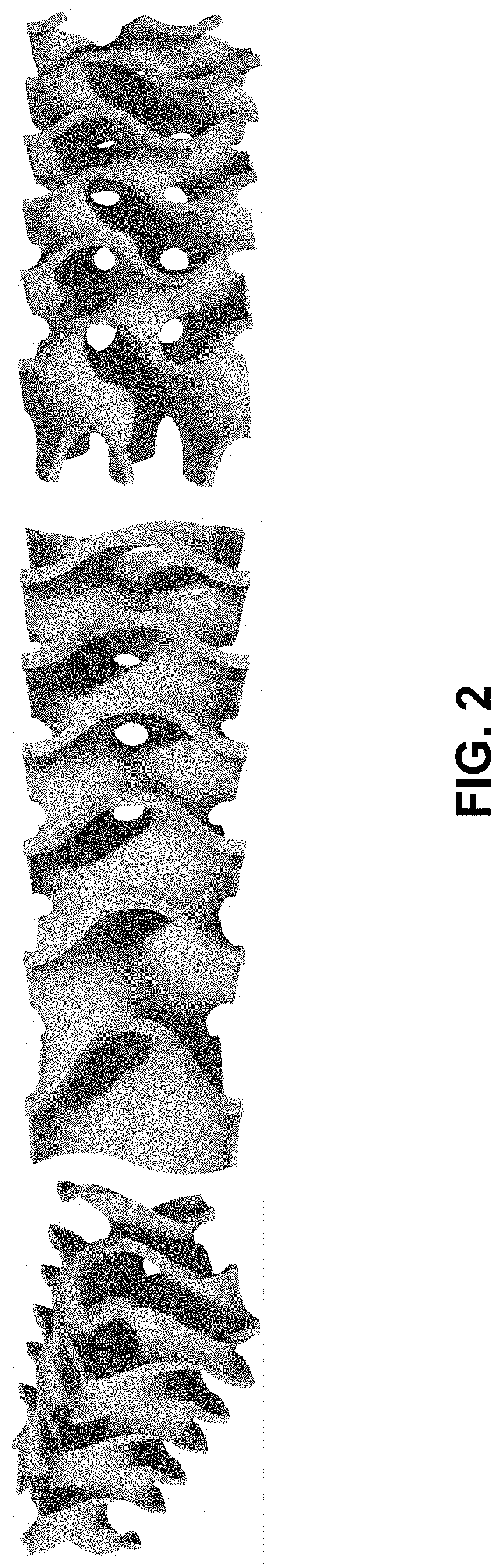



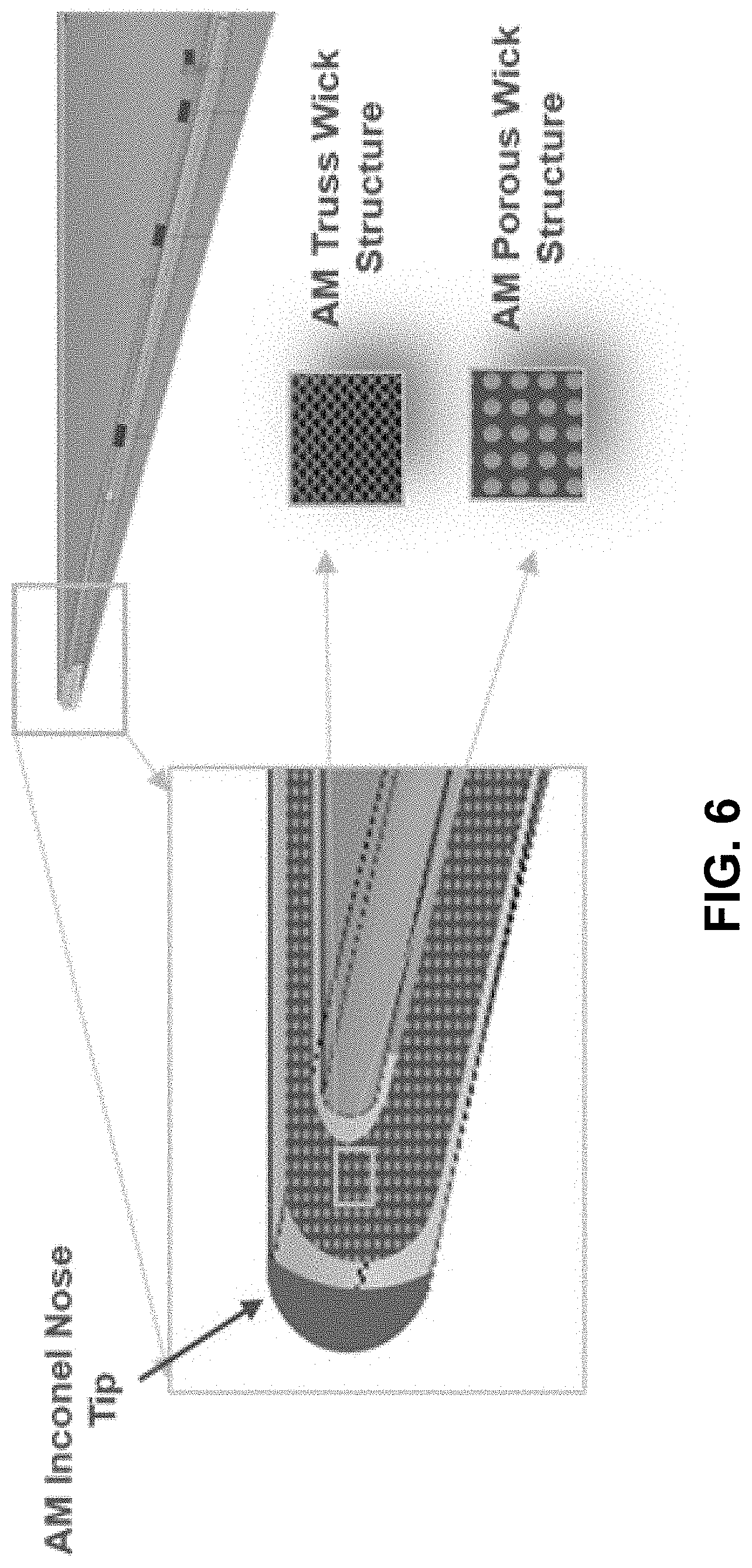
United States Patent
Application |
20200309469 |
Kind Code |
A1 |
Maxwell; Jesse ; et
al. |
October 1, 2020 |
HIGH POROSITY, LOW TORTUOSITY, VARIABLE-PORE-SIZE STRUCTURED
TOPOLOGY FOR CAPILLARY WICKS
Abstract
A heat pipe includes a a heat pipe wick having a gyroid or other
periodic lattice structure, thereby providing relatively high
porosity, high surface area, consistent pore size, structural
stability, low tortuosity, and low convective acceleration.
Inventors: |
Maxwell; Jesse; (Silver
Spring, MD) ; Phoenix; Austin; (Washington, DC)
; Tenney; Charles; (Washington, DC) |
|
Applicant: |
Name |
City |
State |
Country |
Type |
The Government of the United States of America, as represented by
the Secretary of the Navy |
Arlington |
CA |
US |
|
|
Family ID: |
1000004763608 |
Appl. No.: |
16/833379 |
Filed: |
March 27, 2020 |
Related U.S. Patent Documents
|
|
|
|
|
|
Application
Number |
Filing Date |
Patent Number |
|
|
62824693 |
Mar 27, 2019 |
|
|
|
Current U.S.
