U.S. patent application number 16/788039 was filed with the patent office on 2020-05-28 for preparation of libraries of protein variants expressed in eukaryotic cells and use for selecting binding molecules.
This patent application is currently assigned to IONTAS LIMITED. The applicant listed for this patent is IONTAS LIMITED. Invention is credited to Michael Dyson, John McCafferty, Kothai Parthiban.
Application Number | 20200165597 16/788039 |
Document ID | / |
Family ID | 50980562 |
Filed Date | 2020-05-28 |



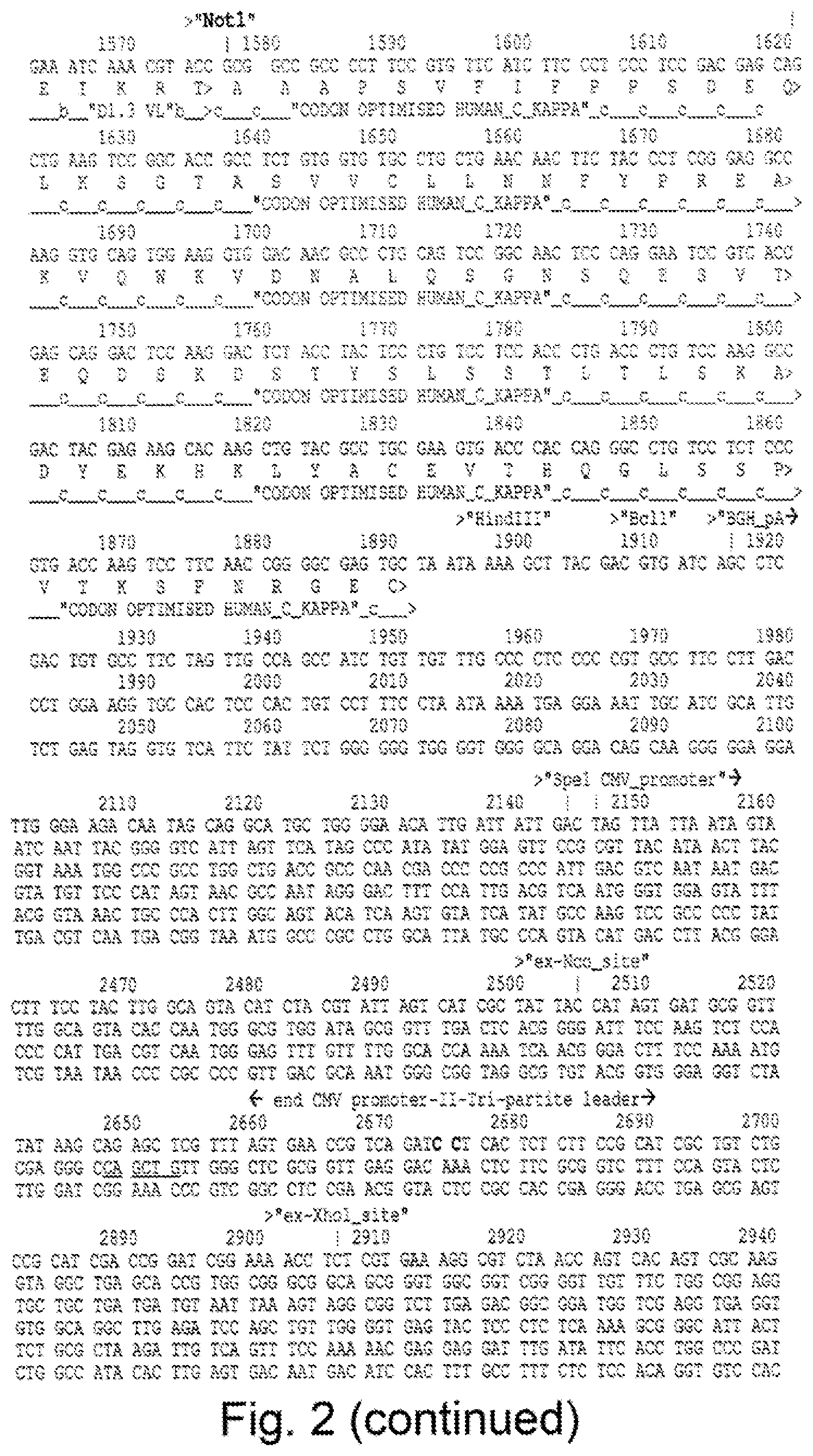


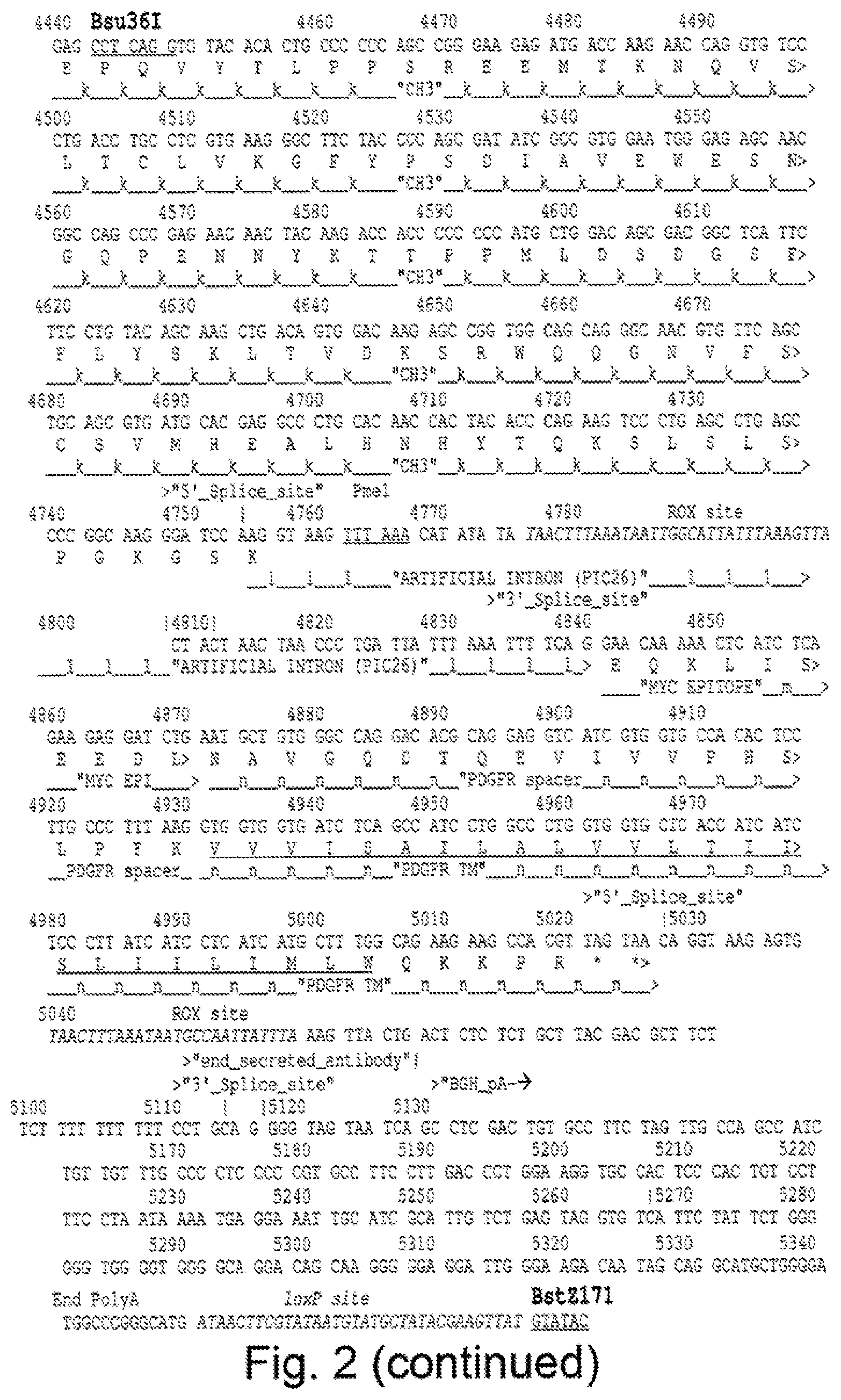
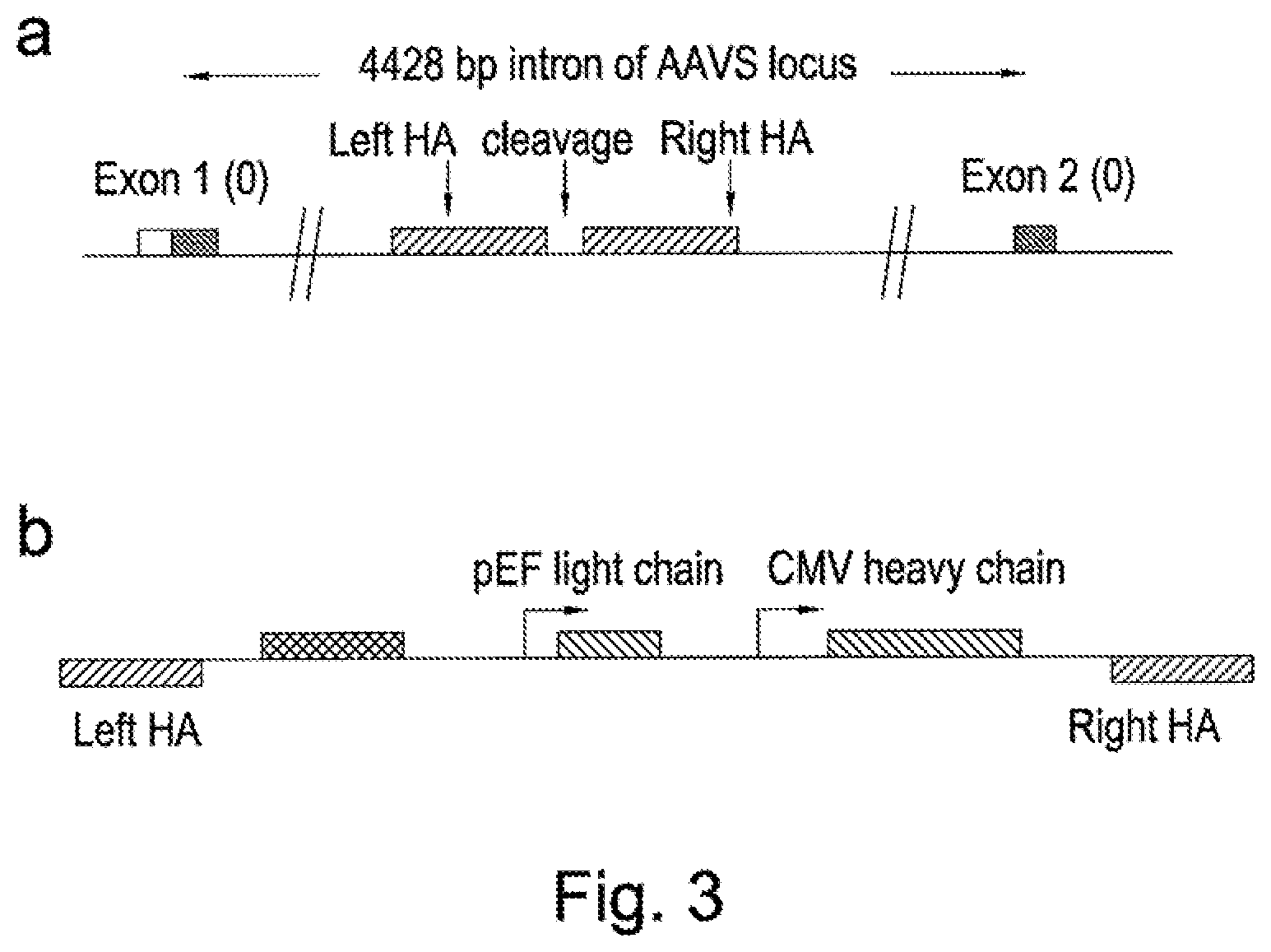

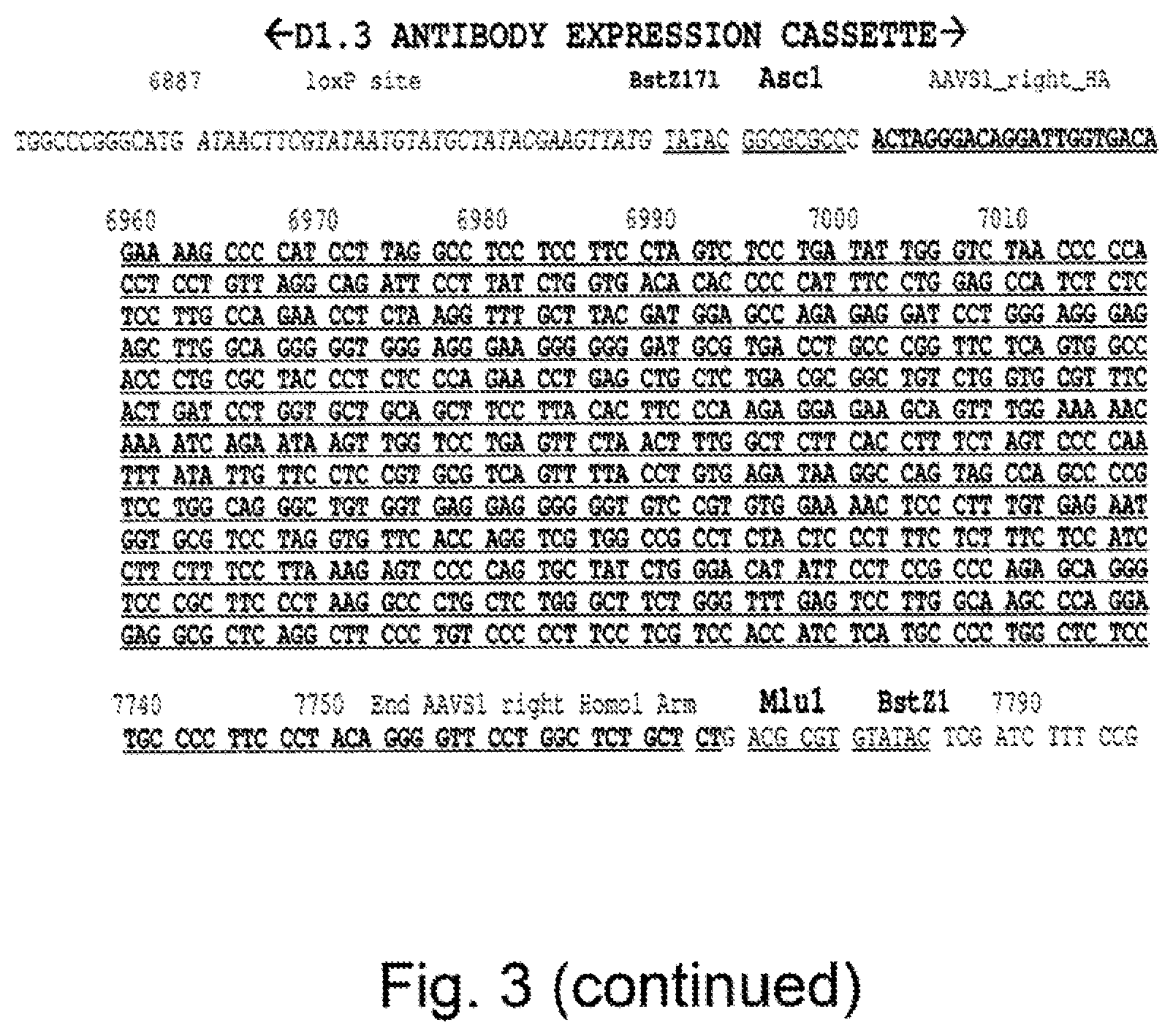
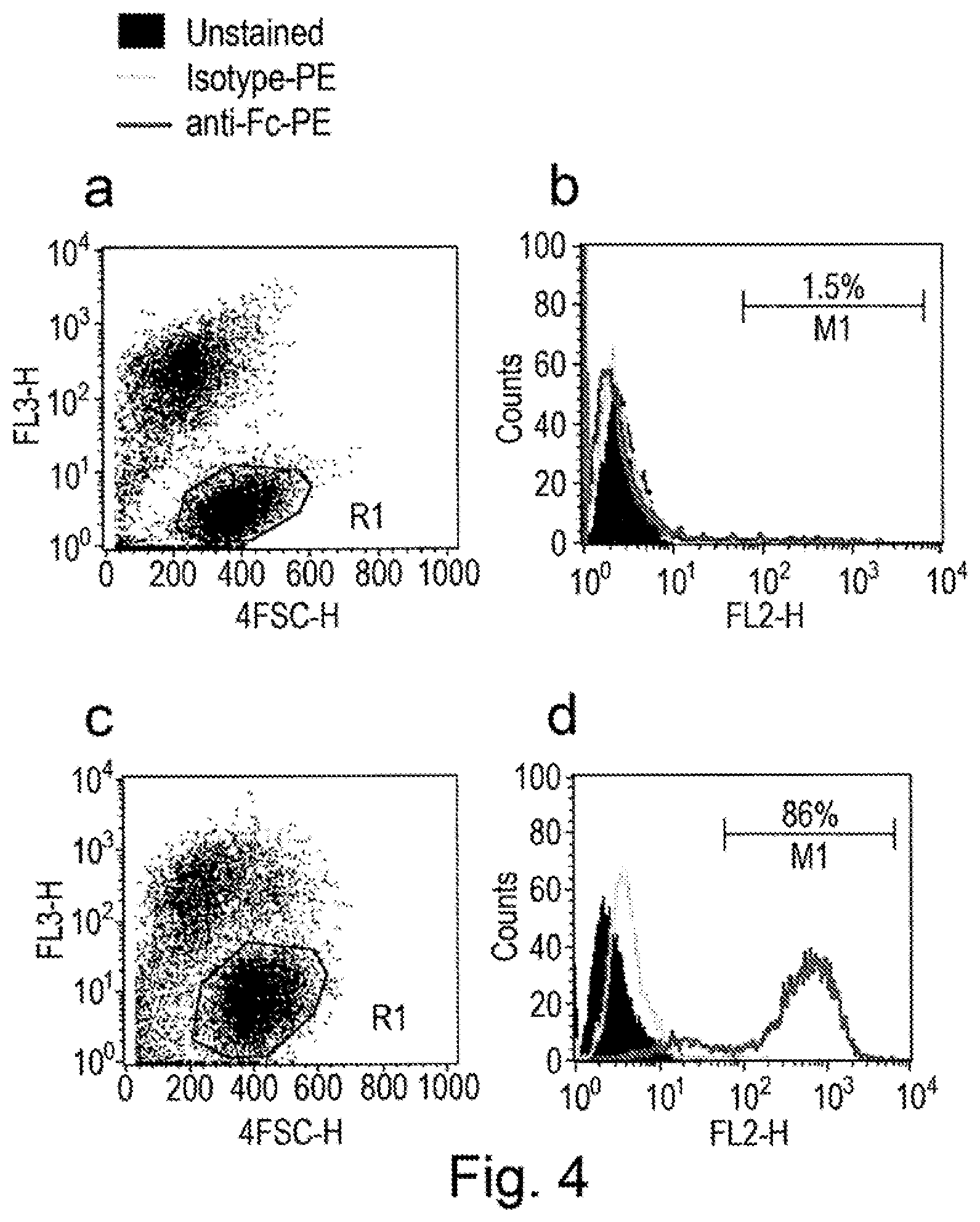
View All Diagrams
United States Patent
Application |
20200165597 |
Kind Code |
A1 |
McCafferty; John ; et
al. |
May 28, 2020 |
PREPARATION OF LIBRARIES OF PROTEIN VARIANTS EXPRESSED IN
EUKARYOTIC CELLS AND USE FOR SELECTING BINDING MOLECULES
Abstract
The invention relates to methods of producing eukaryotic cell
libraries encoding a repertoire of binding molecules ("binders"),
wherein the methods use a site-specific nuclease for targeted
cleavage of cellular DNA to enhance site-specific integration of
binder genes through endogenous cellular repair mechanisms.
Populations of eukaryotic cells are produced in which a repertoire
of genes encoding binders are integrated into a desired locus in
cellular DNA (e.g., a genomic locus) allowing expression of the
encoded binding molecule, thereby creating a population of cells
expressing different binders.
Inventors: |
McCafferty; John; (Babraham,
GB) ; Dyson; Michael; (Cambridge, GB) ;
Parthiban; Kothai; (Cambridge, GB) |
|
Applicant: |
Name |
City |
State |
Country |
Type |
IONTAS LIMITED |
Cambridge |
|
GB |
|
|
Assignee: |
IONTAS LIMITED
Cambridge
GB
|
Family ID: |
50980562 |
Appl. No.: |
16/788039 |
Filed: |
February 11, 2020 |
Related U.S. Patent Documents
|
|
|
|
|
|
Application
Number |
Filing Date |
Patent Number |
|
|
15308570 |
Nov 2, 2016 |
|
|
|
PCT/GB2015/051287 |
May 1, 2015 |
|
|
|
16788039 |
|
|
|
|
Current U.S.
Class: |
1/1 |
Current CPC
Class: |
C12N 15/907 20130101;
C07K 2317/622 20130101; C07K 2317/64 20130101; C07K 2319/036
20130101; C07K 2319/035 20130101; C12N 15/1037 20130101; C07K
16/005 20130101; C07K 2319/03 20130101; C12N 15/102 20130101; C12N
2800/80 20130101; C12N 2800/30 20130101; C12N 2310/20 20170501;
C07K 2317/24 20130101 |
International
Class: |
C12N 15/10 20060101
C12N015/10; C07K 16/00 20060101 C07K016/00; C12N 15/90 20060101
C12N015/90 |
Foreign Application Data
Date |
Code |
Application Number |
May 2, 2014 |
GB |
1407852.1 |
Claims
1. A method of integrating donor DNA into genomic DNA of a higher
eukaryotic cell a having a genome size of greater than
2.times.10.sup.7 base pairs, comprising providing single-stranded
donor DNA, wherein the donor DNA is packaged in an adeno-associated
viral (AAV) vector and comprises a large transgene encoding a
binder, wherein the binder is an antibody molecule, a receptor or a
protein, introducing the donor DNA into a cell, and cleaving the
genomic DNA with a site-specific nuclease, whereby the transgene is
incorporated into the genomic DNA.
2. The method of claim 1, wherein the binder is an engineered
protein, a T cell receptor (TCR) or a chimeric antigen receptor
(CAR).
3. The method of claim 1, wherein the binder is a full length
immunoglobulin, IgG, Fab, scFv-Fc or scFv.
4. The method of claim 1, wherein the site-specific nuclease is a
meganuclease, a zinc finger nuclease, a TALE nuclease or a nucleic
acid-guided nuclease.
5. The method of claim 4, wherein the site-specific nuclease is a
CRISPR/Cas nuclease.
6. The method of claim 5, wherein the site-specific nuclease is
introduced as a protein:RNA complex.
7. The method of claim 1, wherein the cell is a mammalian cell.
8. The method of claim 7, wherein the cell is a HEK293 cell, a
Chinese hamster ovary cell, a T lymphocyte lineage cell or a B
lymphocyte lineage cell.