Class: |
1/1 |
Current CPC
Class: |
F28D 15/043 20130101;
F28D 15/046 20130101 |
International
Class: |
F28D 15/04 20060101
F28D015/04 |
Goverment Interests
FEDERALLY-SPONSORED RESEARCH AND DEVELOPMENT
[0002] The United States Government has ownership rights in this
invention. Licensing inquiries may be directed to Office of
Technology Transfer, US Naval Research Laboratory, Code 1004,
Washington, D.C. 20375, USA; +1.202.767.7230;
techtran@nrl.navy.mil, referencing NC 110707.
Claims
1. A heat pipe comprising: a heat pipe wick having a gyroid or
other periodic lattice structure, thereby providing relatively high
porosity, high surface area, consistent pore size, structural
stability, low tortuosity, and low convective acceleration.
2. The heat pipe of claim 1, wherein the heat pipe wick includes a
finite-order fractal cross-section, thereby providing relatively
high surface area and porosity for a given channel size.
3. The heat pipe of claim 1, wherein the heat pipe wick comprises
variable pore sizes.
4. The heat pipe of claim 3, wherein the variable pore sizes are
produced via scaling a basic geometry of the heat pipe wick.
5. The heat pipe of claim 3, wherein the variable pore sizes are
produced via skewing a basic geometry of the heat pipe wick.
6. The heat pipe of claim 3, wherein the variable pore sizes are
produced via implementing a basic geometry of the heat pipe wick on
multiple scales in the same bulk structure. The heat pipe of claim
1, integrated into a hypersonic leading edge.
8. The heat pipe of claim 1, wherein the heat pipe is a loop heat
pipe.
9. The heat pipe of claim 1, wherein the heat pipe is integrated
into a vapor chamber heat spreader.
10. The heat pipe of claim 1, wherein the heat pipe wick includes
local fractal or high surface roughness to increase capillary
force, and wherein the local fractal or high surface roughness is
configured to reduce overall pressure drop, thereby greatly
improving heat capacity.
Description
RELATED APPLICATIONS
[0001] This application claims the benefit of U.S. Provisional
Application No. 62/824,693 filed Mar. 27, 2019, which is hereby
incorporated herein by reference.
FIELD OF INVENTION
[0003] The present invention relates generally to capillary wicks,
and more particularly to variable-pore-size topology for capillary
wicks.
BACKGROUND
[0004] Advancements in small micro-electronic devices have
increased power and power density resulting in new challenges in
thermal management. Next-generation electronics cooling techniques
better-able to manage waste heat would provide an avenue to meet
the ever-increasing demands on computing performance.
[0005] Heat pipes can transport 10.sup.2-10.sup.4.times. as much
heat for the same temperature gradient as solid conduction heat
sinks, but they are limited by the pressure drop induced by the
small flow channel sizes. The development of higher-performance
heat pipes through the use of higher-performance wick geometries
represent a crucial development.
[0006] Conventionally, wicks are constructed using two methods. The
first method is a powder sintering process where the porosity is
dictated by the particle sizing. The second method uses a fine mesh
wound around a cylindrical core to provide the structured porosity.
These systems can be augmented by secondary sintering of powders
but do not allow arbitrary variation of the porosity throughout the
volume. Generally, these methods only deliver a single axis of
variable porosity: a gradient along the long axis of a cylinder for
example. A conventional method of creating the grading is by
stacking layers of differently-sized powders, then sintering the
whole group at once. Previous efforts at structured geometries like
trusses have also been demonstrated. Others have discussed the use
of truss structures for wicks using porous materials. These
previous efforts have explored the creation of truss structures
through a laser-melting process, but not the variation of porosity
on a macro/microstructured scale.
[0007] Tunable variable porosity provides significant performance
benefits relative to constant or single axis graded porosity
systems. This concept involves generating self-supporting porous
structures. These structures provide benefits by reducing the
complexity of the fluid path and mitigating the overall fluid
losses inherent to the structure. The use of multi-scale porosity
is also rarely seen in literature. Taking advantage of surface
roughness on a meso-scale truss structure can increase effective
capillary pressure by increasing the effective surface area.