9. The method of claim 8, wherein the cell is a primary T cell or a
T cell line.
10. The method of claim 8, wherein the cell is a primary B cell, a
B cell line, a pre-B cell line or a pro-B cell line.
11. The method of claim 1, the method further comprising culturing
the eukaryotic cell comprising the transgene incorporated into its
genomic DNA under conditions for expression of the binder, and
isolating the binder.
12. A method of producing a library of eukaryotic cell clones
containing DNA encoding a diverse repertoire of binders, wherein
the binders are antibody molecules, receptors or proteins,
comprising providing single-stranded donor DNA molecules encoding
the binders, wherein the donor DNA molecules are packaged in AAV
vectors, providing higher eukaryotic cells with a genome size of
greater than 2.times.10.sup.7 base pairs, introducing the donor DNA
into the cells and providing a site-specific nuclease within the
cells, wherein the nuclease cleaves a recognition sequence in
cellular DNA to create an integration site at which the donor DNA
becomes integrated into the cellular DNA, integration occurring
through DNA repair mechanisms endogenous to the cells, thereby
creating recombinant cells containing donor DNA integrated in the
cellular DNA, and culturing the recombinant cells to produce
clones, thereby providing a library of eukaryotic cell clones
containing donor DNA encoding the repertoire of binders.
13. The method of claim 12, wherein the binders are full length
immunoglobulins, IgG, Fab, scFv-Fc or scFv.
14. The method of claim 12, wherein the binders are T cell
receptors or chimeric antigen receptors (CARs).
15. A method of screening for a binder that recognises a target,
comprising: producing a library by the method of claim 12,
culturing cells of the library to express the binders, exposing the
binders to the target, allowing recognition of the target by one or
more cognate binders, if present, and detecting whether the target
is recognised by a cognate binder.
16. The method of claim 15, further comprising detecting target
recognition by a cognate binder, and recovering cells of a clone
containing DNA encoding the cognate binder.
17. The method of claim 16, further comprising isolating nucleic
acid encoding the binder from the recovered clone, thereby
obtaining nucleic acid encoding a binder that recognises the
target.
18. The method of claim 17, comprising optionally introducing
mutation or converting the nucleic acid to modified nucleic acid
encoding a restructured binder, and introducing DNA encoding the
binder into a host cell.
19. The method of claim 18, further comprising culturing the cells
to express the binder, and purifying the binder.
Description
CROSS-REFERENCE TO RELATED APPLICATIONS
[0001] This application is a continuation of U.S. patent
application Ser. No. 15/308,570, filed Nov. 2, 2016, which is a 35
U.S.C. .sctn. 371 application of International Application Serial
No. PCT/GB2015/051287, filed May 1, 2015; which claims the benefit
of Great Britain Application Number 1407852.1 that was filed on May
2, 2014. The entire content of the applications referenced above
are hereby incorporated by reference herein.
SEQUENCE LISTING
[0002] The instant application contains a Sequence Listing which
has been submitted in ASCII format via EFS-Web and is hereby
incorporated by reference in its entirety. Said ASCII copy, created
on Oct. 31, 2016, is named 00130_003US1_SL.txt and is 92.9
kilobytes in size.
FIELD OF THE INVENTION
[0003] This invention relates to methods of producing eukaryotic
(e.g., mammalian) cell libraries for screening and/or selection of
binding molecules such as antibodies. Libraries can be used to
contain and display a diverse repertoire of binders, allowing
binders to be screened to select one or more binders having a
desired property such as specificity for a target molecule. The
invention especially relates to methods of introducing donor DNA
encoding the binders into eukaryotic cells to provide a cell
library in which a desired number of donor DNA molecules are
faithfully integrated at a desired locus or loci in the cells.
INTRODUCTION
[0004] Protein engineering techniques permit creation of large
diverse populations of related molecules (e.g., antibodies,
proteins, peptides) from which individual variants with novel or
improved binding or catalytic properties can be isolated. The
ability to construct large populations of eukaryotic cells,
particularly mammalian cells, where each cell expresses an
individual antibody, peptide or engineered protein would have great
value in identifying binders with desired properties.
[0005] The basic principle of display technology relies on the
linkage of a binding molecule to the genetic information encoding
that molecule. The binding properties of the binding molecule are
used to isolate the gene which encodes it. This same underlying
principles applies to all forms of display technology including,
bacteriophage display, bacterial display, retroviral display,
baculoviral display, ribosome display, yeast display and display on
higher eukaryotes such as mammalian cells [1, 2, 3, 4].
[0006] Display technology has best been exemplified by display of
antibodies on filamentous bacteriophage (antibody phage display)
which over the last 24 years has provided important tools for
discovery and engineering of novel binding molecules including the
generation of human therapeutic antibodies. Using phage display
antibody molecules are presented on the surface of filamentous
bacteriophage particles by cloning the gene encoding an antibody or
antibody fragment in-frame with the gene encoding a phage coat
protein. The antibody genes are initially cloned into E. coli such
that each bacterium encodes a single antibody. Generation of
bacteriophage from the bacteria using standard methods results in
the generation of bacteriophage particles displaying an antibody
fragment on their surface and encapsulating the encoding antibody
gene within the bacteriophage. The collection of bacteria or the
bacteriophage derived from them is referred to as an "antibody
library". Using antibody phage display, antibodies and their
associated genes can be enriched within the population by exposing
antibody-presenting bacteriophage to a target molecule of
interest.
[0007] To allow recovery of bacteriophage displaying a binder
recognising a target of interest, the target molecule needs to be
immobilised onto the surface of a selection vessel or needs to be
recoverable from solution by secondary reagents, e.g., biotinylated
target protein, recovered from solution using streptavidin-coated
beads. Following incubation of the library of binder-displaying
bacteriophage with the target molecule, unbound phage are removed.
This involves washing the matrix to which the target (and
associated bacteriophage) is attached to remove unbound
bacteriophage. Bound bacteriophage with their associated antibody
gene can be recovered and/or infected into host bacterial cells.
Using the approach outlined above it becomes possible to enrich a
subset of bacteriophage clones capable of binding a target molecule
of choice. Phage display libraries have been shown to provide a
rich source of antibody diversity, providing hundreds of unique
antibodies to a single target [5,6,7].
[0008] Historically, display systems for isolating novel antibody
binding specificities have been based in prokaryotic systems and in
particular on display of single chain Fvs (scFv) and to a lesser
extent as Fabs on bacteriophage. Display of binders on the surface
of bacteria has been described but has not been widely used and
applications have largely been limited to peptide display or
display of antibody fragments pre-enriched for binders through
immunisation [8]. Despite the power of prokaryotic display systems
including phage display there are limitations. Following selection
by phage or ribosome display the genes encoding individual binding
molecules are identified by introducing the selected gene
population into bacteria, plating the bacterial populations,
picking colonies, expressing binding molecules into the supernatant
or periplasm and identifying positive clones in binding assays such
as enzyme or fluorescence linked immunosorbent assays (ELISA).
Although binding molecules are identified this approach does not
resolved information on the extent of expression and the binding
affinity of the resultant clones. Thus although it is possible to
generate potentially thousands of binders, the ability to screen
the output is limited by the need for colony picking, liquid
handling etc., coupled with limited primary information on relative
expression level and affinity.
[0009] Display of binding molecules on the surface of eukaryotic
cells has the potential to overcome some of these problems. In
conjunction with flow cytometry, eukaryotic display allows rapid,
high throughput selection. It becomes possible to survey millions
of cellular clones expressing different binding molecules on their
surface. Cell surface display has best been exemplified for the
display of antibody fragments formatted as scFvs on the surface of
yeast cells. A commonly used modality for yeast surface display
makes use of the yeast agglutinin proteins (Aga1p and Aga2p). As
described by Chao et al. [9], genes encoding a repertoire of scFvs
are genetically fused with the yeast agglutinin Aga2p subunit. The
Aga2p subunit then attaches to the Aga1p subunit present in the
cell wall via disulphide bonds. Yeast cells expressing a
target-specific binding molecule can be identified by flow
cytometry using directly or indirectly labelled target molecule.
For example biotinylated target can be added to cells and binding
to the cell surface can be detected with
streptavidin-phycoerythrin. Within a population it becomes
possible, using limiting target concentrations, to distinguish
those clones which express higher affinity binding molecules since
these clones will capture more target molecules and will therefore
exhibit brighter fluorescence. Typically, each yeast cell will
display 10,000 to 100,000 copies of a single scFv on the surface of
the cell. To control for variation in scFv surface expression in
different cells Chao et al used a fluorescently labelled anti-tag
antibody to measure antibody expression level on the surface of
each cell allowing normalisation for variation in expression level.
This approach therefore allows yeast cells displaying high affinity
binding molecules to be differentiated from those cells expressing
high levels of a lower affinity antibody. Thus using fluorescence
activated cell sorting (FACS) it is possible to separate cell
clones according to the affinity and/or expression level of the
encoded binding molecule.
[0010] Eukaryotic systems have also proven to be more effective
than prokaryotic systems for the display of multi-chain antibody
fragments and in particular with larger fragments such as full
IgGs, FAbs or fusions of scFv with Fc domains (scFv-Fc fusions).
Bead-based or flow sorting-based methods as described above for
yeast cells could also be used to select antibodies from display
libraries based on higher eukaryotes such as mammalian cells. The
ability to format display libraries and select directly as IgGs,
Fabs or as scFv-Fc fusions in mammalian cells would be a further
advantage over yeast display. The glycosylation, expression and
secretion machinery of bacterial and yeast cells is different from
higher eukaryotes giving rise to antibodies with different
post-translational modifications than those produced in mammalian
cells. Since the manufacture of antibodies for research, diagnostic
and therapeutic application is typically carried out in mammalian
cells, display on mammalian cells (or other higher eukaryotic cells
such as invertebrate, avian or plant cell lines) could give a
better indication of potential issues or benefits for downstream
manufacturing, e.g., identifying clones with optimal expression
properties. In addition, antibodies discovered within the context
of display on higher eukaryotes and particularly mammalian cells
could be applied directly into cell-based reporter assays without
extensive purification and without the complicating effect of
contaminants from bacteria and yeast cells. Further, libraries of
binders could be expressed directly in eukaryotic reporter cells
such as mammalian cells to identify clones which directly affect
cellular phenotype.
[0011] Despite the above advantages promised by eukaryotic display
libraries, there remain significant problems with creation of
libraries of binders in eukaryotic cells, especially higher
eukaryotic cells. Introduction of a repertoire of exogenous genes
("transgenes") for expression in higher eukaryotes is more
difficult than in yeast and bacteria. The cells of higher
eukaryotes are more difficult to handle and scale up and
transformation efficiencies are lower. Typical library sizes
achieved are much smaller. In addition, introduced DNA integrates
randomly within the genome leading to position effect variegation.
Further, donor DNA introduced into mammalian cells by standard
transfection or electroporation methods integrates as a linear
array with variable copy number of the transfected transgene. The
introduction of DNA encoding a repertoire of antibody genes
therefore has the potential to introduce multiple antibody genes
into each cell resulting in expression of multiple distinct
antibodies per cell. In addition the presence of multiple antibody
genes will reduce the relative expression of any given antibody and
will lead to the isolation of many passenger antibody genes
reducing the rate of enrichment of specific clones.
[0012] Although display of a library of binders on the surface of
higher eukaryotes is more challenging, some examples have
previously been described. In an early publication using mammalian
display of IgGs derived from human immunisation, 3 rounds of
selection (involving transient transfection, cell sorting, DNA
recovery and re-transfection) were required to achieve a 450 fold
enrichment of antigen-specific cells, averaging 7.6 fold enrichment
per round [10]. Similarly transient expression from immunised
libraries expressed within episomally-replicating vectors has also
been described with antibodies formatted as scFvs [11, 12] or IgGs
[13].
[0013] A number of approaches have been described to introduce a
single or limited number of antibody genes into each cell. This
includes dilution of DNA or mixing with carrier DNA [13] but this
is a relatively uncontrolled method for managing copy number of
introduced genes and reducing DNA input will have a detrimental
effect on library size. Introduction of antibody genes by viral
vectors has provided another solution to control the introduction
of multiple antibody genes per cell. A cell surface display library
has been generated in this way from several hundred human B
lymphocytes generated by immunization and further enriched by flow
sorting of antigen-specific B cells [14]. The antibody genes from
this enriched pool were formatted as scFvs, cloned into a Sindbis
alphavirus expression system and introduced into BHK cells using a
low multiplicity of infection.
[0014] Breous-Nystrom et al. [15] used sequential retroviral
infection to introduce a limited repertoire of 91 V kappa antibody
genes followed by a heavy chain genes repertoire from 6 healthy
donors into a murine pre-B cell line (1624-5). Infectious
retrovirus was generated using the V-Pack system based on Moloney
Murine Leukemia Virus (Stratagene). In order to bias towards single
copy insertions, a multiplicity of infection was chose which led to
infection of approximately 5% of cells. A major disadvantage of
these approaches is that integration within the genome is random,
leading to potential variation in transcription level based on the
transcriptional activity of the site of integration. Another
disadvantage in all these cases is that the integration of the
antibody genes is controlled by limited infection or transfection
which impacts on library size.
[0015] Site-specific integration of transgenes directed by
recombinases has previously been described. Recombinases are
enzymes that catalyse exchange reactions between DNA molecules
containing enzyme-specific recognition sequences. For example Cre
recombinase (derived from the site specific recombination system of
E. coli) or Flp recombinase (utilising a recombination system of
Saccharomyces cerevisiae) act on their specific 34 bp loxP
recognition sites and 34 bp Flp Recombination Target (FRT) site
respectively [16]. Recombinases have mainly been used in cellular
engineering to catalyse site-specific integration. A number of
studies from the work of Chen Zhou [17, 18, U.S. Pat. No.
7,884,054] have described the recombinase-mediated site-specific
integration of antibody genes into the genome of mammalian cells
using Flp recombinase within the "Flp-In" system,
(http://tools.lifetechnologies.com/content/sfs/manuals/flpinsystem_man.pd-
f). The Flp-In system utilises a variety of cell lines which have
previously had a single FRT site introduced within their genome. By
expressing the enzyme Flp recombinase it is possible to direct
integration of expression plasmids, incorporating a FRT
recombination site, into this pre-integrated FRT site in target
cells.
[0016] Using the Flp-In system Zhou et al. [17] introduced an
incoming antibody expression plasmid containing a FRT site into
Chinese Hamster Ovary (CHO) cell line incorporating a FRT site
(CHOF cells). Their work describes construction of a display
library where 4 residues within an existing anti-OX40 ligand
antibody were mutagenised. The library was screened using FACS to
identify antibodies with anti-ligand affinity on the cell surface.
The overall success in generating improved antibodies was limited
to the isolation of a single improved antibody. The number of
unique mammalian cell clones achieved was not reported.
[0017] A follow-on paper by Li et al. in 2012 [18] utilised
lymphocytes from a hepatitis B patient to construct an antibody
display library. Separate libraries were produced with the heavy
and light chain genes obtained from a donor who had been immunised
with HBsAg, individually reported to be libraries of size
1.02.times.10.sup.6 and 1.78.times.10.sup.5, respectively. A
secondary library was then produced including both the heavy and
light chains which reportedly had a size of 4.32.times.10.sup.5.
FACS analysis reportedly indicated that about 40% of the cells
displayed detectable full-length antibodies on the cell surface.
FACS screening of the library identified antibodies binding to
HBsAg. Of a sample of 8 selected library members which bound to the
antigen, six were found to have the same antibody, so in total
three unique anti-HBsAg clones were identified.
[0018] The rather limited success of this work may be due to the
fact that the Flp-In system is designed for accurate integration in
a limited number of clones rather than large library construction.
There is therefore a potential conflict between achieving fidelity
of integration versus achieving maximal library size. The Flp-In
system utilises a mutant Flp recombinase in the plasmid pOG44 which
possesses only 10% of the activity at 37.degree. C. of the native
Flp recombinase [19]. A variant of Flp recombinase (Flpe) with
better thermostability and higher activity than wild type has been
identified [19, 20]. This was further improved by codon
optimization to create Flp.sub.o encoded within plasmid
cCAGGS-Flp.sub.o (Genebridges Cat. A203) According to the Flp-In
manual however: [0019] "When generating Flp-In.TM. expression cell
lines, it is important to remember that you are selecting for a
relatively rare recombination event since you want recombination
and integration of your pcDNA.TM.5/FRT construct to occur only
through the FRT site and for a limited time. In this case, using a
highly inefficient Flp recombinase is beneficial and may decrease
the occurrence of other undesirable recombination events . . . .
[0020] . . . To increase the likelihood of obtaining single
integrants, you will need to lower the transfection efficiency by
limiting the amount of plasmid DNA that you transfect" This is
echoed by Buchholz et al., 1996 [19]: [0021] "FLP may be
particularly useful for applications that do not rely on efficiency
but depend on tight regulation".
[0022] In model experiments and using "instructions described in
the manual", Zhou et al. (2010) [17] indeed demonstrated that
single copy insertions occurred in >90% of clones. In library
construction however relatively high amounts of expression plasmid
(2.5-3.2 .mu.g per 10.sup.6 cells) and a donor excess over pOG44
recombinase-encoding plasmid was used [17, 18]. The Flp-In system
recommends using a ratio of at least 9:1 in favour of the
recombinase encoding plasmid versus the expression plasmid.
However, when seeking to increase library size by transfecting
larger amounts of DNA there is the potential for random integration
of the incoming plasmid [21]. In all studies the accuracy of
integration and the number of integrants per cell under "library
construction" conditions was not reported.
[0023] In nuclease-directed integration of genes a site-specific
nuclease is used to cleave cellular DNA at a specific location. It
has previously been shown that this enhances the rate of homologous
recombination by at least 40,000 fold and also allows repair by
non-homologous end-joining mechanisms. This enhancement of
site-specific integration has not previously been used or
contemplated to solve the problems associated with creating
libraries of binders.
[0024] US20100212035 describes methods for generation of rodents
capable of expressing exogenous antibody by targeting the
immunoglobulin locus of a mammalian embryo with a meganuclease to
direct integration of a donor DNA. The potential to create variant
libraries of meganucleases to create new DNA cleavage specificities
is described but it his does not contemplate the use of
meganucleases towards the generation of libraries of binders.
[0025] WO 2013/190032 A1 describes integration of genes into a
specific locus (FerlL4) previously modified with exogenous DNA ("a
site specific integration" SSI host cell) to incorporate
recombinase sites, such as loxP and FRT sites for
recombinase-mediated site-specific gene introduction.
Nuclease-directed library generation is not described.
[0026] WO 2012/167192 A2 describes targetting genes to a locus that
can then be selected for amplification. Nuclease-directed methods
are employed to target the locus. Nuclease-directed library
generation is not described.
[0027] US 2009/0263900A1 describes DNA molecules comprising
homology arms and their use in methods of homologous recombination.
Nuclease-directed library generation is not described.
[0028] WO 2011/100058 describes methods for integration of nucleic
acid into a genome that avoids the need for long homology arms and
instead relies on microhomology or "sticky ends" on the genome and
donor to help direct integration. Nuclease-directed library
generation is not described.
[0029] WO 2011/090804 describes methods for integration of multiple
genes or multiple copies of the same gene using different zinc
finger nucleases (ZFNs) in sequential rounds. Nuclease-directed
library generation is not described.
[0030] WO2014/039872 describes methods for engineering plant cells,
incorporating a "landing site" into which donor DNA is integrated
by homologous recombination or non-homologous end joining using
site-directed nucleases. Bacterial artificial chromosome (BAC)
libraries are used for initial cloning of donor DNA. Libraries are
mentioned in relation to Illumina sequencing methods.
Nuclease-directed library generation is not described.
[0031] WO2007/047859 A2 describes methods for engineering
specificity of meganucleases and their used to target genomic loci.
Libraries of mutant meganucleases that may contain meganucleases
with new nuclease specificity are described. Nuclease-directed
library generation is not described.
[0032] US2014/0113375 A1 describes a transient expression system
for generation single-stranded DNA sequences homologous to a target
genomic sequence, which can be transported to the nucleus to alter
the genetic information of the target genomic sequence via DNA
repair pathways or homologous recombination. It is suggested that a
"library" of mutations could be created by low fidelity reverse
transcription of the introduced (non-library) DNA. Mammalian
display and selection of molecules with binding activity is not
described.
[0033] US2012/0277120 describes methods and compositions for the
simultaneous integration of a plurality of exogenous nucleic acids
is in a single transformation reaction using the native homologous
recombination machinery in yeast, which recombination may be
further enhanced by inducing targeted double-strand breaks in the
host cell's genome at the intended sites of integration. The
methods are intended to overcome the need for multiple rounds of
engineering to integrate multiple DNA assemblies, for example, for
the construction of functional metabolic pathways in industrial
microbes, such as yeast. The display or expression of libraries of
binding molecules, the use of higher eukaryotes and the selection
of molecules with binding activity is not described.
[0034] To fully realize the potential for antibody display on
mammalian cells and other higher eukaryotes there is a need for a
system to create large libraries which combine accurate integration
into a pre-defined site with an efficiency that allows construction
of large libraries.
SUMMARY OF THE INVENTION
[0035] We have overcome the problem of creating large libraries of
binders encompassing one or two binder genes per cell by using
nuclease-directed integration of populations of genes encoding
binders. The invention thus allows preparation of populations of
eukaryotic cells wherein a repertoire of binder-encoding is
integrated into a fixed locus in the genome allowing expression of
the encoded binding molecule, thereby creating a population of
cells expressing different binders.
[0036] The present invention relates to methods of producing
eukaryotic cell libraries encoding a repertoire of binding
molecules ("binders"), wherein the methods use a site-specific
nuclease for targeted cleavage of cellular DNA to enhance
site-specific integration of binder genes through endogenous
cellular repair mechanisms. Site-specific nucleases permit the
accurate introduction of donor DNA encoding binder molecules into
one or more defined loci within the eukaryotic genome or other
eukaryotic cell DNA. The invention provides methods of preparing
populations of eukaryotic cells in which a repertoire of genes
encoding binders are integrated into a desired locus in cellular
DNA (e.g., a genomic locus) allowing expression of the encoded
binding molecule, thereby creating a population of cells expressing
different binders.
[0037] Construction of libraries of binders within eukaryotic cells
according to the present invention has advantages over
recombinase-directed approaches for site-directed incorporation of
expression constructs. The present invention uses cellular DNA
cleavage by site-specific nucleases to solve problems previously
associated with construction of large repertoires of binder genes
in eukaryotic cells and particularly higher eukaryotes. This
invention allows the efficient creation of large populations of
cell clones each expressing individual binders integrated at a
fixed locus in cellular DNA. From these libraries of cellular
clones it becomes possible to isolate genes encoding novel binding
or function-modifying proteins and peptides.
[0038] Rather than recombinase-directed exchange of DNA, the
approach of the present invention utilises site-specific cleavage
of cellular (e.g., genomic) DNA followed by the use of natural
repair mechanisms to integrate binder-encoding donor DNA. Following
cleavage of the cellular DNA at a sequence recognised by the
site-specific nuclease ("recognition sequence"), breaks in the
cellular DNA are repaired using mechanisms such as homologous
recombination or non-homologous end joining (NHEJ). Creation of
site-specific breaks in the cellular DNA enhances incorporation of
exogenous donor DNA allowing the construction of large populations
of cells with binder genes integrated at a fixed locus.
[0039] To date, site-specific nucleases such as meganucleases,
ZFNs, TALE nucleases and CRISPR/Cas systems have been directed
towards the efficient creation of cells with modifications to
endogenous genes or for introduction of reporter genes for the
study of cell function. There are also instances where
nuclease-directed genomic targeting has been used to integrate
genes encoding single secreted antibodies for antibody production
(by purification from culture medium) [21, 22,].
[0040] The invention simplifies construction of large libraries
while directing integration to a single or limited number of
defined genetic loci. Integration of donor DNA at one or more fixed
loci normalises transcription compared with random integration of
variable numbers of transgenes, and allows selection of antibody
clones on the basis of translational and stability properties of
the binder itself. Faithful integration of donor DNA at a
pre-determined location or locations in the cellular DNA results in
relatively uniform levels of transcription of binders in the
library, and high efficiency of donor DNA introduction, make cell
populations created by the methods of the invention particularly
useful as libraries for display and selection of binders. Methods
of the invention thus produce high quality libraries of binders in
eukaryotic cells, which can be screened to identify cells encoding
and expressing a specific binder for a target of interest.
[0041] In various aspects the invention relates to new and improved
methods of preparing eukaryotic cell libraries, the libraries
themselves, isolation of desired binders, encoding nucleic acid and
cells from the libraries, and uses of the libraries such as for
expression and screening of binding molecules and for screening for
the effects of binding molecules. Various methods will be described
for producing libraries in vitro and using libraries in vitro or in
vivo.
[0042] The invention provides a method of producing a library of
eukaryotic cell clones containing DNA encoding a diverse repertoire
of binders, the method comprising using a site-specific nuclease to
target cleavage of eukaryotic cell DNA to enhance site-specific
integration of binder genes into the cellular DNA through
endogenous cellular DNA repair mechanisms.
[0043] A method of producing a library of eukaryotic cell clones
containing DNA encoding a diverse repertoire of binders may
comprise:
[0044] providing donor DNA molecules encoding the binders, and
eukaryotic cells,
[0045] introducing the donor DNA into the cells and providing a
site-specific nuclease within the cells, wherein the nuclease
cleaves cellular DNA to create an integration site at which the
donor DNA becomes integrated into the cellular DNA, integration
occurring through DNA repair mechanisms endogenous to the
cells.
[0046] For multimeric binders comprising at least a first and
second subunit (i.e., separate polypeptide chains, such as antibody
VH and VL domains presented within a Fab or IgG format), the
multiple subunits may be encoded on the same molecule of donor DNA.
However, it may be desirable to integrate the different subunits
into separate loci, in which case the subunits can be provided on
separate donor DNA molecules. These could be integrated within the
same cycle of nuclease-directed integration or they may be
integrated sequentially using nuclease-directed integration for one
or both integration steps.
[0047] Methods of producing libraries of eukaryotic cell clones
encoding multimeric binders may comprise:
[0048] providing eukaryotic cells containing DNA encoding the first
subunit, and providing donor DNA molecules encoding the second
binder subunit,
[0049] introducing the donor DNA into the cells and providing a
site-specific nuclease within the cells, wherein the nuclease
cleaves a recognition sequence in cellular DNA to create an
integration site at which the donor DNA becomes integrated into the
cellular DNA, integration occurring through DNA repair mechanisms
endogenous to the cells, thereby creating recombinant cells which
contain donor DNA integrated in the cellular DNA. These recombinant
cells will contain DNA encoding the first and second subunits of
the multimeric binder, and may be cultured to express both
subunits. Multimeric binders are obtained by expression and
assembly of the separately encoded subunits.
[0050] In the above example, nuclease-directed integration is used
to integrate DNA encoding a second subunit into cells already
containing DNA encoding a first subunit. The first subunit could be
previously introduced using the techniques of the present invention
or any other suitable DNA integration method. An alternative
approach is to use nuclease-directed integration in a first cycle
of introducing donor DNA, to integrate a first subunit, followed by
introducing the second subunit either by the same approach or any
other suitable method. If the nuclease-directed approach is used in
multiple cycles of integration, different site-specific nucleases
may optionally be used to drive nuclease-directed donor DNA
integration at different recognition sites. A method of generating
the library may comprise:
[0051] providing first donor DNA molecules encoding the first
subunit, and providing eukaryotic cells,
[0052] introducing the first donor DNA into the cells and providing
a site-specific nuclease within the cells, wherein the nuclease
cleaves a recognition sequence in cellular DNA to create an
integration site at which the donor DNA becomes integrated into the
cellular DNA, integration occurring through DNA repair mechanisms
endogenous to the cells, thereby creating a first set of
recombinant cells containing first donor DNA integrated in the
cellular DNA,
[0053] culturing the first set of recombinant cells to produce a
first set of clones containing DNA encoding the first subunit,
[0054] introducing second donor DNA molecules encoding the second
subunit into cells of the first set of clones, wherein the second
donor DNA is integrated into cellular DNA of the first set of
clones, thereby creating a second set of recombinant cells
containing first and second donor DNA integrated into the cellular
DNA, and
[0055] culturing the second set of recombinant cells to produce a
second set of clones, these clones containing DNA encoding the
first and second subunits of the multimeric binder,
[0056] thereby providing a library of eukaryotic cell clones
containing donor DNA encoding the repertoire of multimeric
binders.
[0057] Site-specific integration of donor DNA into cellular DNA
creates recombinant cells, which can be cultured to produce clones.
Individual recombinant cells into which the donor DNA has been
integrated are thus replicated to generate clonal populations of
cells--"clones"--each clone being derived from one original
recombinant cell. Thus, the method generates a number of clones
corresponding to the number of cells into which the donor DNA was
successfully integrated. The collection of clones form a library
encoding the repertoire of binders (or, at an intermediate stage
where binder subunits are integrated in separate rounds, the clones
may encode a set of binder subunits). Methods of the invention can
thus provide a library of eukaryotic cell clones containing donor
DNA encoding the repertoire of binders.
[0058] Methods of the invention can generate libraries of clones
containing donor DNA integrated at a fixed locus, or at multiple
fixed loci, in the cellular DNA. By "fixed" it is meant that the
locus is the same between cells. Cells used for creation of the
library may therefore contain a nuclease recognition sequence at a
fixed locus, representing a universal landing site in the cellular
DNA at which the donor DNA can integrate. The recognition sequence
for the site-specific nuclease may be present at one or more than
one position in the cellular DNA.
[0059] Libraries produced according to the present invention may be
employed in a variety of ways. A library may be cultured to express
the binders, thereby producing a diverse repertoire of binders. A
library may be screened for a cell of a desired phenotype, wherein
the phenotype results from expression of a binder by a cell.
Phenotype screening is possible in which library cells are cultured
to express the binders, followed by detecting whether the desired
phenotype is exhibited in clones of the library. Cellular read-outs
can be based on alteration in cell behaviour such as altered
expression of endogenous or exogenous reporter genes,
differentiation status, proliferation, survival, cell size,
metabolism or altered interactions with other cells. When the
desired phenotype is detected, cells of a clone that exhibits the
desired phenotype may then be recovered. Optionally, DNA encoding
the binder is then isolated from the recovered clone, providing DNA
encoding a binder which produces the desired phenotype when
expressed in the cell.
[0060] A key purpose for which eukaryotic cell libraries have been
used is in methods of screening for binders that recognise a target
of interest. In such methods a library is cultured to express the
binders, and the binders are exposed to the target to allow
recognition of the target by one or more cognate binders, if
present, and detecting whether the target is recognised by a
cognate binder. In such methods, binders may be displayed on the
cell surface and those clones of the library that display binders
with desired properties can be isolated. Thus cells incorporating
genes encoding binders with desired functional or binding
characteristics could be identified within the library. The genes
can be recovered and used for production of the binder or used for
further engineering to create derivative libraries of binders to
yield binders with improved properties.
[0061] The present invention offers advantages over previous
approaches for construction of libraries in higher eukaryotes. Some
studies have used lentiviral infection to introduce antibody genes
into mammalian reporter cells [106]. This has the advantage that
large libraries can be generated but there is no control over the
site of integration and copy number is controlled by using a low
multiplicity of infection (as discussed above). In an alternative
approach antibody genes were introduced via homologous
recombination, without the benefit of nuclease-directed integration
and using homology arms of 10 kb but the efficiency of targeting
was relatively low meaning that the potential library size was
limited [105]. In contrast the use of sequence-directed nucleases
retains the benefits of targeted integration to one or a few loci
of choice while allowing efficient construction of large libraries.
Nuclease-directed integration has the advantage that transgenes are
targeted to a fixed locus or fixed loci within the cellular DNA.
This means that promoter activity driving transcription of binder
genes in all clones will be the same and the functionality of each
binder will be a reflection of its inherent potency, translational
efficiency and stability rather than being due to variation related
to the integration site. Targeting to a single or limited number of
loci will also enable better control of expression if required
e.g., using inducible promoters.
[0062] Various features of the invention are further described
below. It is noted that headings used throughout this specification
are to assist navigation only and should not be interpreted as
definitive, and that embodiments described in different sections
may be combined as appropriate.
DETAILED DESCRIPTION
Eukaryotic Cells
[0063] The potential of populations of eukaryotic cells expressing
a diverse repertoire of binders is exemplified and discussed in the
Examples herein in relation to expression of antibody repertoires
on the surface of mammalian cells. The benefits of the invention
are not limited to mammalian cells and include all eukaryotes.
[0064] Yeast (e.g., Saccharomyces cerevisiae) has a smaller genome
than mammalian cells and homologous recombination directed by
homology arms (in the absence of nuclease-directed cleavage) is an
effective way of introducing foreign DNA compared to higher
eukaryotes. Thus, a particular benefit of nuclease-directed
integration of the present invention relates to integration of
binder genes into higher eukaryotic cells with larger genomes where
homologous recombination in the absence of nuclease cleavage is
less effective. Nuclease-directed integration has been used in
yeast cells to solve the problem of efficient integration of
multiple genes into individual yeast cells, e.g., for engineering
of metabolic pathways (US2012/0277120), but this work does not
incorporate introduction of libraries of binders nor does it
address the problems of library construction in higher
eukaryotes.
[0065] Libraries of eukaryotic cells according to the present
invention are preferably higher eukaryotic cells, defined here as
cells with a genome greater than that of Saccharomyces cerevisiae
which has a genome size of 12.times.10.sup.6 base pairs (bp). The
higher eukaryotic cells may for example have a genome size of
greater than 2.times.10.sup.7 base pairs. This includes, for
example, mammalian, avian, insect or plant cells. Preferably the
cells are mammalian cells, e.g., mouse or human. The cells may be
primary cells or may be cell lines. Chinese hamster ovary (CHO)
cells are commonly used for antibody and protein expression but any
alternative stable cell line may be used in the invention. HEK293
cells are used in Examples herein. Methods are available for
efficient introduction of foreign DNA into primary cells allowing
these to be used (e.g., by electroporation where efficiencies and
viabilities up to 95% have been achieved http://www.maxcyte.
com/technology/primary-cells-stem-cells.php).
[0066] T lymphocyte lineage cells (e.g., primary T cells or a T
cell line) or B lymphocyte lineage cells are among the preferred
cell types. Of particular interest are primary T-cells or T cell
derived cell lines for use in TCR libraries including cell lines
which lack TCR expression [23, 24, 25]. Examples of B lymphocyte
lineage cells include B cells, pre-B cells or pro-B cells and cell
lines derived from any of these.
[0067] Construction of libraries in primary B cells or B cell lines
would be of particular value for construction of antibody
libraries. Breous-Nystrom et al. [15] have generated libraries in a
murine pre-B cell line (1624-5). The chicken B cell derived cell
line DT40 (ATCC CRL-2111) has particular promise for construction
of libraries of binders. DT40 is a small cell line with a
relatively rapid rate of cell division. Repertoires of binders
could be targeted to specific loci using ZFNs, TALE nucleases or
CRISPR/Cas9 targeted to endogenous sequences or by targeting
pre-integrated heterologous sites which could include meganuclease
recognition sites. DT40 cells express antibodies and so it will be
advantageous to target antibody genes within the antibody locus
either with or without disruption of the endogenous chicken
antibody variable domains. DT40 cells have also been used as the
basis of an in vitro system for generation of chicken IgMs termed
the Autonomously Diversifying Library system (ADLib system) which
takes advantage of intrinsic diversification occurring at the
chicken antibody locus. As a result of this endogenous
diversification it is possible to generate novel specificities. The
nuclease-directed approach described here could be used in
combination with ADLib to combine diverse libraries of binders from
heterologous sources (e.g., human antibody variable region
repertoires or synthetically derived alternative scaffolds) with
the potential for further diversification with the chicken IgG
locus. Similar benefits could apply to human B cell lines such as
Nalm6 [26]. Other B lineage cell lines of interest include lines
such as the murine pre-B cell line 1624-5 and the pro-B cell line
Ba/F3. Ba/F3 is dependent on IL-3 [27] and its use is discussed
elsewhere herein. Finally a number of human cell lines could be
used including those listed in the "Cancer Cell Line Encyclopaedia"
[28] or "COSMIC catalogue of somatic mutations in cancer" [29].
[0068] Typically the library will be composed of a single type of
cells, produced by introduction of donor DNA into a population of
clonal eukaryotic cells, for example by introduction of donor DNA
into cells of a particular cell line. The main significant
difference between the different library clones will then be due to
integration of the donor DNA.
Eukaryotic Viral Systems
[0069] The advantages of the system in creation of libraries of
binders in eukaryotic cells could be applied to viral display
systems based around eukaryotic expression systems, e.g.,
baculoviral display or retroviral display [1, 2, 3, 4]. In this
approach each cell will encode a binder capable of being
incorporated into a viral particle. In the case of retroviral
systems the encoding mRNA would be packaged and the encoded binder
would be presented on the cell surface. In the case of baculoviral
systems, genes encoding the binder would need to be encapsulated
into the baculoviral particle to maintain an association between
the gene and the encoded protein. This could be achieved using host
cells carrying episomal copies of the baculoviral genome.
Alternatively integrated copies could be liberated following the
action of a specific nuclease (distinct from the one used to drive
site-specific integration). In the case of multimeric binder
molecules some partners could be encoded within the cellular DNA
with the genes for one or more partners being packaged within the
virus.
Site-Specific Nuclease
[0070] The invention involves use of a site-specific nuclease for
targeted cleavage of cellular DNA in the construction of a library
of eukaryotic cells containing DNA encoding a repertoire of
binders, wherein nuclease-mediated DNA cleavage enhances
site-specific integration of binder genes through endogenous
cellular DNA repair mechanisms. The site-specific nuclease cleaves
cellular DNA following specific binding to a recognition sequence,
thereby creating an integration site for donor DNA. The nuclease
may create a double strand break or a single strand break (a nick).
Cells used for creation of the library may contain endogenous
sequences recognised by the site-specific nuclease or the
recognition sequence may be engineered into the cellular DNA.
[0071] The site-specific nuclease may be exogenous to the cells,
i.e., not occurring naturally in cells of the chosen type.
[0072] The site-specific nuclease can be introduced before, after
or simultaneously with introduction of the donor DNA encoding the
binder. It may be convenient for the donor DNA to encode the
nuclease in addition to the binder, or on separate nucleic acid
which is co-transfected or otherwise introduced at the same time as
the donor DNA. Clones of a library may optionally retain nucleic
acid encoding the site-specific nuclease, or such nucleic acid may
be only transiently transfected into the cells.
[0073] Any suitable site-specific nuclease may be used with the
invention. It may be a naturally occurring enzyme or an engineered
variant. There are a number of known nucleases that are especially
suitable, such as those which recognise, or can be engineered to
recognise, sequences that occur only rarely in cellular DNA.
Nuclease cleavage at only one or two sites is advantageous since
this should ensure that only one or two molecules of donor DNA are
integrated per cell. Rarity of the sequence recognised by the
site-specific nuclease is more likely if the recognition sequence
is relatively long. The sequence specifically recognised by the
nuclease may for example be a sequence of at least 10, 15, 20, 25
or 30 nucleotides.
[0074] Examples of suitable nucleases include meganucleases, zinc
finger nucleases (ZFNs), TALE nucleases, and nucleic acid-guided
(e.g., RNA-guided) nucleases such as the CRISPR/Cas system. Each of
these produces double strand breaks although engineered forms are
known which generate single strand breaks.
[0075] Meganucleases (also known as homing endonucleases) are
nucleases which occur across all the kingdoms of life and recognise
relatively long sequences (12-40 bp). Given the long recognition
sequence they are either absent or occur relatively infrequently in
eukaryotic genomes. Meganucleases are grouped into 5 families based
on sequence/structure. (LAGLIDADG, GIY-YIG, HNH, His-Cys box and
PD-(D/E)XK). The best studied family is the LAGLIDADG family which
includes the well characterised I-SceI meganuclease from
Saccharomyces cerevisiae. I-SceI recognises and cleaves an 18 bp
recognition sequence (5' TAGGGATAACAGGGTAAT) leaving a 4 bp 3'
overhang. Another commonly used example is I-Cre1 which originates
from the chloroplast of the unicellular green algae of
Chlamydomonas reinhardtii, and recognizes a 22 bp sequence [30]. A
number of engineered variants have been created with altered
recognition sequences [31]. Meganucleases represent the first
example of the use of site-specific nucleases in genome engineering
[49, 50]. As with recombinase-based approaches, use of I-SceI and
other meganucleases requires prior insertion of an appropriate
recognition site to be targeted within the genome or engineering of
meganucleases to recognize endogenous sites [30]. By this approach
targeting efficiency in HEK293 cells (as judged by
homology-directed "repair" of an integrated defective GFP gene) was
achieved in 10-20% of cells through the use of I-SceI [32].
[0076] A preferred class of meganucleases for use in the present
invention is the LAGLIDADG endonucleases. These include I-Sce I,
I-Chu I, I-Cre I, Csm I, Pl-Sce I, PI-Tli I, PI-Mtu I, I-Ceu I,
I-Sce II, I-Sce III, HO, Pi-Civ I, PI-Ctr I, PI-Aae I, PI-Bsu I,
PI-Dha I, PI-Dra I, PI-Mav I, PI-Mch I, PI-Mfu PI-Mfl I, PI-Mga I,
PI-Mgo I, PI-Min I, PI-Mka I, PI-Mle I, PI-Mma I, PI-Msh I, PI-Msm
I, PI-Mth I, PI-Mtu PI-Mxe I, PI-Npu I, PI-Pfu I, PI-Rma I, Pl-Spb
I, PI-Ssp I, PI-Fac I, PI-Mja I, PI-Pho I, Pi-Tag I, PI-Thy I,
PI-Tko I, I-Msol, and PI-Tsp I; preferably, I-Sce I, I-Cre I, I-Chu
I, I-Dmo I, I-Csm I, Pl-Sce I, PI-Pfu I, PI-Tli I, PI-Mtu I, and
I-Ceu I.
[0077] In recent years a number of methods have been developed
which allow the design of novel sequence-specific nucleases by
fusing sequence-specific DNA binding domains to non-specific
nucleases to create designed sequence-specific nucleases directed
through bespoke DNA binding domains. Binding specificity can be
directed by engineered binding domains such as zinc finger domains.
These are small modular domains, stabilized by Zinc ions, which are
involved in molecular recognition and are used in nature to
recognize DNA sequences. Arrays of zinc finger domains have been
engineered for sequence specific binding and have been linked to
the non-specific DNA cleavage domain of the type II restriction
enzyme Fok1 to create zinc finger nucleases (ZFNs). ZFNs can be
used to create double stranded break at specific sites within the
genome. Fok1 is an obligate dimer and requires two ZFNs to bind in
close proximity to effect cleavage. The specificity of engineered
nucleases has been enhanced and their toxicity reduced by creating
two different Fok1 variants which are engineering to only form
heterodimers with each other [33]. Such obligate heterodimer ZFNs
have been shown to achieve homology-directed integration in 5-18%
of target cells without the need for drug selection [21, 34, 35].
Incorporation of inserts up to 8 kb with frequencies of >5% have
been demonstrated in the absence of selection.
[0078] It has recently been shown that single-stranded 5' overhangs
created by nucleases such as ZFNs help drive efficient integration
of transgenes to the sites of cleavage [45]. This has been extended
to show that in vivo cleavage of donor DNA (through inclusion of a
specific nuclease recognition site within the donor plasmid)
enhances the efficiency on non-homologous integration. The
mechanism is not entirely clear but it is possible that reduced
exposure to cellular nucleases through in vivo linearisation may
have contributed to the enhancement [45]. It is also possible that
matches in the 5' overhangs of donor and acceptor DNA, generated by
the nucleases drive ligation. Examination of sequences at the
junctions however showed the occurrence of deletions. It is
possible that perfectly matched junctions continue to act as
substrate for the site-directed nucleases until deletion of the
recognition sequence occurs. To overcome this potential problem,
Maresca et al. [36] have inverted the recognition sites of left and
right ZFNs within the donor DNA such that ligation of donor DNA
into the genomic locus will lead to duplication of two left hand
ZFNs on one flank of the integration and duplication of two right
hand ZFNs at the other flank. The use of obligate heterodimer
nucleases (as described for Fok1) means that neither of these newly
created flanking sequences can be cleaved by the targeted
nuclease.
[0079] The ability to engineer DNA binding domains of defined
specificity has been further simplified by the discovery in
Xanathomonas bacteria of Transcription activator-like effectors
(TALE) molecules. These TALE molecules consist of arrays of
monomers of 33-35 amino acids with each monomer recognising a
single base within a target sequence [37]. This modular 1:1
relationship has made it relatively easy to design engineered TALE
molecules to bind any DNA target of interest. By coupling these
designed TALEs to Fok1 it has been possible to create novel
sequence-specific TALE-nucleases. TALE nucleases, also known as
TALENs, have now been designed to a large number of sites and
exhibit high success rate for efficient gene modification activity
[38]. In examples herein we demonstrate the enhanced integration of
donor DNA through the use of TALE nucleases. Other variations and
enhancements of TALE nuclease technology have been developed and
could be used for the generation of libraries of binders through
nuclease-directed integration. These included "mega-TALENs" where a
TALE nuclease binding domain is fused to a meganuclease [39] and
"compact TALENs" where a single TALE nuclease recognition domain is
used to effect cleavage [40].
[0080] In recent years another system for directing double- or
single-stranded breaks to specific sequences in the genome has been
described. This system called "Clustered Regularly Interspaced
Short Palindromic Repeats (CRISPR) and CRISPR Associated (Cas)"
system is based on a bacterial defence mechanism [41]. The
CRISPR/Cas system targets DNA for cleavage via a short,
complementary single-stranded RNA (CRISPR RNA or crRNA) adjoined to
a short palindromic repeat. In the commonly used "Type II" system,
the processing of the targeting RNA is dependent on the presence of
a trans-activating crRNA (tracrRNA) that has sequence complementary
to the palindromic repeat. Hybridization of the tracrRNA to the
palindromic repeat sequence triggers processing. The processed RNA
activates the Cas9 domain and directs its activity to the
complementary sequence within DNA. The system has been simplified
to direct Cas9 cleavage from a single RNA transcript and has been
directed to many different sequences within the genome [42, 43].