SUMMARY OF INVENTION
[0008] A performance limitation of structured wicks is the
feature-size or wall-size limit. Every manufacturing method makes a
trade-off between minimal manufacturable feature size and wall
thickness. For a working fluid that operates optimally when paired
with larger porosities, the manufacturing limit of the wall
thickness will have minimal effect. For working fluids that require
smaller porosities, the wall thickness becomes a more significant
constraint, particularly in light of the fact that the only way to
currently achieve extremely small porosities is with sintered
powder, which produces a structure with a very low porosity. Given
high performance manufacturing capability the present invention
should be able to have high performance across the full range of
working fluids. As the manufacturing quality (minimum feature size)
reduces, increasing your wall size, the working fluids that will
generate the highest performance will require larger
porosities.
[0009] Patents on this general subject suggest the same general
idea: using several mesh sizes along the length of a heat pipe to
roughly grade the porosity axially. Alternately, different
grain-sizes of powder may be layered to create a sintered wick with
regions of differently-sized pores.
[0010] The similarity between the identified existing approaches is
the limitation of conventional manufacturing. It is tremendously
difficult to vary the properties of a wick spatially. The present
invention includes a new method that lends itself to a variety of
fabrication methods including additive manufacturing that would
enable 3-axis variability and the local optimization of pore size,
porosity, tortuosity, convective acceleration, structural rigidity,
and robustness to vibration and impulse.
[0011] According to one aspect of the invention, a heat pipe
includes a heat pipe wick having a gyroid or other periodic lattice
structure, thereby providing relatively high porosity, high surface
area, consistent pore size, structural stability, low tortuosity,
and low convective acceleration.
[0012] Optionally, the heat pipe wick includes a finite-order
fractal cross-section, thereby providing relatively high surface
area and porosity for a given channel size.
[0013] Optionally, the heat pipe wick comprises variable pore
sizes.
[0014] Optionally, the variable pore sizes are produced via scaling
a basic geometry of the heat pipe wick.
[0015] Optionally, the variable pore sizes are produced via skewing
a basic geometry of the heat pipe wick.
[0016] Optionally, the variable pore sizes are produced via
implementing a basic geometry of the heat pipe wick on multiple
scales in the same bulk structure.
[0017] Optionally, the heat pipe is integrated into a hypersonic
leading edge.
[0018] Optionally, the heat pipe is a loop heat pipe.
[0019] Optionally, the heat pipe is integrated into a vapor chamber
heat spreader.
[0020] Optionally, the heat pipe wick includes local fractal or
high surface roughness to increase capillary force, and wherein the
local fractal or high surface roughness is configured to reduce
overall pressure drop, thereby greatly improving heat capacity.
[0021] The foregoing and other features of the invention are
hereinafter described in greater detail with reference to the
accompanying drawings.
BRIEF DESCRIPTION OF THE DRAWINGS
[0022] FIG. 1 shows exemplary gyroid geometry with solid walls.
[0023] FIG. 2 shows exemplary stretched gyroid geometry enabling
continuously-variable porosity capabilities.
[0024] FIG. 3 shows exemplary cross sections for an 8th-order
Quadratic Flake and a 5th-order Quadratic Cross (top), and Koch
Snowflakes of order zero to 4. Each illustrates structured geometry
with a large perimeter relative to the enclosed area.
[0025] FIG. 4 shows porosity hierarchy with partially sintered
porous powder, unstructured porosity, controllable gradient
porosity, structured porosity, tunable structured porosity and then
macro micro porosity.
[0026] FIG. 5 shows other exemplary periodic high-surface-area
lattices derived from zero potential surfaces or minimal
surfaces.
[0027] FIG. 6 shows an exemplary leading edge heat pipe with
additively manufactured variable wick structure using truss or
directly printed variable porous wick.