This approach to genome cleavage has the advantage of being
directed via a short RNA sequence making it relatively simple to
engineer cleavage specificity. Thus there are a number of different
ways to achieve site-specific cleavage of genomic DNA. As described
above this enhances the rate of integration of a donor plasmid
through endogenous cellular DNA repair mechanisms.
[0081] Use of meganucleases, ZFNs, TALE nuclease or nucleic acid
guided systems such as the CRISPR/Cas9 systems will enable
targeting of endogenous loci within the genome. In the Examples
herein we have demonstrated targeting to the AAVS locus but
alternative loci could be targeted. For example the Type I collagen
gene locus has been used for efficient transgene expression
[44].
[0082] Alternatively heterologous recognition sites for targeted
nucleases, including meganucleases, ZFNs and TALE nucleases could
be introduced in advance for subsequent library targeting. In
Examples herein, we describe the use of a TALE nuclease recognising
a sequence within the AAVS locus to introduce by homologous
recombination, an I-Scel meganuclease recognition sequence and
heterologous TALE nuclease recognition sites within the AAVS locus.
Nuclease-directed targeting could be used to drive insertion of
target sequences by homologous recombination or NHEJ using vector
DNA or even double stranded oligonucleotides [45]. As an
alternative, non-specific targeting methods could be used to
introduce targeting sites through the use of transposon-directed
integration [46] to introduce recognition sites for site-specific
nucleases. Viral-based systems, such as lentivirus, applied at low
titre could also be used to introduce targeting sites. Transfection
of DNA coupled with screening for single copy insertion has also
been used to identify unique integration sites [17]. Such
non-specific approaches would be particularly useful in the case of
cells which do not have an obvious site to target or for genomes
which have not been sequenced or for genomes for which no existing
TALE nucleases, ZFNs or Cas9/CRISPR systems are available. Once a
cell line has been established following random insertion of a
nuclease recognition site, the cell line can be used subsequently
to create libraries of binders where all clones of the library
contain the transgene at the fixed locus using nuclease-directed
integration.
[0083] In the Examples presented, three different plasmids are used
encompassing pairs of TALE nucleases or ZFNs on individual plasmids
with a separate plasmid for donor DNA. In the case of meganuclease
the site-specific nuclease is encoded by a single gene and this is
introduced on one plasmid with the donor DNA present on a second
plasmid. Of course, combinations could be used incorporating two or
more of these elements on the same plasmid and this could enhance
the efficiency of targeting by reducing the number of number of
plasmids to be introduced. In addition it may be possible to
pre-integrate the nuclease(s) which could also be inducible to
allow temporal control of nuclease activity as has been
demonstrated for transposases [46]. Finally the nuclease could be
introduced as recombinant protein or protein:RNA complex (for
example in the case of an RNA directed nuclease such as
CRISPR:Cas9).
Locus
[0084] A recognition sequence for the site-specific nuclease may be
present in genomic DNA, or episomal DNA which is stably inherited
in the cells. Donor DNA may therefore be integrated at a genomic or
episomal locus in the cellular DNA.
[0085] In its simplest form a single gene encoding a binder (binder
gene) is targeted to a single site within the eukaryotic genome.
Identification of a cell demonstrating a particular binding
activity or cellular phenotype will allow direct isolation of the
gene encoding the desired property (e.g., by PCR from mRNA or
genomic DNA). This is facilitated by using a unique recognition
sequence for the site-specific nuclease, occurring once in the
cellular DNA. Cells used for creation of the library may thus
contain a nuclease recognition sequence at a single fixed locus,
i.e., one identical locus in all cells. Libraries produced from
such cells will contain donor DNA integrated at the fixed locus,
i.e., occurring at the same locus in cellular DNA of all clones in
the library.
[0086] Optionally, recognition sequences may occur multiple times
in cellular DNA, so that the cells have more than one potential
integration site for donor DNA. This would be a typical situation
for diploid or polyploid cells where the recognition sequence is
present at corresponding positions in a pair of chromosomes, i.e.,
replicate loci. Libraries produced from such cells may contain
donor DNA integrated at replicate fixed loci. For example libraries
produced from diploid cells may have donor DNA integrated at
duplicate fixed loci and libraries produced from triploid cells may
have donor DNA integrated at triplicate fixed loci. Many suitable
mammalian cells are diploid, and clones of mammalian cell libraries
according to the invention may have donor DNA integrated at
duplicate fixed loci.
[0087] The sequence recognised by the site-specific nuclease may
occur at more than one independent locus in the cellular DNA. Donor
DNA may therefore integrate at multiple independent loci. Libraries
of diploid or polyploid cells may comprise donor DNA integrated at
multiple independent fixed loci and/or at replicate fixed loci.
[0088] In cells containing recognition sequences at multiple loci
(whether replicate or independent loci), each locus represents a
potential integration site for a molecule of donor DNA.
Introduction of donor DNA into the cells may result in integration
at the full number of nuclease recognition sequences present in the
cell, or the donor DNA may integrate at some but not all of these
potential sites. For example, when producing a library from diploid
cells containing recognition sequences at first and second fixed
loci (e.g., duplicate fixed loci), the resulting library may
comprise clones in which donor DNA is integrated at the first fixed
locus, clones in which donor DNA is integrated at the second fixed
locus, and clones in which donor DNA is integrated at both the
first and second fixed loci.
[0089] Methods of producing libraries may therefore involve
site-specific nuclease cleavage of multiple fixed loci in a cell,
and integration of donor DNA at the multiple fixed loci. As noted
above, in cases where there are multiple copies of the same
recognition sequence (e.g., as occurs when targeting endogenous
loci in diploid or polyploid cells) it is possible that two binder
genes will be integrated, particularly when an efficient targeting
mechanisms is used, with only one gene being specific to the
target. This can be resolved during subsequent screening once
binder genes have been isolated.
[0090] In some instances it may be desirable to introduce more than
one binder per cell. For example bi-specific binders could be
generated from two different antibodies coming together and these
may have properties absent in the individual binders [47]. This
could be achieved by introducing different antibody genes into both
alleles at duplicate fixed loci or by targeting different antibody
populations into independent fixed loci using the methods described
herein. Furthermore a binder may itself be composed of multiple
chains (e.g., antibody VH and VL domains presented within a Fab or
IgG format). In this case it may be desirable to integrate the
different sub-units into different loci. These could be integrated
within the same cycle of nuclease-directed integration, they could
be integrated sequentially using nuclease-directed integration for
one or both integration steps.
Introduction of Donor DNA
[0091] Numerous methods have been described for introducing donor
DNA into eukaryotic cells, including transfection, infection or
electroporation. Transfection of large numbers of cells is possible
by standard methods including polyethyleneimine-mediated
transfection as described herein. In addition methods are available
for highly efficient electroporation of 10.sup.10 cells in 5
minutes, e.g., http://www.maxcyte.com.
[0092] Combinatorial libraries could be created wherein members of
multimeric binding pairs (e.g., VH and VL genes of antibody genes)
or even different parts of the same binder molecule are introduced
on different plasmids. Introduction of separate donor DNA molecules
encoding separate binders or binder subunits may be done
simultaneously or sequentially. For example an antibody light chain
could be introduced by transfection or infection, the cells grown
up and selected if necessary. Other components could then be
introduced in a subsequent infection or transfection step. One or
both steps could involve nuclease-directed integration to specific
genomic loci.
Integration of Donor DNA
[0093] The donor DNA is integrated into the cellular DNA, forming
recombinant DNA having a contiguous DNA sequence in which the donor
DNA is inserted at the integration site. In the present invention,
integration is mediated by the natural DNA repair mechanisms that
are endogenous to the cell. Thus, integration can be allowed to
occur simply by introducing the donor DNA into a cell, allowing the
site-specific nuclease to create an integration site, and allowing
the donor DNA to be integrated. Cells may be kept in culture for
sufficient time for the DNA to be integrated. This will usually
result in a mixed population of cells, including (i) recombinant
cells into which the donor DNA has integrated at the integration
site created by the site-specific nuclease, and optionally (ii)
cells in which donor DNA has integrated at sites other than the
desired integration site and/or optionally (iii) cells that into
which donor DNA has not integrated. The desired recombinant cells
and the resulting clones of the library may thus be provided in a
mixed population of other eukaryotic cells. Selection methods
described elsewhere herein may be used to enrich for cells of the
library.
[0094] Endogenous DNA repair mechanisms in eukaryotic cells include
homologous recombination, non-homologous end joining (NHEJ) and
microhomology-directed end joining. The efficiency of DNA
modification by such processes can be increased by the introduction
of double stranded breaks (DSBs) in the DNA and efficiency gains of
40,000 fold have been reported using rare cutting endonucleases
(meganucleases) such as I-SceI [48, 49, 50].
[0095] Unlike the site-specific recombination involved in systems
such as the Flp-In system [16], the present invention does not
require exogenous recombinases or engineered recombinase
recognition sites. Therefore, optionally the present invention does
not include a step of recombinase-mediated DNA integration in
creating the library, and/or optionally the eukaryotic cells into
which the donor DNA is introduced lack a recombination site for a
site-specific recombinase. The mechanisms and practicalities of
directed insertion of donor DNA into cellular DNA by recombinases
and nucleases are very distinct. As discussed by Jasin 1996
[50]:
[0096] " . . . the reaction catalyzed by site-specific recombinases
is quite distinct from cellular repair of DSBs. Site-specific
recombinases, such as cre, synapse two recognition sites and create
single-strand breaks within the sites, thus forming Holliday
intermediates. The intermediates are resolved to produce deletions,
inversions and insertions (cointegrants), all of which restore the
two recognition sites. The reaction is absolutely precise and,
hence, reversible. The breaks are never exposed to the cellular
repair machinery."
[0097] In contrast site-specific nuclease act to create breaks or
nicks within the cellular DNA (e.g., genomic or episomal), which
are exposed to and repaired by endogenous cellular repair
mechanisms such as homologous recombination or NHEJ.
Recombinase-based approaches have an absolute requirement for
pre-integration of their recognition sites, so such methods require
engineering of the "hot spot" integration site into the cellular
DNA as a preliminary step. With nuclease-directed integration it is
possible to engineer nucleases or direct via guide RNA in the case
of CRISPR:Cas9 to recognise endogenous loci, i.e., nucleic acid
sequences occurring naturally in the cellular DNA. Finally, at a
practical level nuclease-directed approaches are more efficient for
direct integration of transgenes at the levels required to make
large libraries of binders.
[0098] The DNA repair mechanism by which the donor DNA is
integrated in methods of the invention can be pre-determined or
biased to some extent by design of the donor DNA and/or choice of
site-specific nuclease.
[0099] Homologous recombination is a natural mechanism used by
cells to repair double stranded breaks using homologous sequence
(e.g., from another allele) as a template for repair. Homologous
recombination has been utilised in cellular engineering to
introduce insertions (including transgenes), deletions and point
mutations into the genome. Homologous recombination is promoted by
providing homology arms on the donor DNA. The original approach to
engineering higher eukaryotic cells typically used homology arms of
5-10 kb within a donor plasmid to increase efficiency of targeted
integration into the site of interest. Despite this, homologous
recombination driven purely by long homology arms, is less
efficient than Flp and Cre directed recombination particularly in
higher eukaryotes with large genomes. Homologous recombination is
particularly suitable for eukaryotes such as yeast, which has a
genome size of only 12.5.times.10.sup.6 bp, where it is more
effective compared with higher eukaryotes with larger genomes e.g.,
mammalian cells with 3000.times.10.sup.6 bp.
[0100] Homologous recombination can also be directed through [52]
nicks in genomic DNA and this could also serve as a route for
nuclease-directed integration into genomic DNA. Two distinct
pathways have been shown to promote homologous recombination at
nicked DNA. One is essentially similar to repair at double strand
breaks, utilizing Rad51/Brca2, while the other is inhibited by
Rad51/Brca2 and preferentially uses single-stranded DNA or nicked
double stranded donor DNA [51].
[0101] Non homologous end-joining (NHEJ) is an alternative
mechanism to repair double stranded breaks in the genome where the
ends of DNA are directly re-ligated without the need for a
homologous template. Nuclease-directed cleavage of genomic DNA can
also enhance transgene integration via non-homology based
mechanisms. This approach to DNA repair is less accurate and can
lead to insertions or deletions. NHEJ nonetheless provides a simple
means of integrating in-frame exons into intron or allows
integration of promoter:gene cassettes into the genome. Use of
non-homologous methods allows the use of donor vectors which lack
homology arms thereby simplifying the construction of donor
DNA.
[0102] It has been pointed out that short regions of terminal
homology are used to re-join DNA ends and it was hypothesized that
4 bp of microhomology might be utilized for directing repairing at
double strand breaks, referred to as microhomology-directed end
joining [50].
Donor DNA
[0103] The donor DNA will usually be circularised DNA, and may be
provided as a plasmid or vector. Linear DNA is another possibility.
Donor DNA molecules may comprise regions that do not integrate into
the cellular DNA, in addition to one or more donor DNA sequences
that integrate into the cellular DNA. The DNA is typically
double-stranded, although single-stranded DNA may be used in some
cases. The donor DNA contains one or more transgenes encoding a
binder, for example it may comprise a promoter:gene cassette.
[0104] In the simplest format double-stranded, circular plasmid DNA
can be used to drive homologous recombination. This requires
regions of DNA flanking the transgenes which are homologous to DNA
sequence flanking the cleavage site in genomic DNA. Linearised
double-stranded plasmid DNA or PCR product or synthetic genes could
be used to drive both homologous recombination and NHEJ repair
pathways. As an alternative to double-stranded DNA it is possible
to use single-stranded DNA to drive homologous recombination [52].
A common approach to generating single-stranded DNA is to include a
single-stranded origin of replication from a filamentous
bacteriophage into the plasmid.
[0105] Single-stranded DNA viruses such as adeno-associated virus
(AAV) have been used to drive efficient homologous recombination
where the efficiency has been shown to be improved by several
orders of magnitude [53, 54]. Systems such as the AAV systems could
be used in conjunction with nuclease-directed cleavage for the
construction of large libraries of binders. The benefits of both
systems could be applied to targeting of libraries of binders. The
packaging limit of AAV vectors is 4.7 kb but the use of nuclease
digestion of target genomic DNA will reduce this allowing larger
transgene constructs to be incorporated.
[0106] A molecule of donor DNA may encode a single binder or
multiple binders. Optionally, multiple subunits of a binder may be
encoded per molecule of donor DNA. In some embodiments, donor DNA
encodes a subunit of a multimeric binder.
Promoters and Genetic Elements for Selection
[0107] Transcription of the binder from the encoding donor DNA will
usually be achieved by placing the sequence encoding the binder
under control of a promoter and optionally one or more enhancer
elements for transcription. A promoter (and optionally other
genetic control elements) may be included in the donor DNA molecule
itself. Alternatively, the sequence encoding the binder may lack a
promoter on the donor DNA, and instead may be placed in operable
linkage with a promoter on the cellular DNA, e.g., an endogenous
promoter or a pre-integrated exogenous promoter, as a result of its
insertion at the integration site created by the site-specific
nuclease.
[0108] Donor DNA may further comprise one or more further coding
sequences, such as genetic elements enabling selection of cells
containing or expressing the donor DNA. As with the sequence
encoding the binder, discussed above, such elements may be
associated with a promoter on the donor DNA or may be placed under
control of a promoter as a result of integration of the donor DNA
at a fixed locus. The latter arrangement provides a convenient
means of selecting specifically for those cells which have
integrated the donor DNA at the desired site, since these cells
should express the genetic element for selection. This may be, for
example, a gene conferring resistance to a negative selection agent
such as blasticidin or puromycin. One or more selection steps may
be applied to remove unwanted cells, such as cells that lack the
donor DNA or which have not integrated the donor DNA at the correct
position. Alternatively these cells may be permitted to remain
mixed with clones of the library.
[0109] The expression of a membrane anchored binder could itself be
used as a form of selectable marker. For example if a library of
antibody genes, formatted as IgG or scFv-Fc fusions are introduced,
then cells which express the antibody can be selected using
secondary reagents which recognise the surface expressed Fc using
methods described herein. Upon initial transfection with donor DNA
encoding the transgene under the control of an exogenous promoter,
transient expression (and cell surface expression) of the binder
will occur and it will be necessary to wait for transient
expression to abate (to achieve targeted integration of e.g., 1-2
antibody genes/cell).
[0110] As an alternative a construct encoding a membrane tethering
element (e.g., the Fc domain of the present example fused to the
PDGF receptor transmembrane domain) could be pre-integrated before
the library of binders is introduced. If this membrane-tethering
element lacks a promoter or is encoded within an exon which is out
of frame with the preceding exon then surface expression will be
compromised. Targeted integration of an incoming donor molecule can
then correct this defect (e.g., by targeting a promoter or an
"in-frame" exon into the intron which is upstream of the defective
tethering element). If the frame "correcting exon" also encodes a
binder then a fusion will be produced between the binder and the
membrane tethering element resulting in surface expression of both.
Thus correctly targeted integration will result in-frame expression
of the membrane tethering element alone or as part of a fusion with
the incoming binder. Furthermore if the incoming library of binders
lack a membrane tethering element and these are incorrectly
integrated they will not be selected. Thus expression of the binder
itself on the cell surface can be used to select the population of
cells with correctly targeted integration.
Number of Clones and Library Diversity
[0111] Yeast display libraries of 10.sup.7-1010 have previously
been constructed and demonstrated to yield binders in the absence
of immunisation or pre-selection of the population [9, 55, 56, 57].
Many of the previously published mammalian display libraries used
antibody genes derived from immunised donors or even enriched
antigen-specific B lymphocytes, given the limitations of library
size and variability when using cells from higher eukaryotes.
Thanks to the efficiency of gene targeting described in the present
invention large, naive libraries can be constructed in higher
eukaryotes such as mammalian cells, which match those described for
simpler eukaryotes such as yeast.
[0112] Following integration of donor DNA into the cellular DNA,
the resulting recombinant cells are cultured to allow their
replication, generating a clone of cells from each
initially-produced recombinant cell. Each clone is thus derived
from one original cell into which donor DNA was integrated at an
integration site created by the site-specific nuclease. Methods
according to the present invention are associated with a high
efficiency and high fidelity of donor DNA integration, and a
library according to the present invention may contain at least
100, 10.sup.3, 10.sup.4, 10.sup.5, 10.sup.6, 10.sup.7, 10.sup.8,
10.sup.9 or 10.sup.10 clones.
[0113] Using nuclease-directed integration it is possible to target
10% or more of transfected mammalian cells. It is also practical to
grow and transform >10.sup.10 cells (e.g. from 5 litres of cells
growing at 2.times.10.sup.6 cells/ml). Transfection of such large
numbers of cells could be done using standard methods including
polyethyleneimine-mediated transfection as described herein. In
addition methods are available for highly efficient electroporation
of 10.sup.10 cells in 5 minutes e.g. http://www.maxcyte.com. Thus
using the approach of the present invention it is possible to
create libraries in excess of 10.sup.9 clones.
[0114] When the population of donor DNA molecules that is used to
create the library contains multiple copies of the same sequence,
two or more clones may be obtained that contain DNA encoding the
same binder. It can also be the case that a clone may contain donor
DNA encoding more than one different binder, for example if there
is more than one recognition sequence for the site-specific
nuclease, as detailed elsewhere herein. Thus, the diversity of the
library, in terms of the number of different binders encoded or
expressed, may be different from the number of clones obtained.
[0115] Clones in the library preferably contain donor DNA encoding
one or two members of the repertoire of binders and/or preferably
express only one or two members of the repertoire of binders. A
limited number of different binders per cell is an advantage when
it comes to identifying the clone and/or DNA encoding a particular
binder identified when screening the library against a given
target. This is simplest when clones encode a single member of the
repertoire of binders. However it is also straightforward to
identify the relevant encoding DNA for a desired binder if a clone
selected from a library encodes a small number of different
binders, for example a clone may encode two members of the
repertoire of binders. As discussed elsewhere herein, clones
encoding one or two binders are particularly convenient to generate
by selecting a recognition sequence for the site-specific nuclease
that occurs once per chromosomal copy in a diploid genome, as
diploid cells contain duplicate fixed loci, one on each chromosomal
copy, and the donor DNA may integrate at one or both fixed loci.
Thus, clones of the library may each express only one or two
members of the repertoire of binders.
[0116] Binders displayed on the surface of cells of the library may
be identical to (having the same amino acid sequence as) other
binders displayed on the same cell. The library may consist of
clones of cells which each display a single member of the
repertoire of binders, or of clones displaying a plurality of
members of the repertoire of binders per cell. Alternatively a
library may comprise some clones that display a single member of
the repertoire of binders, and some clones that display a plurality
of members (e.g., two) of the repertoire of binders.
[0117] Accordingly, a library according to the present invention
may comprise clones encoding more than one member of the repertoire
of binders, wherein the donor DNA is integrated at duplicate fixed
loci or multiple independent fixed loci.
[0118] As noted above, it is easiest to identify the corresponding
encoding DNA for a binder if the corresponding clone expresses only
one binder. Typically, a molecule of donor DNA will encode a single
binder. The binder may be multimeric so that a molecule of donor
DNA includes multiple genes or open reading frames corresponding to
the various subunits of the multimeric binder.
[0119] A library according to the present invention may encode at
least 100, 10.sup.3, 10.sup.4, 10.sup.5 or 10.sup.6, 10.sup.7,
10.sup.8, 10.sup.9 or 10.sup.10 different binders. Where the
binders are multimeric, diversity may be provided by one or more
subunits of the binder. Multimeric binders may combine one or more
variable subunits with one or more constant subunits, where the
constant subunits are the same (or of more limited diversity)
across all clones of the library. In generating libraries of
multimeric binders, combinatorial diversity is possible where a
first repertoire of binder subunits may pair with any of a second
repertoire of binder subunits.
Binders
[0120] A "binder" in accordance with the present invention is a
binding molecule, representing a specific binding partner for
another molecule. Typical examples of specific binding partners are
antibody-antigen and receptor-ligand.
[0121] The repertoire of binders encoded by a library will usually
share a common structure and have one or more regions of diversity.
The library therefore enables selection of a member of a desired
structural class of molecules, such as a peptide or a scFv antibody
molecule. For example, the binders may be polypeptides sharing a
common structure and having one or more regions of amino acid
sequence diversity.
[0122] This can be illustrated by considering a repertoire of
antibody molecules. These may be antibody molecules of a common
structural class, e.g., IgG, Fab, scFv-Fc or scFv, differing in one
or more regions of their sequence. Antibody molecules typically
have sequence variability in their complementarity determining
regions (CDRs), which are the regions primarily involved in antigen
recognition. A repertoire of binders in the present invention may
be a repertoire of antibody molecules which differ in one or more
CDRs, for example there may be sequence diversity in all six CDRs,
or in one or more particular CDRs such as the heavy chain CDR3
and/or light chain CDR3.