DETAILED DESCRIPTION
[0028] A 3-axis variable-porosity-wick heat pipe can be leveraged
to improve the effective capillary pumping pressure and minimize
hydraulic losses. By using self-supporting structures such as the
gyroid, truss structures, or other structured topology, a structure
with continuously and arbitrarily varying porosity and pore size
throughout a volume can be generated. This enables total control of
the overall porosity resulting in improved peak heat transfer and
more robust heat pipes. Previous work has identified methods to
enable radial or axial variations in the porosity of the wick
structure through variable powder sizing in a sintered wick
process. These previous processes are based on powder size
variation and limit the directions and degree to which a gradient
can be produced as well as the accuracy with which an arbitrary
gradient can be represented.
[0029] The goal is to maximize heat transfer in a two-phase heat
transfer system such as a heat pipe or loop heat pipe. In order to
do so, we can increase the capillary pressure with small pore
sizes, increase the bulk porosity of the wicking structure,
increase the wetted surface area of the wick structure, reduce the
tortuosity of the flow paths, reduce the convective acceleration of
the flow paths, use gradients in pore sizes where large or smaller
flow paths are locally preferred, and construct the wick with
multi-scale flow channels. The heat transfer capability of a heat
pipe is directly related to the capillary pressure rise available
and the pressure losses within the system. Small pore sizes
increases capillary pressure, but also increases viscous pressure
losses. Increasing the bulk porosity enables more efficient
cross-sectional fluid flow. Increasing the wetted surface area
increases the capillary pressure produced. Reducing the tortuosity
of the flow paths reduces pressure losses. Reducing the convective
acceleration of the flow paths reduces pressure losses. Graded pore
sizes allow the creation of high capillary pressure where needed
without incurring small-channel pressure losses everywhere.
Multi-scale flow channels enable low-flow-rate high-pressure
channels in parallel with high-flow-rate low-pressure channels. The
consideration and improvement of each of these features will
increase the heat transport capacity, temperature range,
robustness, and reliability of a two-phase heat transfer
system.
[0030] Sintered powder wicks have excellent pore size but poor
porosity, tortuosity, convective acceleration, structural rigidity,
and robustness to vibration and impulse. Mesh screen wicks have
poor pore size, tortuosity, and convective acceleration, but have
excellent structural rigidity and robustness.
[0031] A critical capability of the present invention is the
ability to continuously and widely vary the porosity of a coherent,
structured topology throughout a volume. The purest expression of
this idea is the use of a mesostructure, such as the gyroid unit
cell shown in FIG. 1, to directly define the geometry of the wick.
A given section can then be deformed arbitrarily, shown in FIG. 2,
to produce variations in porosity along any dimension.
[0032] The gyroid is one example of the proposed mesostructural
geometry concept. The gyroid provides several benefits compared to
other structures. For the purposes of wicking, it provides a
favorable ratio of wetted perimeter to void fraction while also
allowing fluid to progress in any Cartesian direction through the
lattice due to its interconnected channels. The channels in the
gyroid provide simple paths with low tortuosity and low convective
acceleration. For the purposes of manufacture, the gyroid has very
limited overhanging areas, is fully connected, and an absence of
sharp features. This makes the geometry favorable for additive
manufacturing--particularly at a small scale. It also provides
excellent load paths for stress relieving in all directions and
retains structural rigidity.
[0033] The microstructural geometry is an additional expression of
tunable porosity. The lattice mesostructure can itself be made of
porous material, such as being formed from sintered powder. In this
case, the degree of sintering of the powder directly affects the
characteristic diameter of the pores. This allows for independent
control of mesostructural and microstructural porosity: that is,
the meso-scale porosity resulting from the space between lattice
walls, and the micro-scale porosity within the partially-sintered
lattice walls.
[0034] By varying pore size across the geometry, improvements can
be made. In particular, small pore size gives high capillary
pressure, but high pressure drop. This would be desirable desirable
near heat source & along vapor-liquid interface locations.