[0123] Antibody molecules and other binders are described in more
detail elsewhere herein. The potential of the present invention
however extends beyond antibody display to include display of
libraries of peptides or engineered proteins, including receptors,
ligands, individual protein domains and alternative protein
scaffolds [58, 59]. Nuclease-directed site-specific integration can
be used to make libraries of other types of binders previously
engineered using other display systems. Many of these involve
monomeric binding domains such as DARPins and lipocalins,
affibodies and adhirons [58, 59, 152]. Display on eukaryotes,
particularly mammalian cells, also opens up the possibility of
isolating and engineering binders or targets involving more
complex, multimeric targets. For example T cell receptors (TCRs)
are expressed on T cells and have evolved to recognise peptide
presented in complex with MHC molecules on antigen presenting
cells. Libraries encoding and expressing a repertoire of TCRs may
be generated, and may be screened to identify binding to MHC
peptide complexes as further described elsewhere herein.
[0124] For multimeric binders, donor DNA encoding the binder may be
provided as one or more DNA molecules. For example, where
individual antibody VH and VL domains are to be separately
expressed, these may be encoded on separate molecules of donor DNA.
The donor DNA integrates into the cellular DNA at multiple
integration sites, e.g., the binder gene for the VH at one locus
and the binder gene for the VL at a second locus. Methods of
introducing donor DNA encoding separate binder subunits are
described in more detail elsewhere herein. Alternatively, both
subunits or parts of a multimeric binder may be encoded on the same
molecule of donor DNA which integrates at a fixed locus.
[0125] A binder may be an antibody molecule or a non-antibody
protein that comprises an antigen-binding site. An antigen binding
site may be provided by means of arrangement of peptide loops on
non-antibody protein scaffolds such as fibronectin or cytochrome B
etc., or by randomising or mutating amino acid residues of a loop
within a protein scaffold to confer binding to a desired target
[60, 61, 62]. Protein scaffolds for antibody mimics are disclosed
in WO/0034784 in which the inventors describe proteins (antibody
mimics) that include a fibronectin type III domain having at least
one randomised loop. A suitable scaffold into which to graft one or
more peptide loops, e.g., a set of antibody VH CDR loops, may be
provided by any domain member of the immunoglobulin gene
superfamily. The scaffold may be a human or non-human protein.
[0126] Use of antigen binding sites in non-antibody protein
scaffolds has been reviewed previously [63]. Typical are proteins
having a stable backbone and one or more variable loops, in which
the amino acid sequence of the loop or loops is specifically or
randomly mutated to create an antigen-binding site having for
binding the target antigen. Such proteins include the IgG-binding
domains of protein A from S. aureus, transferrin, tetranectin,
fibronectin (e.g. 10th fibronectin type III domain) and lipocalins.
Other approaches include small constrained peptide e.g., based
on"knottin" and cyclotides scaffolds [64]. Given their small size
and complexity particularly in relation to correct formation of
disulphide bond, there may be advantages to the use of eukaryotic
cells for the selection of novel binders based on these scaffolds.
Given the common functions of these peptides in nature, libraries
of binders based on these scaffolds may be advantageous in
generating small high affinity binders with particular application
in blocking ion channels and proteases.
[0127] In addition to antibody sequences and/or an antigen-binding
site, a binder may comprise other amino acids, e.g., forming a
peptide or polypeptide, such as a folded domain, or to impart to
the molecule another functional characteristic in addition to
ability to bind antigen. A binder may carry a detectable label, or
may be conjugated to a toxin or a targeting moiety or enzyme (e.g.,
via a peptidyl bond or linker). For example, a binder may comprise
a catalytic site (e.g., in an enzyme domain) as well as an antigen
binding site, wherein the antigen binding site binds to the antigen
and thus targets the catalytic site to the antigen. The catalytic
site may inhibit biological function of the antigen, e.g., by
cleavage.
Antibody Molecules
[0128] Antibody molecules are preferred binders. Antibody molecules
may be whole antibodies or immunoglobulins (Ig), which have four
polypeptide chains--two identical heavy chains and two identical
light chains. The heavy and light chains form pairs, each having a
VH-VL domain pair that contains an antigen binding site. The heavy
and light chains also comprise constant regions: light chain CL,
and heavy chain CH1, CH2, CH3 and sometimes CH4 (the fifth domain
CH4 is present in human IgM and IgE). The two heavy chains are
joined by disulphide bridges at a flexible hinge region. An
antibody molecule may comprise a VH and/or a VL domain.
[0129] The most common native format of an antibody molecule is an
IgG which is a heterotetramer consisting of two identical heavy
chains and two identical light chains. The heavy and light chains
are made up of modular domains with a conserved secondary structure
consisting of a four-stranded antiparallel beta-sheet and a
three-stranded anti-parallel beta-sheet, stabilised by a single
disulphide bond. Antibody heavy chains each have an N terminal
variable domain (VH) and 3 relatively conserved "constant"
immunoglobulin domains (CH1, CH2, CH3) while the light chains have
one N terminal variable domain (VL) and one constant domains (CL).
Disulphide bonds stabilise individual domains and form covalent
linkages to join the four chains in a stable complex. The VL and CL
of the light chain associates with VH and CH1 of the heavy chain
and these elements can be expressed alone to form a Fab fragment.
The CH2 and CH3 domains (also called the "Fc domain") associate
with another CH2:CH3 pair to give a tetrameric Y shaped molecule
with the variable domains from the heavy and light chains at the
tips of the "Y". The CH2 and CH3 domains are responsible for the
interactions with effector cells and complement components within
the immune system. Recombinant antibodies have previously been
expressed in IgG format or as Fabs (consisting of a dimer of VH:CH1
and a light chain). In addition the artificial construct called a
single chain Fv (scFv) could be used consisting of DNA encoding VH
and VL fragments fused genetically with DNA encoding a flexible
linker.
[0130] Binders may be human antibody molecules. Thus, where
constant domains are present these are preferably human constant
domains.
[0131] Binders may be antibody fragments or smaller antibody
molecule formats, such as single chain antibody molecules. For
example, the antibody molecules may be scFv molecules, consisting
of a VH domain and a VL domain joined by a linker peptide. In the
scFv molecule, the VH and VL domains form a VH-VL pair in which the
complementarity determining regions of the VH and VL come together
to form an antigen binding site.
[0132] Other antibody fragments that comprise an antibody
antigen-binding site include, but are not limited to, (i) the Fab
fragment consisting of VL, VH, CL and CH1 domains; (ii) the Fd
fragment consisting of the VH and CH1 domains; (iii) the Fv
fragment consisting of the VL and VH domains of a single antibody;
(iv) the dAb fragment [65, 66, 67], which consists of a VH or a VL
domain; (v) isolated CDR regions; (vi) F(ab')2 fragments, a
bivalent fragment comprising two linked Fab fragments (vii) scFv,
wherein a VH domain and a VL domain are linked by a peptide linker
which allows the two domains to associate to form an antigen
binding site [68, 69]; (viii) bispecific single chain Fv dimers
(PCT/US92/09965) and (ix) "diabodies", multivalent or multispecific
fragments constructed by gene fusion (WO94/13804; [70]). Fv, scFv
or diabody molecules may be stabilised by the incorporation of
disulphide bridges linking the VH and VL domains [71].
[0133] Various other antibody molecules including one or more
antibody antigen-binding sites have been engineered, including for
example Fab2, Fab3, diabodies, triabodies, tetrabodies and
minibodies (small immune proteins). Antibody molecules and methods
for their construction and use have been described [72].
[0134] Other examples of binding fragments are Fab', which differs
from Fab fragments by the addition of a few residues at the
carboxyl terminus of the heavy chain CH1 domain, including one or
more cysteines from the antibody hinge region, and Fab'-SH, which
is a Fab' fragment in which the cysteine residue(s) of the constant
domains bear a free thiol group.
[0135] A dAb (domain antibody) is a small monomeric antigen-binding
fragment of an antibody, namely the variable region of an antibody
heavy or light chain. VH dAbs occur naturally in camelids (e.g.,
camel, llama) and may be produced by immunizing a camelid with a
target antigen, isolating antigen-specific B cells and directly
cloning dAb genes from individual B cells. dAbs are also producible
in cell culture. Their small size, good solubility and temperature
stability makes them particularly physiologically useful and
suitable for selection and affinity maturation. Camelid VH dAbs are
being developed for therapeutic use under the name
"Nanobodies.TM.".
[0136] Synthetic antibody molecules may be created by expression
from genes generated by means of oligonucleotides synthesized and
assembled within suitable expression vectors, for example as
described by Knappik et al. [73] or Krebs et al. [74].
[0137] Bispecific or bifunctional antibodies form a second
generation of monoclonal antibodies in which two different variable
regions are combined in the same molecule [75]. Their use has been
demonstrated both in the diagnostic field and in the therapy field
from their capacity to recruit new effector functions or to target
several molecules on the surface of tumour cells. Where bispecific
antibodies are to be used, these may be conventional bispecific
antibodies, which can be manufactured in a variety of ways [76],
e.g., prepared chemically or from hybrid hybridomas, or may be any
of the bispecific antibody fragments mentioned above. These
antibodies can be obtained by chemical methods [77, 78] or somatic
methods [79, 80] but likewise and preferentially by genetic
engineering techniques which allow the heterodimerisation to be
forced and thus facilitate the process of purification of the
antibody sought [81]. Examples of bispecific antibodies include
those of the BiTE.TM. technology in which the binding domains of
two antibodies with different specificity can be used and directly
linked via short flexible peptides. This combines two antibodies on
a short single polypeptide chain. Diabodies and scFv can be
constructed without an Fc region, using only variable domains,
potentially reducing the effects of anti-idiotypic reaction.
[0138] Bispecific antibodies can be constructed as entire IgG, as
bispecific Fab'2, as Fab'PEG, as diabodies or else as bispecific
scFv. Further, two bispecific antibodies can be linked using
routine methods known in the art to form tetravalent
antibodies.
[0139] Bispecific diabodies, as opposed to bispecific whole
antibodies, may also be particularly useful. Diabodies (and many
other polypeptides, such as antibody fragments) of appropriate
binding specificities can be readily selected. If one arm of the
diabody is to be kept constant, for instance, with a specificity
directed against an antigen of interest, then a library can be made
where the other arm is varied and an antibody of appropriate
specificity selected. Bispecific whole antibodies may be made by
alternative engineering methods as described in Ridgeway et al.,
1996 [82].
[0140] A library according to the invention may be used to select
an antibody molecule that binds one or more antigens of interest.
Selection from libraries is described in detail below. Following
selection, the antibody molecule may then be engineered into a
different format and/or to contain additional features. For
example, the selected antibody molecule may be converted to a
different format, such as one of the antibody formats described
above. The selected antibody molecules, and antibody molecules
comprising the VH and/or VL CDRs of the selected antibody
molecules, are an aspect of the present invention. Antibody
molecules and their encoding nucleic acid may be provided in
isolated form.
[0141] Antibody fragments can be obtained starting from an antibody
molecule by methods such as digestion by enzymes e.g. pepsin or
papain and/or by cleavage of the disulphide bridges by chemical
reduction. In another manner, the antibody fragments can be
obtained by techniques of genetic recombination well known to the
person skilled in the art or else by peptide synthesis by means of,
for example, automatic peptide synthesisers, or by nucleic acid
synthesis and expression.
[0142] It is possible to take monoclonal and other antibodies and
use techniques of recombinant DNA technology to produce other
antibodies or chimaeric molecules that bind the target antigen.
Such techniques may involve introducing DNA encoding the
immunoglobulin variable region, or the CDRs, of an antibody to the
constant regions, or constant regions plus framework regions, of a
different immunoglobulin. See, for instance, EP-A-184187, GB
2188638A or EP-A-239400, and a large body of subsequent
literature.
[0143] Antibody molecules may be selected from a library and then
modified, for example the in vivo half-life of an antibody molecule
can be increased by chemical modification, for example PEGylation,
or by incorporation in a liposome.
Sources of Binder Genes
[0144] The traditional route for generation of monoclonal
antibodies utilises the immune system of laboratory animals like
mice and rabbits to generate a pool of high affinity antibodies
which are then isolated by the use of hybridoma technology. The
present invention provides an alternative route to identifying
antibodies arising from immunisation. VH and VL genes could be
amplified from the B cells of immunised animals and cloned into an
appropriate vector for introduction into eukaryotic libraries
followed by selection from these libraries. Phage display and
ribosome display allows very large libraries (>10.sup.9 clones)
to be constructed enabling isolation of human antibodies without
immunisation. The present invention could also be used in
conjunction with such methods. Following rounds of phage display
selection, the selected population of binders could be introduced
into eukaryotic cells by nuclease-directed integration as described
herein. This would allow the initial use of very large libraries
based in other systems (e.g., phage display) to enrich a population
of binders while allowing their efficient screening using
eukaryotic cells as described above. Thus the invention can combine
the best features of both phage display and eukaryotic display to
give a high throughput system with quantitative screening and
sorting.
[0145] Using phage display and yeast display it has previously been
demonstrated that it is also possible to generate binders without
resorting to immunisation, provided display libraries of sufficient
size are used. For example multiple binders were generated from a
non-immune antibody library of >10.sup.7 clones [83]. This in
turn allows generation of binders to targets which are difficult by
traditional immunisation routes e.g., generation of antibodies to
"self-antigens" or epitopes which are conserved between species.
For example, human/mouse cross-reactive binders can be enriched by
sequential selection on human and then mouse versions of the same
target. Since it is not possible to specifically immunise humans to
most targets of interest, this facility is particularly important
in allowing the generation of human antibodies which are preferred
for therapeutic approaches.
[0146] In examples of mammalian display to date, where library
sizes and quality were limited, binders have only been generated
using repertoires which were pre-enriched for binders, e.g., from
immunisation or from engineering of pre-existing binders. The
ability to make large libraries in eukaryotic cells and
particularly higher eukaryotes creates the possibility of isolating
binders direct from these libraries starting with non-immune
binders or binders which have not previously been selected within
another system. With the present invention it is possible to
generate binders from non-immune sources. This in turn opens up the
possibilities for using binder genes from multiple sources. Binder
genes could come from PCR of natural sources such as antibody
genes. Binder genes could also be re-cloned from existing
libraries, such as antibody phage display libraries, and cloned
into a suitable donor vector for nuclease-directed integration into
target cells. Binders may be completely or partially synthetic in
origin. Furthermore various types of binders are described
elsewhere herein, for example binder genes could encode antibodies
or could encode alternative scaffolds [58, 59], peptides or
engineered proteins or protein domains.
Binder Display
[0147] To provide a repertoire of binders for screening against a
target of interest, the library may be cultured to express the
binders in either soluble secreted form or in transmembrane form.
For cell surface display it is necessary to retain the expressed
binder on the surface of the cell which encodes it. Binders may
comprise or be linked to a membrane anchor, such as a transmembrane
domain, for extracellular display of the binder at the cell
surface. This may involve direct fusion of the binder to a membrane
localisation signal such as a GPI recognition sequence or to a
transmembrane domain such as the transmembrane domain of the PDGF
receptor [84]. Retention of binders at the cell surface can also be
done indirectly by association with another cell surface retained
molecule expressed within the same cell. This associated molecule
could itself be part of a heterodimeric binder, such as tethered
antibody heavy chain in association with a light chain partner that
is not directly tethered.
[0148] Although cell surface immobilisation facilitates selection
of the binder, in many applications it is necessary to prepare
cell-free, secreted binder. It will be possible to combine membrane
tethering and soluble secretion using a recapture method of
attaching the secreted binders to cell surface receptors. One
approach is to format the library of binders as secreted molecules
which can associate with a membrane anchored molecule expressed
within the same cell which can function to capture a secreted
binder. For example, in the case of antibodies or binder molecules
fused to antibody Fc domains, a membrane tethered Fc can "sample"
secreted binder molecules being expressed in the same cell
resulting in display of a monomeric fraction of the binder
molecules being expressed while the remainder is secreted in a
bivalent form (U.S. Pat. No. 8,551,715). An alternative is to use a
tethered IgG binding domain such as protein A.
[0149] Other methods for retaining secreted antibodies with the
cells producing them are reviewed in Kumar et al. (2012) [85] and
include encapsulation of cells within microdrops, matrix aided
capture, affinity capture surface display (ACSD), secretion and
capture technology (SECANT) and "cold capture" [85]. In examples
given for ACSD and SECANT [85], biotinylation is used to facilitate
immobilisation of streptavidin or a capture antibody on the cell
surface. The captured molecule in turn captures secreted
antibodies. In the example of SECANT in vivo biotinylated of the
secreted molecule occurs. Using the "cold capture" technique
secreted antibody can be detected on producer cells using
antibodies directed to the secreted molecule. It has been proposed
that this due to association of the secreted antibody with the
glycocalyx of the cell [86]. Alternatively it has been suggested
that the secreted product is trapped by staining antibodies on the
cell surface before being endocytosed [87]. The above methods have
been used to identify high expressing clones within a population
but could potentially be adapted for identification of binding
specificity, provided the association has sufficient longevity at
the cell surface.
[0150] Even when the binder is directly tethered to the cell
surface it is possible to generate a soluble product. For example
the gene encoding the selected binder can be recovered and cloned
into an expression vector lacking the membrane anchored sequence.
Alternatively, and as demonstrated in the Examples, an expression
construction can be used in which the transmembrane domain is
encoded within an exon flanked by recombination sites, e.g., ROX
recognition sites for Dre recombinase [88]. In this example the
exon encoding the transmembrane domain can be removed by
transfection with a gene encoding Dre recombinase to switch
expression to a secreted form. As demonstrated herein, secreted
antibody was produced by this method without the need for
recombinase action. This is presumably as a result of alternative
splicing [89].
[0151] Any of the above methods or other suitable approaches can be
used to ensure that binders expressed by clones of a library are
displayed on the surface of their expressing cells.
Screening to Identify Binders to a Target of Interest
[0152] As noted, the eukaryotic cell library may be used in a
method of screening for a binder that recognises a target. Such a
method may comprise:
[0153] providing a library as described herein,
[0154] culturing cells of the library to express the binders,
[0155] exposing the binders to the target, allowing recognition of
the target by one or more cognate binders, if present, and
[0156] detecting whether the target is recognised by a cognate
binder.
[0157] Selections could be carried using a range of target molecule
classes, e.g., protein, nucleic acid, carbohydrate, lipid, small
molecules. The target may be provided in soluble form. The target
may be labelled to facilitate detection, e.g., it may carry a
fluorescent label or it may be biotinylated. Cells expressing a
target-specific binder may be isolated using a directly or
indirectly labelled target molecule, where the binder captures the
labelled molecule. For example, cells that are bound, via the
binder:target interaction, to a fluorescently labelled target can
be detected and sorted by flow cytometry or FACS to isolate the
desired cells. Selections involving cytometry require target
molecules which are directly fluorescently labelled or are labelled
with molecules which can be detected with secondary reagents, e.g.,
biotinylated target can be added to cells and binding to the cell
surface can be detected with fluorescently labelled streptavidin
such as streptavidin-phycoerythrin. A further possibility is to
immobilise the target molecule or secondary reagents which bind to
the target on a solid surface, such as magnetic beads or agarose
beads, to allow enrichment of cells which bind the target. For
example cells that bind, via the binder: target interaction, to a
biotinylated target can be isolated on a substrate coated with
streptavidin, e.g., streptavidin-coated beads.
[0158] In screening libraries it is preferable to over-sample,
i.e., screen more clones than the number of independent clones
present within the library to ensure effective representation of
the library. Identifying binders from very large libraries provided
by the present invention could be done by flow sorting but this
would take several days, particularly if over-sampling the library.
As an alternative initial selections could be based on the use of
recoverable antigen, e.g., biotinylated antigen recovered on
streptavidin-coated magnetic beads. Thus streptavidin-coated
magnetic beads could be used to capture cells which have bound to
biotinylated antigen. Selection with magnetic beads could be used
as the only selection method or this could be done in conjunction
with flow cytometry where better resolution can be achieved, e.g.,
differentiating between a clone with higher expression levels and
one with a higher affinity [56, 57].
[0159] The in vitro nature of display technology approaches makes
it is possible to control selection in a way that is not possible
by immunisation, e.g., selecting on a particular conformational
state of a target [90, 91]. Targets could be tagged through
chemical modification (fluorescein, biotin) or by genetic fusion
(e.g. protein fused to an epitope tag such as a FLAG tag or another
protein domain or a whole protein). The tag could be nucleic acid
(e.g., DNA, RNA or non-biological nucleic acids) where the tag is
part fused to target nucleic acid or could be chemically attached
to another type of molecule such as a protein. This could be
through chemical conjugation or through enzymatic attachment [92].
Nucleic acid could be also fused to a target through a
translational process such as ribosome display. The "tag" may be
another modification occurring within the cell (e.g.,
glycosylation, phosphorylation, ubiqitinylation, alkylation,
PASylation, SUMO-lation and others described at the
Post-translational Database (db-PTM) at
http://dbptm.mbc.nctu.edu.tw/statistics.php) which can be detected
via secondary reagents. This would yield binders which bind an
unknown target protein on the basis of a particular
modification.
[0160] Targets could be detected using existing binders which bind
to that target molecule, e.g., target specific antibodies. Use of
existing binders for detection will have the added advantage of
identifying binders within the library of binders which recognise
an epitope distinct from the binder used for detection. In this way
pairs of binders could be identified for use in applications such
as sandwich ELISA. Where possible a purified target molecule would
be preferred. Alternatively the target may be displayed on the
surface of a population of target cells and the binders are
displayed on the surface of the library cells, the method
comprising exposing the binders to the target by bringing the
library cells into contact with the target cells. Recovery of the
cells expressing the target (e.g., using biotinylated cells
expressing target) will allow enrichment of cells which express
binders to them. This approach would be useful where low affinity
interactions are involved since there is the potential for a strong
avidity effect.
[0161] The target molecule could also be unpurified recombinant or
unpurified native targets provided a detection molecule is
available to identify cell binding (as described above). In
addition binding of target molecules to the cell expressing the
binder could be detected indirectly through the association of
target molecule to another molecule which is being detected, e.g.,
a cell lysate containing a tagged molecule could be incubated with
a library of binders to identify binders not only to the tagged
molecule but also binders to its associated partner proteins. This
would result in a panel of antibodies to these partners which could
be used to detect or identify the partner (e.g., using mass
spectrometry). Cellular fractionation could be used to enrich
targets from particular sub-cellular locations. Alternatively
differential biotinylation of surface or cytoplasmic fractions
could be used in conjunction with streptavidin detection reagents
for eukaryotic display [93,94]. The use of detergent solubilised
target preparations is a particularly useful approach for intact
membrane proteins such as GPCRs and ion channels which are
otherwise difficult to prepare. The presence of detergents may have
a detrimental effect on the eukaryotic cells displaying the binders
requiring recovery of binder genes without additional growth of the
selected cells.
[0162] Following detection of target recognition by a cognate
binder, cells of a clone containing DNA encoding the cognate binder
may be recovered. DNA encoding the binder may then be isolated
(e.g., identified or amplified) from the recovered clone, thereby
obtaining DNA encoding a binder that recognises the target.
[0163] Exemplary binders and targets are detailed elsewhere herein.
A classic example is a library of antibody molecules, which may be
screened for binding to a target antigen of interest. Other
examples include screening a library of TCRs against a target
MHC:peptide complex or screening a library of MHC:peptide complexes
against a target TCR.
TCR:MHC and Other Receptor Interactions
[0164] T cell receptors (TCRs) are expressed on T cells and have
evolved to recognise peptide presented in complex with MHC
molecules on antigen presenting cells. TCRs are heterodimers
consisting in 95% of cases of alpha and beta heterodimers and in 5%
of cases of gamma and delta heterodimers. Both monomer units have
an N terminal immunoglobulin domain which has 3 variable
complementarity determining regions (CDRs) involved in driving
interaction with target. The functional TCR is present within a
complex of other sub-units and signalling is enhanced by
co-stimulation with CD4 and CD8 molecules (specific for class I and
class II MHC molecules respectively). On antigen presenting cells,
proteins are processed, and presented on the cell surface in
complex with MHC molecules which are themselves part of a
multimeric protein complex. TCRs recognizing peptides originating
from "self" are removed during development and the system is poised
for recognition of foreign peptides presented on antigen presenting
cells to effect an immune response. The outcome of recognition of a
peptide:MHC complex depends on the identity of the T cell and the
affinity of that interaction.
[0165] It would be valuable to identify the genes encoding TCRs or
MHC:peptide complexes which drive interactions involved in
pathological conditions, e.g., as occurs in autoimmune disease. In
the case of autoimmune disease, identification of interacting
partners, e.g., a TCR driving a pathogenic condition could pave the
way to either specifically blocking such interactions or removing
offending cytotoxic cells. It would be desirable to engineer TCRs
for altered binding e.g. higher affinity to targets of interest,
e.g., in re-targeting T cells in cancer or enhancing the effect of
existing T cells [95]. Alternatively the behaviour of regulatory or
suppressive T cells might be altered as a therapeutic modality,
e.g., for directing or enhancing immunotherapy of cancer by
introducing specific TCRs into T cells or by using expressed TCR
protein as therapeutic entities [96].
[0166] Display of libraries of TCRs on surface of yeast cells and
mammalian cells has previously been demonstrated. In the case of
yeast cells it was necessary to engineer the TCR and present it in
a single chain format. Since the affinity of interaction between
TCR and peptide:MHC complex is low, the soluble component (e.g.,
peptide:MHC in this case) is usually presented in a multimeric
format. TCR specificity has been engineered for peptides in complex
with MHC class I [97] and MHC class II [98]. TCRs have also been
expressed on the surface of a mutant mouse T cells (lacking TCR
alpha and beta chains) and variant TCRs with improved binding
properties have been isolated [99]. For example Chervin et al.
introduced TCRs by retroviral infection and an effective library
size of 10.sup.4 clones was generated [100]. Using
nuclease-directed integration of binders as proposed here, a
similar approach could be taken to engineering T cells. As well as
selecting TCRs with altered recognition properties, display
libraries could be used to screen libraries of peptide or of MHC
variants for recognition by TCRs. For example peptide:MHC complexes
have been displayed on insect cells and used to epitope map TCRs
presented in a multimeric format [101].
[0167] As noted, screening methods may involve displaying the
repertoire of binders on the cell surface and probing with a target
presented as a soluble molecule, which may be a multimeric target.
An alternative, which can be especially useful with multimeric
targets, is to screen directly for cell:cell interactions, where
binder and target are presented on the surface of different cells.
For example if activation of a TCR of interest led to expression of
a reporter gene this could be used to identify activating peptides
or activating MHC molecules presented within a peptide:MHC library.
In this particular example the reporter cell does not encode the
library member but could be used to identify the cell which does
encode it. The approach could potentially extend to a "library
versus library" approach. For example extending the example
described above, a TCR library could be screened against a
peptide:MHC library. More broadly the example of screening a
library of binders presented on one cell surface using a binding
partner on another cell could be extended to other types of
cell:cell interactions e.g., identification of binders which
inhibit or activate signalling within the Notch or Wnt pathways.
Thus the present invention could be used in alternative cell based
screening system including recognition systems based on cell:cell
interactions.
[0168] As an example, chimeric antigen receptors ("CARS") represent
a fusion between an antibody binding domain (usually formatted as a
scFv) and a signalling domain. These have been introduced into T
cells with the aim of re-directing the T cell in vivo to attack
tumour cells through antibody recognition and binding to
tumour-specific antigens. A number of different factors could
affect the success of this strategy including the combination of
antibody specificity, format, antibody affinity, linker length,
fused signalling module, expression level in T cells, T cell
sub-type and interaction of the CAR with other signalling molecules
[102, 103]. The ability to create large libraries of CARs in
primary T cells incorporating individual or combinations of the
above variables would allow a functional search for effective and
optimal CAR construction. This functional "search" could be carried
out in vitro or in vivo. For example Alonso-Camino (2009) have
fused a scFv recognizing CEA to the .xi. chain of the TCR:CD3
complex and introduced this genetic construct into a human Jurkat
cell line [104]. Upon interaction with CEA present on either HeLa
cells or tumour cells they showed upregulation of the early T cell
activation marker CD69. This approach could be used to identify CAR
fusion constructs with appropriate activation or inhibitory
properties using cultured or primary cells.
[0169] Going one step further functionality of CAR constructs could
be assessed in vivo. For example a library of CARs constructed in
primary mouse T cells could be introduced into tumour bearing mice
to identify T cell clones stimulated to proliferate through
encounter with tumour. If necessary this T cell library could be
pre-selected based on antigen binding specificity using the methods
described above. In either case the incoming library of binders
could be used to replace an existing binder molecule (e.g., MHC or
TCR or antibody variable domain).
Phenotype Screens
[0170] Described here are various methods for selection of binders
which modify cell signalling and cellular behaviour.
[0171] A library may be screened for altered cellular phenotype as
a result of the action of the binder on its target.
[0172] Antibodies which modify cell signalling by binding to
ligands or receptors have a proven track record in drug development
and the demand for such therapeutic antibodies continues to grow.
Such antibodies and other classes of functional binders also have
potential in controlling cell behaviour in vivo and in vitro. The
ability to control and direct cellular behaviour however relies on
the availability of natural ligands which control specific
signalling pathways. Unfortunately many natural ligands such as
those controlling stem cell differentiation (e.g., members of FGF,
TGF-beta, Wnt and Notch super-families) often exhibit promiscuous
interactions and have limited availability due to their poor
expression/stability profiles. With their exquisite specificity,
antibodies have great potential in controlling cellular
behaviour.
[0173] The identification of functional antibodies that modify cell
signalling has historically been relatively laborious involving
picking clones, expressing antibody, characterising according to
sequence and binding properties, conversion to mammalian expression
systems and addition to functional cell based assays. The
eukaryotic display approach described herein will reduce this
effort but there is still a requirement for production of antibody
and addition to a separate reporter cell culture. Therefore, a
preferred alternative may be to directly screen libraries of
binders expressed in eukaryotic cells for the effect of binding on
cell signalling or cell behaviour by using the production cell
itself as a reporter cell. Following introduction of antibody
genes, clones within the resulting population of cells showing
alteration in reporter gene expression or altered phenotypes can be
identified.
[0174] A number of recent publications have described the
construction of antibody libraries by cloning repertoires of
antibody genes into reporter cells [47, 105, 106]. These systems
combine expression and reporting within one cell, and typically
introduce a population of antibodies selected against a pre-defined
target (e.g., using phage display).
[0175] A population of antibody genes may be introduced into
reporter cells to produce a library by methods described herein,
and clones within the population with an antibody-directed
alteration in phenotype (e.g., altered gene expression or survival)
can be identified. For this phenotypic-directed selection to work
there is a requirement to retain a linkage between the antibody
gene present within the expressing cell (genotype) and the
consequence of antibody expression (phenotype). This has been
achieved previously either through tethering the antibody to the
cell surface [47] as described for antibody display or through the
use of semi-solid medium to retain secreted antibodies in the
vicinity of producing cells [105]. Alternatively antibodies and
other binders can be retained inside the cell [107]. Binders
retained on the cell surface or in the surrounding medium can
interact with an endogenous or exogenous receptor on the cell
surface causing activation of the receptor. This in turn can cause
a change in expression of a reporter gene or a change in the
phenotype of the cell. As an alternative the antibody can block the
receptor or ligand to reduce receptor activation. The gene encoding
the binder which causes the modified cellular behaviour can then be
recovered for production or further engineering.
[0176] An alternative to this "target-directed" approach, it is
possible to introduce a "naive" antibody population which has not
been pre-selected to a particular target [108]. The cellular
reporting system is used to identify members of the population with
altered behaviour. Since there is no prior knowledge of the target,
this non-targeted approach has a particular requirement for a large
antibody repertoire, since pre-enrichment of the antibody
population to the target is not possible. This approach will
benefit from using nuclease-directed transgene integration as
described in the present invention.
[0177] The "functional selection" approach could be used on other
applications involving libraries in eukaryotic cells, particularly
higher eukaryotes such as mammalian cells. The antibody could be
fused to a signalling domain such that binding to target causes
activation of the receptor. Kawahara et al. have constructed
chimeric receptors where an extra-cellular scFv targeting
fluorescein was fused to a spacer domain (the D2 domain of the Epo
receptor) and various intracellular cytokine receptor domain
including the thrombopoeitin (Tpo) receptor, erythropoietin (Epo)
receptor, gp130, IL-2 receptor and the EGF receptor [109, 110,
111]. These were introduced into an IL-3 dependent proB cell line
(BaF3) [27], where chimaeric receptors were shown to exhibit
antigen-dependent activation of the chimaeric receptor leading to
IL-3 independent growth. This same approach was used in model
experiments to demonstrate antigen mediated chemoattraction of BaF3
cells [110]. The approach was extended beyond stable culture cells
to primary cells exemplified by the survival and growth of
Tpo-responsive haematopoeitic stem cells [112] or IL2 dependant
primary T cells where normal stimulation by Tpo and IL-2
respectively was replaced by fluorescein directed stimulation of
scFv chimaeric receptors. Thus a system based on chimaeric
antibody-receptor chimaeras can be used to drive target dependent
gene expression or phenotypic changes in primary or stable reporter
cells. This capacity could be used to identify fused binders which
drive a signalling response or binders which inhibit the
response.
[0178] In a modification of the above approach separate VH and VL
domains from an anti-lysozyme antibody were fused to the Epo
intracellular domain [113]. Cells grew in response to addition of
lysozyme indicating an antigen induced dimerisation or
stabilisation of the separate VH and VL fusion partners. Thus three
interacting components come together for an optimal response in
this system.
[0179] Although described here with reference to antibody
molecules, the above methods may also be adapted and performed with
libraries of other binders.
[0180] Protein fragment complementation represents an alternative
system for studying and for selecting protein:protein interactions
in mammalian cells [114, 115]. This involves restoring function of
split reporter proteins through protein:protein interactions.
Reporter proteins which have been used include ubiquitin, DNAE
intein, beta-galactosidase, dihydrofolate reductase, GFP, firefly
luciferase, beta-lactamase, TEV protease. For example a recent
example of this approach is the mammalian membrane 2 hybrid (MaMTH)
approach where association of a bait protein:split
ubiquitin:transcription factor fusion with a partner protein:split
ubiquitin restores ubiquitin recognition and liberates the
transcription factor to effect reporter gene expression [116].
Again binders which interfere with or enhance this interaction
could be identified through perturbed signalling.
Recovery and Reformatting of Binders and Encoding DNA
[0181] Following selection of a binder or clone of interest from
the library, a common next step will be to isolate (e.g., identify
or amplify) the DNA encoding the binder. Optionally, it may be
desired to modify the nucleic acid encoding the binder, for example
to restructure the binder and/or to insert the encoding sequence
into a different vector.
[0182] Where the binder is an antibody molecule, a method may
comprise isolating DNA encoding the antibody molecule from cells of
a clone, amplifying DNA encoding at least one antibody variable
region, preferably both the VH and VL domain, and inserting DNA
into a vector to provide a vector encoding the antibody molecule. A
multimeric antibody molecule bearing a constant domain may be
converted to a single chain antibody molecule for expression in a
soluble secreted form.
[0183] Antibodies may be presented in different formats but
whatever format an antibody is selected in, once the antibody gene
is isolated it is possible to reconfigure it in a number of
different formats. Once VH or VL domains are isolated, they can be
re-cloned into expression vectors encompassing the required partner
domains (see Example 1 showing a dual promoter IgG expression
cassette).
[0184] A reformatting step may comprise reformatting of binders
composed of a pair of subunits (e.g., scFv molecules), to a
different molecular binder format (e.g., Ig or Fab) in which the
original pairing of the subunits is maintained. Such methods are
described in more detail elsewhere herein and can be used for
monoclonal, oligoclonal or polyclonal clone reformatting. The
method can be used to convert "en masse" an entire output
population from any of the commonly used display technologies
including phage, yeast or ribosome display.
Display of scFvs on the Surface of Mammalian Cells Fused to Fc
Domains.
[0185] Although many antibody phage display libraries are formatted
to display scFvs, eukaryotic display systems will allow
presentation in Fab or IgG format. To take full advantage of the
potential for IgG/Fab expression, particularly when using scFvs
from other display systems will be necessary to take selected
linked VH and VL domains within a bacterial expression system and
express them within a eukaryotic system fused to appropriate
constant domains. Described here is a method to convert scFv
populations to immunoglobulin (Ig) or fragment, antigen binding
(Fab) format in such a way that original VH and VL chain pairings
are maintained. In the present invention, conversion is possible
using individual clones, oligoclonal mixes or whole populations
formatted as scFv while retaining the original pairing of VH and
VLs chains. The method proceeds via the generation of an
intermediate non-replicative "mini-circle" DNA which brings in a
new "stuffer" DNA fragment. The circular DNA is linearised (e.g.,
by restriction digestion or PCR) which alters the relative position
of the original VH and VL fragments and places the "stuffer" DNA
between them. Following linearization the product can be cloned
into a vector of choice, e.g., a mammalian expression vector. In
this way all of the elements apart from the VH and VL can be
replaced. Elements for bacterial expression can be replaced with
elements for mammalian expression and fusion to alternative
partners. The complete conversion process only requires a single
transformation step of E. coli bacteria to generate a population of
bacterial colonies each harbouring a plasmid encoding a unique Ig
or Fab formatted recombinant antibody. Extending beyond conversion
of scFv to IgG/Fab, the method can be employed to reformat any two
joined DNA elements to clone into a vector such that after
re-formatting each DNA element is surrounded by different DNA
control features whilst maintaining the original pairing. A
previous method has been described wherein 2 sequential cloning
steps are used [117] to replace these elements in contrast to the
present method which proceeds via an intermediate non-replicative
circular intermediate.
[0186] A method of restructuring a binder, or population of
binders, may comprise converting scFv to Ig or a fragment thereof,
e.g., Fab. The method may comprise converting nucleic acid encoding
scFv to DNA encoding an immunoglobulin (Ig) or fragment thereof
such as Fab format, in such a way that the original variable VH and
VL chain pairings are maintained. Preferably the conversion
proceeds via circular DNA intermediate which may be a
non-replicative "mini-circle" DNA. The method requires a single
transformation of E. coli for the direct generation of bacterial
transformants harbouring plasmids encoding Ig or Fab DNA.
[0187] The method may be used for monoclonal, oligoclonal or
polyclonal clone reformatting. The method may be used to convert
"en masse" an entire output population from any of the commonly
used display technologies including phage, yeast or ribosome
display.
[0188] More generally, this aspect of the invention relates to a
method that allows the reformatting of any two joined DNA elements
into a vector where the DNA elements are cloned under the control
of separate promoters, or separated by alternative control
elements, but maintaining the original DNA pairing.
[0189] Examples 7 and 14 describe such methods in further
detail.
[0190] Isolation, and optional restructuring, of DNA encoding
binders may be followed by introduction of that DNA into further
cells to create a derivative library as described elsewhere herein,
or DNA encoding one or more particular binders of interest may be
introduced into a host cell for expression. The host cell may be of
a different type compared with the cells of the library from which
it was obtained. Generally the DNA will be provided in a vector.
DNA introduced into the host cell may integrate into cellular DNA
of the host cell. Host cells expressing the secreted soluble
antibody molecule can then be selected.
[0191] Host cells encoding one or more binders may be provided in
culture medium and cultured to express the one or more binders.
Derivative Libraries
[0192] Following production of a library by the method of the
invention, one or more library clones may be selected and used to
produce a further, second generation library. When a library has
been generated by introducing DNA into eukaryotic cells as
described herein, the library may be cultured to express the
binders, and one or more clones expressing binders of interest may
be recovered, for example by selecting binders against a target as
described elsewhere herein. These clones may subsequently be used
to generate a derivative library containing DNA encoding a second
repertoire of binders.
[0193] To generate the derivative library, donor DNA of the one or
more recovered clones is mutated to provide the second repertoire
of binders. Mutations may be addition, substitution or deletion of
one or more nucleotides. Where the binder is a polypeptide,
mutation will be to change the sequence of the encoded binder by
addition, substitution or deletion of one or more amino acids.
Mutation may be focussed on one or more regions, such as one or
more CDRs of an antibody molecule, providing a repertoire of
binders of a common structural class which differ in one or more
regions of diversity, as described elsewhere herein.
[0194] Generating the derivative library may comprise isolating
donor DNA from the one or more recovered clones, introducing
mutation into the DNA to provide a derivative population of donor
DNA molecules encoding a second repertoire of binders, and
introducing the derivative population of donor DNA molecules into
cells to create a derivative library of cells containing DNA
encoding the second repertoire of binders.
[0195] Isolation of the donor DNA may involve obtaining and/or
identifying the DNA from the clone. Such methods may encompass
amplifying the DNA encoding a binder from a recovered clone, e.g.,
by PCR and introducing mutations. DNA may be sequenced and mutated
DNA synthesised.
[0196] Mutation may alternatively be introduced into the donor DNA
in the one or more recovered clones by inducing mutation of the DNA
within the clones. The derivative library may thus be created from
one or more clones without requiring isolation of the DNA, e.g.,
through endogenous mutation in avian DT40 cells.
[0197] Antibody display lends itself especially well to the
creation of derivative libraries. Once antibody genes are isolated,
it is possible to use a variety of mutagenesis approaches (e.g.,
error prone PCR, oligonucleotide-directed mutagenesis, chain
shuffling) to create display libraries of related clones from which
improved variants can be selected. For example, with
chain-shuffling the DNA encoding the population of selected VH
clone, oligoclonal mix or population can be sub-cloned into a
vector encoding a suitable antibody format and encoding a suitably
formatted repertoire of VL chains [118]. Alternatively and again
using the example of VHs, the VH clone, oligomix or population
could be introduced into a population of eukaryotic cells which
encode and express a population of appropriately formatted light
chain partners (e.g., a VL-CL chain for association with an IgG or
Fab formatted heavy chain). The VH population could arise from any
of the sources discussed above including B cells of immunised
animals or scFv genes from selected phage populations. In the
latter example cloning of selected VHs into a repertoire of light
chains could combine chain shuffling and re-formatting (e.g., into
IgG format) in one step.
[0198] A particular advantage of display on eukaryotic cells is the
ability to control the stringency of the selection/screening step.
By reducing antigen concentration, cells expressing the highest
affinity binders can be distinguished from lower affinity clones
within the population. The visualisation and quantification of the
affinity maturation process using flow cytometry is a maj or
benefit of eukaryotic display as it gives an early indication of
percentage positives in naive library and allows a direct
comparison between the affinity of the selected clones and the
parental population during sorting. Following sorting, the affinity
of individual clones can be determined by pre-incubating with a
range of antigen concentrations and analysis in flow cytometry or
with a homogenous Time Resolved Fluorescence (TRF) assay or using
surface plasmon resonance (SPR) (Biacore).
Characteristics and Form of the Library
[0199] The present invention enables construction of eukaryotic
cell libraries having many advantageous characteristics. The
invention provides libraries having any one or more of the
following features:
[0200] Diversity. A library may encode and/or express at least 100,
10.sup.3, 10.sup.4, 10.sup.5 10.sup.6, 10.sup.7, 10.sup.8 or
10.sup.9 different binders.
[0201] Uniform integration. A library may consist of clones
containing donor DNA integrated at a fixed locus, or at a limited
number of fixed loci in the cellular DNA. Each clone in the library
therefore contains donor DNA at the fixed locus or at least one of
the fixed loci. Preferably clones contain donor DNA integrated at
one or two fixed loci in the cellular DNA. As explained elsewhere
herein, the integration site is at a recognition sequence for a
site-specific nuclease. Integration of donor DNA to produce
recombinant DNA is described in detail elsewhere herein and can
generate different results depending on the number of integration
sites. Where there is a single potential integration site in cells
used to generate the library, the library will be a library of
clones containing donor DNA integrated at the single fixed locus.
All clones of the library therefore contain the binder genes at the
same position in the cellular DNA. Alternatively where there are
multiple potential integration sites, the library may be a library
of clones containing donor DNA integrated at multiple and/or
different fixed loci. Preferably, each clone of a library contains
donor DNA integrated at a first and/or a second fixed locus. For
example a library may comprise clones in which donor DNA is
integrated at a first fixed locus, clones in which donor DNA is
integrated at a second fixed locus, and clones in which donor DNA
is integrated at both the first and second fixed loci. In preferred
embodiments there are only one or two fixed loci in the clones in a
library, although it is possible to integrate donor DNA at multiple
loci if desired for particular applications. Therefore in some
libraries each clone may contain donor DNA integrated at any one or
more of several fixed loci, e.g., three, four, five or six fixed
loci.
[0202] For libraries containing binder subunits integrated at
separate sites, clones of the library may contain DNA encoding a
first binder subunit integrated at a first fixed locus and DNA
encoding a second binder subunit integrated at a second fixed
locus, wherein the clones express multimeric binders comprising the
first and second subunits.
[0203] Uniform transcription. Relative levels of transcription of
the binders between different clones of the library is kept within
controlled limits due to donor DNA being integrated at a controlled
number of loci, and at the same locus in the different clones
(fixed locus). Relatively uniform transcription of binder genes
leads to comparable levels of expression of binders on or from
clones in a library. Binders displayed on the surface of cells of
the library may be identical to (having the same amino acid
sequence as) other binders displayed on the same cell. The library
may consist of clones of cells which each display a single member
of the repertoire of binders, or of clones displaying a plurality
of members of the repertoire of binders per cell. Alternatively a
library may comprise some clones that display a single member of
the repertoire of binders, and some clones that display a plurality
of members (e.g., two) of the repertoire of binders. Preferably
clones of a library express one or two members of the repertoire of
binders.