Accordingly, large pore size gives low capillary pressure, but low
pressure drop, and this would be desirable elsewhere.
[0035] Additional useful geometries include finite-order fractal
topologies in two and three dimensions, such as the Koch Snowflake
and the Quadratic Flake or Cross. These are illustrated in FIG. 3
and feature large perimeters for a given enclosed area.
[0036] In summary, four main features are being described herein.
First, we have identified the gyroid and other periodic lattice
structures for use as heat pipe wicks because of their capability
to deliver high porosity, high surface area, consistent pore size,
structural stability, additive manufacturability, low tortuosity,
and low convective acceleration. As a subset of the
three-dimensional topologies, we have identified the advantages of
finite-order fractals as two-dimensional channel cross-sections
that produce high surface area and porosity for a given channel
size.
[0037] Second, we propose to use these structures to deliver
tunable and variable pore sizes by scaling, skewing, and distorting
the basic geometry. This may optionally include implementing such
geometries and channels on multiple scales in the same bulk
structure.
[0038] Third, we propose to use these variable-pore-size heat pipes
conformal to and integrated into a hypersonic leading edge in
addition to the wick's use in traditional cylindrical heat pipes,
loop heat pipes, and vapor chamber heat spreaders.
[0039] Fourth, we propose that the intentional addition of fractal
or high surface roughness locally can increase the capillary force.
By defining explicitly the locations to introduce the additional
fractal roughness the impact to overall pressure drop can be
reduced to greatly improve the heat capacity of the proposed wick
design.
[0040] Of the porosity generating methods shown in FIG. 4, only
three exist in literature: micro-scale porosity resulting from
uniformly sintered powder, meso-scale unstructured porosity
resulting from burnout or dissolution of a filler material in a
packed bed, and variable micro-scale porosity resulting from
discrete regions of differently sized powders or mesh screens
stacked together.
[0041] Novel contributions described herein include the remaining
three geometries proposed: meso-scale structured porosity resulting
from selective coalescence of material through advanced
manufacturing processes, tunable meso-scale structured porosity
that continuously grades the characteristic pore diameter of a
lattice throughout a volume, and multi-scale porosity that
leverages both tunable structured porosity on the meso-scale and
independently grades the micro-scale porosity of the lattice walls
themselves by varying the degree of coalescence used to form
them.
[0042] The novel geometric configurations for the structured
porosity described herein include gyroids and other zero-potential
surfaces or minimal surfaces as well as finite-order fractal
cross-sections. The family of zero-potential surfaces, some seen in
FIG. 5, provide benefit through smooth surfaces reducing localized
stresses and the pressure loss associated with flow through those
regions. These structures introduce a new capability that generates
the next generation of high-performance heat pipes for a variety of
applications.
[0043] The novel use of these advanced systems in the application
of a leading edge hypersonic vehicle as seen in FIG. 6 is also
contemplated. The temperature regimes associated with hypersonics
results in working fluids that lend themselves to large-pore
structures. The high-temperature concentrated heat loads in
hypersonics is an ideal application of these high-performance heat
transfer systems and this enabling technology.
[0044] Although the invention has been shown and described with
respect to a certain embodiment or embodiments, it is obvious that
equivalent alterations and modifications will occur to others
skilled in the art upon the reading and understanding of this
specification and the annexed drawings. In particular regard to the
various functions performed by the above described elements
(components, assemblies, devices, compositions, etc.), the terms
(including a reference to a "means") used to describe such elements
are intended to correspond, unless otherwise indicated, to any
element which performs the specified function of the described
element (i.e., that is functionally equivalent), even though not
structurally equivalent to the disclosed structure which performs
the function in the herein illustrated exemplary embodiment or
embodiments of the invention. In addition, while a particular
feature of the invention may have been described above with respect
to only one or more of several illustrated embodiments, such
feature may be combined with one or more other features of the
other embodiments, as may be desired and advantageous for any given
or particular application.
* * * * *