[0204] For example, a library of eukaryotic cell clones according
to the present invention may express a repertoire of at least
10.sup.3, 10.sup.4, 10.sup.5 10.sup.6, 10.sup.7, 10.sup.8 or
10.sup.9 different binders, e.g., IgG, Fab, scFv or scFv-Fc
antibody fragments, each cell containing donor DNA integrated at a
fixed locus in the cellular DNA. The donor DNA encodes the binder
and may further comprise a genetic element for selection of cells
into which the donor DNA is integrated at the fixed locus. Cells of
the library may contain DNA encoding an exogenous site-specific
nuclease.
[0205] These and other features of libraries according to the
present invention are further described elsewhere herein.
[0206] The present invention extends to the library either in pure
form, as a population of library clones in the absence of other
eukaryotic cells, or mixed with other eukaryotic cells. Other cells
may be eukaryotic cells of the same type (e.g., the same cell line)
or different cells. Further advantages may be obtained by combining
two or more libraries according to the present invention, or
combining a library according to the invention with a second
library or second population of cells, either to facilitate or
broaden screening or for other uses as are described herein or
which will be apparent to the skilled person.
[0207] A library according to the invention, one or more clones
obtained from the library, or host cells into which DNA encoding a
binder from the library has been introduced, may be provided in a
cell culture medium. The cells may be cultured and then
concentrated to form a cell pellet for convenient transport or
storage.
[0208] Libraries will usually be provided in vitro. The library may
be in a container such as a cell culture flask containing cells of
the library suspended in a culture medium, or a container
comprising a pellet or concentrated suspension of eukaryotic cells
comprising the library. The library may constitute at least 75%,
80%, 85% or 90% of the eukaryotic cells in the container.
BRIEF DESCRIPTION OF THE DRAWINGS
[0209] Embodiments of the invention will now be described in more
detail, with reference to the accompanying drawings, which are as
follows:
[0210] FIG. 1. Vector for expression of IgG formatted antibodies
[0211] a. pDUAL D1.3, a dual promoter expression vector for IgG
secretion (in in "pCMV/myc/ER" vector backbone) [0212] b.
pINT3-D1.3, a dual promoter expression vector for IgG secretion (in
"pSF-CMV-f1-Pac1" vector backbone) [0213] c. pCMV/myc/ER vector
backbone. ECoR1 site precedes CMV promoter. BstB1 and BstZ171 sites
flank the SV40 poly A sites. [0214] d. pSF-CMV-f1-Pac1 vector
backbone (Oxford Genetics) [0215] e. synthetic gene with exon
encoding PDGFR transmembrane Region.TM. and exon causing secretion
(sec). Solid arrows represent Rox recombination sites.
[0216] FIG. 2. Sequence of pD1 (SEQ ID NO: 1, 2, 3, 4 & 5): a
dual promoter antibody expression cassette for surface
expression.
Features:
[0217] pEF promoter 13-1180 BM40 leader 1193-1249
Humanised D1.3 VL 1250-1578
[0218] Human C kappa 1577-1891BGH poly A 1916-2130 CMV promoter
2146-2734 Mouse VH leader with intron 32832-3414
Humanised D1.3 VH 3419-3769
[0219] Optimised human IgG2 CH1-CH3
[0220] FIG. 3. Construction of AAVS Donor plasmid (pD2)
a. Representation of the human AAVS locus. Exon 1 and Exon 2 of the
AAVS locus (encoding protein phosphatase 1, regulatory subunit 12C,
PPP1R12C) are separated by an intron of 4428 bp. Splicing is in
frame "0" i.e. splicing occurs between 2 intact codons from each
exon. TALENs and CRISPR/Cas9 constructs are available to cleave
within this intron. Hatched blocks represent the regions to the
left and right of this cleavage site that are used within vector
constructs to drive homologous recombination into this locus (AAVS
homology arm left "Left HA" and AAVS homology arm right ("right
HA"). b. Representation of the antibody encoding donor plasmid pD2.
Left and right homology arms are shown at the ends of the construct
representation. A synthetic Blasticidin gene is preceded by a
splice acceptor which creates an "in-frame" fusion with AAVS exon
1. Also shown is the antibody expression cassette consisting of a
D1.3 light chain and a D1.3 IgG2 heavy chain driven by pEF and CMV
promoters respectively. c. Sequence of donor construct pD1-huD1.3
(SEQ ID NO: 6, 7 & 8). AAVS homology arms are shown underlined
and emboldened. For brevity antibody cassette (previously shown in
FIG. 2) is not shown in detail. Restriction sites used in clone are
shown emboldened. The sequence of the plasmid backbone is shown up
to the ampicillin resistance gene.
[0221] FIG. 4. Expression of IgG on cell surface
a, c. Analysis was focused on viable cells using forward scatter
and staining in the FL3 channel (a, c). Cells positive for staining
in the FL3 channel (representing non-viable cells which took up
7-AAD) were excluded. All cells were transfected with pD2-D1.3 in
absence (a, b) or presence (c.d) of the AAVS TALENs and were
stained with anti-Fc antibody.
[0222] FIG. 5. Antibody binding of antigen on cell surface. Viable
cells were selected on the basis of Forward Scatter and occlusion
of 7AAD (a). Cells were incubated with fluorescently labelled hen
egg lysozyme (b).
[0223] FIG. 6. Effect of TALEN-directed genomic cleavage on
integration of blasticidin resistance gene. Figure shows number of
colonies under the conditions described.
[0224] FIG. 7. Analysis of integration of pD2-D1.3 donor plasmid
into AAVS locus. Following transfection cells were selected in
Blasticidin and genomic DNA was prepared. Samples 1-9 benefitted
from addition of AAVS-directed TALE nucleases, samples 10-11 were
from clones arising from cell transfected in the absence of TALE
nuclease. Genomic DNA analysis carried out by PCR as described in
text. [0225] a. Verification from 5' end of integration site.
[0226] b. Verification from 3' end of integration site.
[0227] FIG. 8. Construction of the scFv-Fc expression vector pD6
[0228] a. Antibodies formatted as scFv are cloned into the
Nco1/Not1 sites to create an "in-frame" fusion with the human Fc
region of IgG2. [0229] b. Sequence of pD6 from the Nco1 to Pme1
sites (SEQ ID NO: 9, 10 &11).
[0230] FIG. 9. Selection of binders from cell surface scFv-Fc
library (from selected phage populations). Flow cytometry analysis
is shown of cells with:
a-c. integrated anti-CD229 sFv-Fc population from 2 rounds of phage
display selection on CD229 d. f integrated
anti-.beta.-galactosidase sFv-Fc population from 1 round of phage
display selection on .beta.-galactosidase .beta.-galR1 cells) e.
integrated anti-.beta.-galactosidase sFv-Fc population from 2
rounds of phage display selection on .beta.-galactosidase Sample
(a) shows unstained cells and the rest were stained with human
anti-Fc-phycoerythrin (in FL2) and 100 nM appropriate biotinylated
antigen/streptavidin FITC (in FL1). Cells were analysed after 13
days (a, b, d, e). Examples c and f show cells stained after 20
days and the marked region shows cells collected by flow cytometry
h. .beta.-galR1 cells selected by flow cytometry (FIG. 6f) were
grown for 22 days and re-analysed for scFv-Fc expression and
antigen binding (using 100 nM antigen). g. show the unstained
equivalent. j. shows unsorted .beta.-galR1 cells from the original
population (as in d) which had been grown for 42 days after
transfection (j). Unlabelled cells of each population are shown for
comparison (g, i)
[0231] FIG. 10. Mammalian display and sorting of IgG formatted
library.
A population of antibodies were selected on .beta.-galactosidase
using 1 or 2 rounds phage display, reformatted as IgG and targeted
via nuclease-directed integration into the AAVS locus of HEK293
cells. Panels a, b show cells derived from the round 1 phage
population either a, unsorted (after 38 days growth) or b, sorted
by flow cytometry and grown for 19 days. Panels c, d show cells
derived from round 2 phage population either c, unsorted (after 38
days growth or d, sorted and grown for 19 days.
[0232] FIG. 11. Construction of large naive scFv-Fc library and
selection of binders Cells from the naive scFv-Fc library were
stained with 500 nM biotinylated antigen and streptavidin-FITC
along with phycoerythrin-labelled anti-Fc antibody as before.
Region shows cells which were selected by flow sorting. Samples
were labelled with biotinylated: [0233] a. CD28 [0234] b.
.beta.-galactosidase [0235] c. Thyroglobulin [0236] d. EphB4
[0237] FIG. 12. Targeting vector to introduce intron containing
"multiple landing" sites for comparison of integration methods.
[0238] a. Intermediate GFP expression plasmid (pD3) [0239] b.
AAVS1_directed targeting vector (pD4). "Landing site" incorporates
elements for directing integration which are FRT, lox 2272, I-SceI
meganuclease and GFP TALEN Following integration of pD4 into the
AAVS locus, multiple recombination or nuclease cleavage sites are
present within the genome. The incoming pD5 plasmid (FIG. 15) has
left and right homology arms equivalent to the sequence present on
either side of the "landing site" to drive antibody insertion by
homologous recombination.
[0240] FIG. 13. Sequence of pD4 (SEQ ID NO: 12, 13, 14, 15, 16, 17
& 18.).
5 Sequence features include: AAVS Left homology 19-822 FRT site
832-879, Lox 2272 site 884-917, I-Sce1meganuclease site 933-950 GFP
left TALEN binding 954-968, GFP right TALEN binding 984-997
T2A 1041-1103
GFP 1104-1949
[0241] PGK promoter 2178-2691 Puromycin delta thymidine kinase
2706-4307 loxP 4634-4667 AAVS right homology 4692-5528
[0242] FIG. 14. Verification of integration of "multiple landing
site" intron in clone 6F. Following transfection cells were
selected in puromycin and genomic DNA was prepared. Samples 1
represents the whole selected population. Sample 2 represents clone
6F, sample 3 is a clone transfected in the absence of TALENs and
sample 4 is wild-type HEK293 cells. Primers and conditions
described in text were used to verify by PCR the correct
integration at the 5' and 3' ends of the genomic insertion. The
major (correct sized) band is seen for the selected clone (6F) as
well as the selected population.
[0243] FIG. 15. Sequence of donor plasmid for integration into
Flp/GFP TALEN sites (pD5) (SEQ ID NO: 19, 20 & 21). Features
include:
AAVS HA 13-233
[0244] FRT site 243-290
Lox2272 295-328
I-SceI 344-361
[0245] Blasticidin resistance 417-818
Poly A 832-1070
[0246] FIG. 16. Sequence of I-SceI meganuclease construct (SEQ ID
NO: 22 & 23)
[0247] FIG. 17. Flow cytometry analysis comparing nuclease-directed
integration using I-Scel meganuclease with recombinases.
Clone 6F cells were co-transfected with pD5-D1.3 and plasmids
encoding the indicated nuclease/recombinase. Cells were selected
with blasticidin and analysed 13 days after transfection using
biotinylated anti-human Fc antibodies and streptavidin
phycoerythrin. Percentage positive cells are indicated (also
summarized in Table 5) a. Non-transfected, b Donor only c. I-Scel,
d, eGFP TALEN, e. Cre, f. Flp recombinase (encoded by pOG44
plasmid).
[0248] FIG. 18. Nuclease-directed integration drives homologous
recombination and "non-homologous end joining" (NHEJ).
a. Representation of structure of plasmid pD5 used to target the
multiple "landing site" within the intron of "clone 6F cells
showing position of primer J48. The "landing site" in this plasmid
incorporates a FRT site, a lox2272 site and an I-SceI meganuclease
site (but no GFP TALEN site). b. Representation of integration site
within clone 6F (derived from pD4) showing position of primer J44.
"Landing site" incorporates elements for directing integration
which are FRT, lox 2272, I-SceI meganuclease and GFP TALEN. c.
Representation of clone 6F integration site after homologous
recombination of pD5, showing position of primers J44 and J48. d.
Representation of integration site of clone 6F after NHEJ or Flp
recombination of pD5, showing position of primers J44, J46 and
reverse primer J44. The double headed arrow indicates the "extra"
plasmid derived DNA incorporated by NHEJ or Flp-directed
integration. Note in this example the incoming plasmid DNA (pD5)
has homology arms (which direct homologous recombination but are
not required for NHEJ). These sequences are retained after
integration by NHEJ, causing a duplication of the sequence
represented within the homology arms with one pair coming from the
plasmid in this case and the other pair representing the endogenous
genomic sequences. For simplicity the plasmid encoded homology arms
are not shown, just their equivalent sequence within the genome. e.
Primers 44 and 48 were used as PCR primers for samples i-iv where
genomic DNA from cells transfected with the following
nucleases/integrases were used: i. Scel, ii. TALEN (GFP), iii. Flp
(pOG44), iv. Donor only. Molecular weight markers were "GeneRuler 1
kb ladder (New England Biolabs). Primers J44 and J48 reveal
homologous recombination has occurred producing a band of 1928 bp
(indicated by arrow) in nuclease cleaved samples i and ii. Primers
44 and 46 were used for samples v-viii where genomic DNA from the
following samples was used. v. Scel, vi. TALEN (GFP), vii. Flp
(pOG44), viii. Donor only. Primers J44 and J46 reveal that cleavage
of donor and genomic DNA by I-SceI meganuclease has resulted in
NHEJ (sample v.) producing a band of 1800 bp (indicated by arrow).
As expected a similar sized band was achieved by Flp mediated
integration (vii). NHEJ has not occurred with GFP TALEN since there
was no cleavage site in the incoming plasmid.
[0249] FIG. 19. Secretion of IgG antibodies into culture
supernatant.
a. Coomassie stained gel of protein A purified IgG from culture
supernatants. i. IgG purified from supernatant of pD2-D 1.3 cells
without transfection of Dre recombinase gene. ii. IgG purified from
supernatant of pD2-D 1.3 cells transfected with Dre recombinase
gene. b. Polyclonal ELISA of secreted antibodies. Sorted cells from
the experiment shown in FIG. 9H (originally from antibody
population cells selected by 1 round of phage display) were grown
for 7 days post sorting and the culture supernatant collected.
ELISA plates were coated with either .beta.-galactosidase (10
ug/ml) or BSA (10 ug/ml) overnight. Culture supernatants were
diluted down to 66% after mixing with a 33% volume of 6% Marvel-PBS
(this is described above as the `neat` sample). Supernatant was
also diluted 1/10 in PBS and mixed with 6% MPBS in the same manner.
Detection of bound scFv-Fc fusion was performed using anti-Human
IgG-Eu (Perkin Elmer Cat 1244-330).
[0250] FIG. 20. Preparation of DNA fragments for the conversion of
selected populations of scFv to IgG format. (a) Generation of
CL-pA-CMV-Sigp DNA insert (as depicted in FIG. 21b). PCR
amplification from plasmid pD2 with primers 2595 and 2597 and gel
purified. Lane m, Generuler 1 kb ladder (Thermo, SM031D), lane 1
C.sub.L-pA-CMV-Sigp DNA insert. (b) Generation of scFv DNA insert
was as described in Example 6. Lane m, Generuler 1 kb ladder
(Thermo, SM031D), lane 1 blank, lane 2 purified scFv, lane 3
.beta.-galactosidase round 1 output scFv population, lane 4
.beta.-galactosidase round 2 output scFv population, lane 5 CD229
round 2 output scFv population. (c) Purification of NheI and XhoI
digested "mini-circle" DNA. Ligations between NcoI/NotI digested
DNA encoding scFv (FIG. 21, insert a) and DNA encoding constant
light (CL) chain, poly A (pA), CMV promoter and signal peptide
(FIG. 21, insert b) to form "mini-circle" DNA (FIG. 21c) were spin
column purified, digested with NheI and XhoI and purified by 1%
agarose gel. Lanes are m, Generuler 1 kb ladder (Thermo, SM031D),
lane 1 .beta.-galactosidase round 1 output, lane 2
.beta.-galactosidase round 2 output, lane 3 CD229 round 2 output.
Linearised product at 2.6 kb, indicated by arrow, was excised and
purified.
[0251] FIG. 21. Schematic representation of the conversion process
from scFv to IgG format. A DNA insert (a) encoding the antibody VH
and VL domains is ligated with DNA fragment (b) encoding a constant
light (CL) chain, a polyadenylation sequence (pA) a cytomegalovirus
(CMV) promoter and a signal peptide (SigP). The joining of DNA
molecules a and b to create a non-replicative DNA "mini-circle" c
is facilitated by a "sticky-end" ligation. After ligation, the
"mini-circle" c is linearized with restriction enzymes NheI and
XhoI. Linearized product d is then purified and ligated with the
digested vector e. The vector e includes a pEF promoter and SigP
sequence upstream of the NheI site and encodes the antibody
constant heavy (CH) domains 1 to 3 downstream of the XhoI site. The
product of ligation of insert d with vector e would result in
plasmid f, which can be used to transform bacteria and growth with
a suitable selectable marker would allow the production and
purification of plasmid DNA by standard methods. Purified plasmid f
can be introduced into mammalian cells [134] for heterologous Ig
antibody expression. Alternatively DNA encoding CH1-3 in vector e,
could be replaced with DNA encoding a single CH1 domain for Fab
expression. V.sub.H and V.sub.L are antibody variable heavy and
light chain respectively. DNA encoding an elongation factor
promoter (pEF) an antibody constant light chain (CL) and constant
heavy domains 1 to 3 (CH1-3), a polyadenylation sequence (pA) a
cytomegalovirus (CMV) promoter and a signal peptide (SigP) are
depicted.
[0252] FIG. 22. Additional example of preparation of DNA fragments
required for the conversion of scFv to IgG (a) scFv inserts
generated as described in Example 14 were separated on a 1% agarose
TBE gel. Lanes 1 and 14 is a 500 bp DNA ladder starting at 500 bp.
Lanes 2 to 13 are scFv PCRs. (b) The purification of the linearised
"mini-circle" d (FIG. 21) was performed by separation on a 1%
agarose TBE gel. From left to right, the first lane is a DNA ladder
(1 kb ladder, Lifetech, 15615-024) and remaining lanes linearised
"mini-circle" d. (c) As (b) except the CMV promoter is replaced by
a P2A sequence and the DNA ladder employed was Generuler 1 kb
ladder (Thermo, SM031D).
[0253] FIG. 23. Nuclease-directed integration of binder genes using
flow electroporation systems. A 50:50 mix pD6 plasmids encoding
either an anti-FGFR1 or an FGFR2 antibody was electroporated using
a Flow electroporation system. After 13 days blasticidin selection
cells were labelled with FGFR1-Fc labelled with Dyelight-633
(FGFR1-Dy633) or FGFR2-Fc labelled with Dyelight 488 (FGFR2-Dy488).
Dot blots represent: [0254] a. Single staining FGFR2-488 (sample 1b
from Table 7) [0255] b. single staining FGFR1-633 (sample 1b from
Table 7) [0256] c. Dual staining FGFR1-633/FGFR2-488 (sample 1b
from Table 7) [0257] d. Dual staining FGFR1-633/FGFR2-488 (sample 3
from Table 7)
[0258] FIG. 24. Recovery of antibody genes after flow sorting.
A population of antibodies was selected by one round of phage
display and a mammalian display library was created by Flow
electroporation using the Maxcyte system. Cells were sorted using
either 1 nM or 10 nM antigen and mRNA isolated directly. The
antibody genes were recovered by PCR, cloned into a bacterial
expression vector and the proportion of ELISA positives determined
("1 nM output", "10 nM output"). This was compared with the
original round 1 output ("R1 phage output"). Plot shows the profile
of ELISA signals obtained with each population.
[0259] FIG. 25. pINT20 vector for expression of T Cell Receptors.
[0260] a. Representation of the dual promoter plasmid pINT20
showing AAVS homology arms, puromycin selectable gene (with region
around splice acceptor site shown below). Alpha chain (encompassing
variable alpha, mouse alpha constant-CD3) is flanked by Nhe1, Not1
and Acc65I restriction sites and is under the control of pEF
promoter. The beta chain (encompassing variable beta, mouse beta
constant-CD3) is flanked by Nco1, XhoI and hind3 sites and is under
the control of the CMV promoter. [0261] b. Sequence at the splice
acceptor and beginning of puromycin gene (SEQ ID NO: 24 & 25)
[0262] c. Sequence of T cell receptor clone c12/c2 alpha chain
construct showing Nhe1, Not1 and Acc65I restriction sites (SEQ ID
NO: 26 & 27). [0263] d. Sequence of T cell receptor clone
c12/c2 beta chain construct showing Nco1. XhoI and Hind 3
restriction sites (SEQ ID NO: 28 & 29). [0264] e. Sequence of T
cell receptor clone 4JFH alpha chain construct showing Nhe1/Not1
restriction sites (SEQ ID NO: 30 & 31). [0265] f. Sequence of T
cell receptor clone 4JFH beta chain construct showing
Nco1/XhoIrestriction sites (SEQ ID NO: 32 & 33). [0266] g.
Strategy and primer used to mutate CDR3 of c12/c2 TCR alpha chain
(SEQ ID NO: 34, 35, 36 & 37). [0267] h. Strategy and primer
used to mutate CDR3 of c12/c2 TCR beta chain (SEQ ID NO: 38, 39, 40
& 41). (N=A, C, G, T; S'.sup.2 C OR G; W=A OR T)
[0268] FIG. 26. Recognition of peptide; MHC complexes by T cell
receptors introduced into mammalian cells by nuclease-directed
integration.
TCR1 is TCR c12/c2 recognising peptide 1 (SLLMWITQV) in the form of
complex with phycoerythrin-labelled HLA-A2 (peptide 1). TCR2 is TCR
4JFH recognizing peptide 2 (ELAGIGILTV) in the form of complex with
phycoerythrin-labelled HLA-A2 (peptide 2). Sample a and c show
cells expressing TCR1 exposed to peptide 1 (a) or peptide 2 (c).
Sample b and d show cells expressing TCR2 exposed to peptide 1 (a)
or peptide 2 (c). Samples e and f show non-transfected HEK293 cells
labelled with peptide 1 (e) or peptide 2 (f). g. Plasmid encoding
TCR1 was mixed with 100 fold excess of TCR2 plasmid, introduced by
nuclease-directed integration into HEK cells. 1.15% of cells and
was labelled with peptide 1. 1.15% of cells were positive. h.
Plasmid encoding TCR2 was mixed with 100 fold excess of TCR1
plasmid, introduced by nuclease-directed integration and was
labelled with peptide 2. 0.62% of cells were labelled. Positive
cells collected by flow sorting and mRNA recovered for analysis of
specific TCR enrichment. Samples i-1 illustrate expression of a T
cell library in HEK293 cells. TCR library was introduced by Maxcyte
electroporation and selected for 11 days in puromycin I. Shows
cells labelled with an APC labeled anti-TCR antibody (y axis). j.
Shows cells labelled with phycoerythrin-labelled peptide 1:MHC (x
axis) k. Shows untransfected cells labelled with both anti-TCR
antibody and peptide 1:MHC l. Shows TCR1 library transfected cells
labelled with both anti-TCR antibody and peptide 1:MHC Samples m-n
illustrate expression of TCRs in Jurkat cells. TCR1 was delivered
by Amaxa electroporation and selected for 25 days in puromycin.
Plasmid was transfected in presence (m) or absence (n) of TALE
nuclease and was incubated with an APC labelled anti-TCR .beta.
chain antibody. Samples o-r illustrates T cell receptor activation
of the same TCR1-transfected Jurkat cells. All cells are labelled
with anti-CD69 antibody (y axis). Sample o was unstimulated and p
was stimulated for 24 hours with an anti-CD3 antibody. Samples q
and r were incubated for 24 hours with 2 ul and 6 ul respectively
of PE labeled MHC:peptide 1. All cells were also exposed to CD28
antibody.
[0269] FIG. 27. pINT21 CAR1 and pINT21 CAR2 vectors for
introduction of Chimeric Antigen Receptor (CAR) libraries into
human cells.
Representation of the single promoter plasmid pINT21 showing AAVS
homology arms, puromycin selectable gene, CMV promoter driving
fusion of binder to CD3 signalling domain. Nco1 and Not 1 sites are
sued for cloning the binder. [0270] a. pINT21 CAR1 fuses the binder
to the juxtamembrane, transmembrane and signalling domain of CD3.
[0271] b. pINT21 CAR2 fuses the binder to CD8 hinge and
transmembrane domain, 4-1BB and CD3.xi. activation domains [0272]
c. Sequence of CD3.xi. in pINT 21_CAR1 (SEQ ID NO: 42, 43 & 44)
[0273] d. Sequence of CD8, 4-1BB and CD3.xi. in pINT 21_CAR2 (SEQ
ID NO: 45 & 46) [0274] e. Sequence of FMC63 H-L (anti CD19
antibody) (SEQ ID NO: 47 & 48)
[0275] FIG. 28. Expression of scFv and alternative scaffold within
chimeric antigen receptor construct introduced into human cells by
nuclease-mediated integration.
HEK cells were transfected with anti-FGFR1 antibodies (b) or lox1
adhiron (d) and labelled with labelled FGFR1 and lox1 respectively.
As control the same antigens were incubated with non-transfected
HEK293 cells (a and c respectively). Populations from phage display
libraries selected on mesothelin and CD229 were introduced into HEK
cells by nuclease-mediated integration (f and h respectively) and
were selected in puromycin for 11 days. These cells or
untransfected HEK293 cells were incubated with labelled mesothelin
(e, f) or CD229 (g, h). e and h represent untransfected HEK293
cells.
[0276] FIG. 29. Sequence of alternative binder scaffolds for
mammalian display Libraries of different binder formats can easily
be introduced by nuclease-directed integration using vector
described herein. By example Adhiron constructs were prepared with
flanking Nco1 and Not 1 sites for introduction into CAR constructs
or Fc fusion constructs. [0277] a. Sequence of lox1 binding
Adhiron_lox1A (SEQ ID NO: 49 & 50) [0278] b. Sequence of lox1
binding Adhiron_lox1B (SEQ ID NO: 51 & 52) (Variable loops are
shown emboldened and underlined on the protein sequence). [0279] c.
Potential mutagenic primers for construction of library of binders
within loop 1 (adhiron mut1) (SEQ ID NO: 53, 55 & 56) or loop 2
(adhiron mut2) (SEQ ID NO: 54, 57 & 58), Below is a
representation of the region covered by the primers (lower strand)
showing protein translation. n represents variable number of NNS
codons giving rise to different loop lengths. [0280] d. Sequence of
trypsin binding knottin MCoTI-II with flanking Nco1 and Not1 sites
allowing knottin expression within vectors described herein.
Sequence of first loop is underlined SEQ ID NO: 59 & 60).
[0281] e. Strategy for creation of library of knottin mutants. In
this example loop 1 is replaced by 10 randomised amino acids. In
this example VNS codons are introduced (V=A, C or G) providing 24
codons encoding 17 amino acids. This sequence can be introduced
into a clone encoding MCoTI-II using standard methods (SEQ ID NO:
61, 62 & 63).
[0282] FIG. 30. Example sequences for Nuclease mediated antibody
gene insertion by ligation or microhomology-mediated end-joining
(MMEJ).
a. Sequence of pD7-SceI (nucleotides 1-120) (SEQ ID NO: 64, 65).
The sequence is as pD6 (see FIG. 3c, 8) except the AAVS left arm
between EcoR1 and Nsi1 has been replaced by the I-SceI meganuclease
recognition (bold). Also the AAVS right arm between Asc1 and Mlu1
has been replaced by an insert encoded by primers 2723 and 2734
(not shown). b. Sequence of pD7-ObLiGaRe (nucleotides 1-120) (SEQ
ID NO: 66, 67). The sequence is as pD6 (see FIG. 3c, 8) except the
AAVS left arm between EcoR1 and Nsi1 has been replaced by the AAVS
TALE right and left arm recognitions sites. Also the AAVS right arm
between Asc1 and Mlu1 has been replaced by an insert encoded by
primers 2723 and 2734 (not shown).
[0283] FIG. 31. Nuclease directed integration of binders into the
ROSA 26 locus. [0284] a. Shows sequence of left homology arm up to
the beginning of the puromycin gene showing primers and restriction
sites mentioned in example 22 (SEQ ID NO: 68). [0285] b. Sequence
of right homology arm for nuclease directed integration into the
ROSA 26 locus showing primers and restriction sites mentioned in
example 22 (SEQ ID NO: 69).
EXAMPLES
Example 1. Construction of Vectors for Expression of IgG Formatted
Antibodies
[0286] To effect genetic selections of binders (e.g. antibody,
protein or peptide) it is necessary to introduce a gene encoding
this binder and to drive expression of this gene from an exogenous
promoter, or by directing integration of the transgene downstream
of a promoter pre-existing in the cellular DNA, e.g., an endogenous
promoter. Antibodies represent the most commonly used class of
binders and they can be formatted for expression in different
forms. In examples below, we describe expression of a single gene
format where a scFv is fused to a Fc domain (scFv-Fc). We also
exemplify expression of antibodies formatted as human IgG2
molecules. To express IgG or FAb formatted antibodies in producer
cells such as higher eukaryotes, it is necessary to express the
separate heavy and light chains. This can be done by introducing
separate plasmids encoding each chain or by introducing them on a
single plasmid. Within a single plasmid the 2 chains can be
expressed from a multi-cistronic single mRNA. Expression of
distinct proteins from a single message requires elements such as
an Internal Ribosome Entry (IRE) sequences which enables
translation to initiate at a secondary downstream location.
Alternatively, sequence elements promoting stalling/re-initation of
translation such as viral 2A sequences could be used [119].
[0287] Alternatively, multiple distinct proteins can be expressed
from a single plasmid using multiple promoters. FIGS. 1a and 1b
show the organisation of 2 similar expression cassettes within
different vector backbones (pDUAL and pINT3) which were developed
for expression of secreted IgG formatted antibodies. These
expression cassettes were created using a combination of gene
synthesis and polymerase chain reaction amplification of standard
elements such as promoters and poly A sequences. First separate
plasmids were created within pCMV/myc/ER (FIG. 1c, Life
Technologies) for expression of antibody heavy chain
(pBIOCAM1-NewNot) and light chain (pBIOCAM2-pEF). The elements from
pBIOCAM2-pEF (including pEF promoter, light chain gene and poly A
site) were cloned into pBIOCAM1-NewNot) to create pDUAL. The
examples shown include VH and VL domains from a humanised
anti-lysozyme antibody called D1.3 [120] and are referred to as
pDUAL-D1.3 and pINT3-D1.3. The elements of pDUAL D1.3 represented
in FIG. 1a are present between the EcoR1 and BGH polyA site of the
plasmid backbone from pCMV/myc/ER (Life Technologies Cat V82320
FIG. 1c).
[0288] In a similar way separate light chain and heavy chain
cassettes were introduced into pSF-pEF (Oxford Genetics OG43) and
pSF-CMV--F1-Pac1 (Oxford Genetics OG111) respectively to create
pINT1 and pINT2. These were combined by cloning the light chain
cassette (including pEF promoter, light chain gene and poly A site)
upstream of the CMV promoter in pINT2, to create pINT3. The
elements of pINT3-D1.3 represented in FIG. 1b are cloned between
the first Bgl2 and the Sbf1 represented within the plasmid
pSF-CMV--F1-Pac1 (FIG. 1d, Oxford Genetics OG111).
[0289] The immediate early promoter of cytomegalovirus (CMV
promoter) is a powerful promoter and was used to drive expression
of heavy chains. pDUAL D1.3 also incorporates an adenovirus 2
tripartite leader (TPL) and enhanced major late promoter (enh MLP)
immediately downstream of the CMV promoter [121]. Elongation
factor-1 alpha protein is ubiquitously and abundantly expressed in
most eukaryotic cells and its promoter (pEF promoter) is commonly
used for driving transgene expression [122]. In pDUAL-D 1.3 and
pINT3-D1.3 the pEF promoter is used to drive antibody light chain
expression. The polyadenylation sites originating in bovine growth
hormone (BGH polyA) is present at the end of each expression
cassette.
[0290] Secretion of the separate heavy and light chains in the
endoplasmic reticulum (and ultimately culture supernatant) is
directed by 2 different leader sequences. Light chain secretion is
directed by a BM40 leader sequence [123]. This is followed by Nhe1
and Not1 cloning sites which allow in-frame cloning of VL genes
which are in turn fused to a human C kappa gene. Secretion of the
heavy chain is directed by a leader split by an intron originating
from a mouse VH gene (as found in pCMV/myc/ER). The leader is
followed by Nco1 and XhoI sites allowing in frame cloning of
antibody VH genes followed by a codon optimised IgG2 gene. The VL
and VH genes of the humanised D1.3 antibody [120] were cloned into
the Nhe1/Not1 and Nco1/XhoI sites respectively within pDUAL-D1.3
and pINT3-D1.3.
[0291] Membrane anchored versions of these plasmids were created
for mammalian display. Plasmid pD1 was created by digesting
pDUAL-D1.3 with Bsu36I (which cuts in CH3 domain of the IgG2 heavy
chain gene) and with BstZ171, which cuts in the backbone after the
SV40 poly A region of the neomycin resistance cassette (FIG. 1c).
This therefore removes most of the CH3 domain and the entire
neomycin expression cassette. The CH3 domain is replaced by a
synthetic insert with compatible Bsu36I and BstZ171 ends
(represented in FIG. 1e). The synthetic insert was designed to
replace the stop codon at the end of the antibody CH3 domain with a
splice donor and intron which causes splicing of the CH3 terminus
to an exon encoding the human PDGF receptor transmembrane domain
[84] the first 5 intracellular residues, a stop codon and an
additional splice donor. This is followed by an additional intron
and splice acceptor followed by a codon for single amino acid then
a stop codon (FIG. 1e). The 2 synthetic introns which flank the
exon encoding the transmembrane domain were designed with ROX
recognition sites located within them. ROX sites are recognized by
Dre recombinase causing recombination between DNA containing these
sites [88]. Inclusion of 2 ROX sites flanking the transmembrane
domain-encoding exon creates the potential to remove this exon by
the transfection of a gene encoding Rox recombinase. This would be
anticipated to create a secreted antibody product.
[0292] FIG. 2 shows the sequence of the resulting dual promoter
antibody expression plasmid expressing a humanised D1.3
anti-lysozyme antibody (hereafter referred to as pD1-D1.3 (SEQ ID
NO: 1). Anti-lysozyme binding specificity is incorporated through
inclusion of VH and VL sequences from D1.3 [120] between Nco/XhoI
and Nhe1/Not1 restriction sites respectively. The sequence is shown
from the ECoR1 site to the BstZ171. The sequences beyond the ECoR1
and BstZ171 sites are from the vector backbone as represented in
FIG. 1c.
Example 2. Construction of Vector (pD2) for Targeting an Antibody
Cassette to the AAVS Locus
[0293] Cleavage within the genome using site-specific nucleases
facilitates the insertion of heterologous DNA through homologous
recombination or non-homologous end joining (NHEJ). Human HEK293
cells were cleaved with nucleases targeting the first intron of the
protein phosphatase 1, regulatory subunit 12C (PPP1R12C) gene. This
locus was identified as a common integration site of
adeno-associated virus and is referred to as the AAVS site (FIG.
3a). The AAVS site is considered a "safe harbour" locus for
insertion and expression of heterologous genes in human cells
[124].
[0294] Following site-specific cleavage within the genome it is
possible to promote integration of a protein expressing cassette
using homologous recombination. To do this it is necessary to flank
the expression cassette with regions homologous to the sequences
found on either side of the genomic cleavage site. To direct
integration into the AAVS locus, an 804 bp section of the AAVS
locus 5' to the intended cleavage site, was PCR amplified to create
an EcoR1 and an Mfe 1 site at the 5' and 3' end respectively. This
product, representing the left homology arm for targeting the
antibody cassette, was cloned into the EcoR1 site of pD1 recreating
the EcoR1 site at the 5' end. For the right homology arm an 836 bp
section of the AAVS locus, 3' of the cleavage site, was PCR
amplified to create Bstz171 sites at each terminus and this was
cloned into the Bstz171 of pD1. The construct is represented in
FIG. 3b and the sequence of the resulting construct (pD2) is shown
in FIG. 3c.
[0295] During cloning of the AAVS left homology arm Nsi1 and Pac1
restriction sites were also inserted at the 3' end. These sites
were subsequently used to clone a synthetic intron followed by a
blasticidin gene with an accompanying poly A site. The blasticidin
gene lacks a promoter but is preceded by a splice acceptor site
that creates an in-frame fusion with the upstream exon from the
AAVS locus (FIG. 3a, b). Integration into the AAVS locus causes
expression of the promoter-less blasticidin gene. The sequence of
this final construct, called pD2, is shown in FIG. 3c.
[0296] The sequence of the antibody cassette, encompassing the pEF
promoter, D1.3 light chain, poly A region, CMV promoter, D1.3 heavy
chain, alternative splice sites and poly A site, is shown in FIG.
2. To avoid duplication this sequence is represented in FIG. 3c as
a block labelled "D1.3 ANTIBODY EXPRESSION CASSETTE".
Example 3. AAVS TALEN-Directed Integration of IgG Construct for
Cell Surface Antibody Expression and Antigen Binding
[0297] HEK293F cells (Life Technologies), grown in Freestyle medium
were transfected with pD2-D1.3 DNA in the presence or absence of an
AAVS directed TALEN vector pair. An AAVS TALEN pair ("AAVS
original") was previously described [125] and recognises the
sequence:
TABLE-US-00001 LEFT TALEN: (SEQ ID NO: 70) 5' (T)CCCCTCCACCCCACAGT
(SEQ ID NO: 71) Spacer 5' GGGGCCACTAGGGAC Right TALEN: (SEQ ID NO:
72) complement of 5' AGGATTGGTGACAGAAAA (SEQ ID NO: 73) (i.e. 5'
TTTTCTGTCACCAATCCT
[0298] An alternative, more efficient AAVS targeted TALEN pair was
identified and used in later experiments (pZT-AAVS1 L1 TALE-N and
pZT-AAVS1 R1 TALE, Cat No GE601A-1 System Biosciences). This pair,
which recognises the same site (but not the first "T" residue shown
in brackets above), are referred to as the "AAVS-SBI" TALEN
pair.
[0299] Cells were seeded at 0.5.times.10.sup.6 cells/ml and
transfected next day at 10.sup.6 cells/ml using DNA:polyethylene
imine (PolyPlus) added at a ratio of 1:2 (w/w). Cells were
transfected with 0.6 .mu.g/ml of pD2 and were co-transfected with
either pcDNA3.0 as a control (0.6 .mu.g/ml) or the combined left
and right "original AAVS" TALEN plasmids (0.3 .mu.g each/ml). pD3
which expresses EGFP from the CMV promoter (see below) was included
in the experiment as a transfection control and showed 35%
transfection efficiency. Cells were selected in suspension culture
using Freestyle medium (Life Technology) supplemented with 5 ug/ml
blasticidin.
[0300] To determine whether antibody expression had occurred on the
cell surface, cells were stained with an anti-human Fc antibody
according to the following protocol:
1. 16 days after transfection, 0.5-1.times.10.sup.6 cells from the
populations selected with Blasticidin were centrifuged for 2
minutes (200-300.times.g) at 4.degree. C. 2. Wash cells with 1 ml
wash buffer (0.1% BSA in PBS Gibco #10010) and spin cells for 2
minutes (200-300.times.g) at 4.degree. C. 3. Resuspend cells in 100
.mu.l staining buffer (1% BSA in PBS) and add 5-10 ul of
fluorochrome--conjugated antibodies. Antibodies were
phycoerythrin-labelled anti-human IgG Fc (clone HP6017, Cat. No.
409304, Biolegend) or phycoerythrin-labelled mouse IgG2a, K isotype
control (Cat. No. 400214, Biolegend). Incubate for >30 min at
4.degree. C. in the dark. 4. Wash twice with lml wash buffer and
resuspend in 500 ul wash buffer. 5. Add 5 ul of cell viability
staining solution (#00-6993-50 eBioscience) containing 50 ug/ml
7-amino-actinomycin D (7-AAD) to identify dead cells. 6. Cells were
analysed on a (Beckton Dickinson FACS II) flow cytometer.
[0301] FIG. 4 shows that there was a significantly higher
population of antibody expressing cells when pD2-D1.3 is
transfected in the presence of the AAVS targeted TALEN with 86%
positive compared with pD2-D1.3 alone with 1.5% positive.
[0302] The functionality of the surface expressed anti-lysozyme
antibody was determined by assessing binding to labelled antigen.
Hen egg lysozyme (Sigma: L6876) was labelled using Lightning-Link
Rapid conjugation system (Dylight 488, Innova Biosciences:
322-0010) as follows:
1. Add 10 ul LL-Rapid Modifier reagent to 100 ul lysozyme (200 ug
dissolved in 100 ul PBS) and mix gently. 2. Add the mix to
Lightning-Link.RTM. Rapid mix and resuspend gently by pipetting up
and down. 3. Incubate the mix for 15-30 minutes in the dark at room
temperature. 4. Add 10 ul LL-Rapid Quencher reagent to the reaction
and mix gently. 5. Store at 4.degree. C. Final concentration of
lysosyme-Dy488 is 1.6 .mu.g/l. 6. Use 6 ul lysosyme-Dy488
(.about.10 ug) per staining. 7. Staining, washing and flow
cytometry was as described above.
[0303] Analysis shows that 86% of cells transfected with pD2-huD1.3
bound labelled HEL (as judged by the M1 gate) compared with 0.29%
for un-transfected cells (FIG. 5).
Example 4. Site-Specific Nucleases (AAVS Directed TALENs) Enhance
Donor DNA Integration
[0304] Transfected cells were also plated out and selected with
blasticidin to determine the number of cells in which expression of
the promoterless blasticidin gene was activated. 24 hours after
transfection cells were plated at 0.25.times.10.sup.6 cells/10 cm
petri dish (tissue culture treated) and were grown in 10% foetal
bovine serum (10270-10.sup.6, Gibco) and 1% Minimal Essential
medium non-essential amino acid (MEM_NEAA #11140-035 Life
Technologies). 5 ug/ml blasticidin was added after another 24 hours
and medium was changed every 2 days. After 9 days cells which did
not receive pD2 plasmid were all dead. After 12 days plates were
stained with 2% methylene blue (in 50% methanol). Colony density
was too high for accurate quantitation but showed an increased
number of blasticidin resistant colonies in the presence of the
AAVS TALENs suggesting targeted integration into the AAVS locus. A
reduced amount of DNA was introduced for more accurate
quantitation.
[0305] Transfections were carried out as described earlier using
either 50, 200 or 400 ng pD2-D1.3/10.sup.6 cells in presence or
absence of the AAVS TALENs (0.3 ug/ml of each TALEN where present,
Table 1A). The total DNA input was adjusted to 1.2 ug DNA per
10.sup.6 cells with control plasmid pcDNA3.0. After 24 hrs of
transfection, 0.25.times.10.sup.6 cells were plated in a 10 cm dish
and 7.5 ug/ml blasticidin was added after 24 hrs of plating. 10
days after blasticidin selection the colonies are stained with 2%
methylene blue (in 50% methanol). Results are shown in FIG. 6 and
summarised in Table 1A. This shows that co-transfection of DNA
encoding AAVS-directed TALENs increases the number of blasticidin
resistant colonies achieved by approximately 10 fold.
[0306] A comparison was carried out between "AAVS original" and the
"AAVS SBI" TALEN pairs targeting the AAVS locus. Table 1B shows an
increased number of blasticidin resistant colonies using the "AAVS
SBI" TALEN pair.
TABLE-US-00002 TABLE 1 Quantitation of blasticidin-resistant
colonies from transfection of pD2-D1.3 A. pD2-D1.3 donor With AAVS
Without AAVS Enzyme plasmid (ng/10.sup.6 cells) TALEN TALEN AAVS
original 50 319 32 AAVS original 200 526 41 AAVS original 400 686
75 B. Without AAVS TALEN pD2-D1.3 donor With AAVS (Control Enzyme
plasmid (ng/10.sup.6 cells) TALEN pcDNA3.0) AAVS original 300 1420
111 AAVS original 1000 1080 127 AAVS original 3000 560 70 AAVS-SBI
300 2800 111 AAVS-SBI 1000 1630 127 AAVS-SBI 3000 870 70
[0307] Here we have compared the effect of TALEN nuclease addition
using either cell surface antibody expression (Example 3) or
activation of a promoter-less blasticidin gene (Example 4). The
benefit of nuclease-directed integration is more obvious when
measuring antibody expression compared to effect on
blasticidin-resistant colonies. One likely explanation is that the
levels of expression required to effect survival in the presence of
blasticidin may be significantly less than the expression levels
required to detect IgG2 expression on the surface. Thus
misincorporation/splicing of the promoter-less blasticidin gene
could lead to a low level expression of the blasticidin resistance
gene causing a higher background of blasticidin resistant colonies
in the absence of significant antibody expression.
Example 5. Determination of Accuracy of Integration Using AAVS
TALEN
[0308] To investigate the accuracy of integration, colonies were
picked from the experiment in Example 4/Table 1A (from duplicate,
unstained plates), expanded and genomic DNA from these cells was
used as template in PCR. For preparation of genomic DNA, cells were
harvested and were re-suspended in 700 .mu.L of lysis buffer (10 mM
Tris.Cl, pH=8.0, 50 mM EDTA, 200 mM NaCl, 0.5% SDS, supplemented
with 0.5 mg/mL of Proteinase K (added just before lysis). The cell
re-suspension in lysis buffer was then transferred to a microfuge
tube and kept at 60.degree. C. for about 18 hours. Next day, 700
.mu.L of isopropanol was added to the lysate in order to
precipitate genomic DNA. The microfuge tube was spun at 13,000 rpm
for 20 minutes. The genomic DNA pellet was then washed with 70%
ethanol, and spun at 13,000 rpm for another 10 minutes. After
spinning, the supernatant was carefully separated taking care not
to touch the genomic DNA pellet. The genomic DNA pellet was then
re-suspended in 100 .mu.L buffer containing 10 mM Tris (pH 8.0),
and 1 mM EDTA and kept at 60.degree. C. for 30 minutes keeping the
lid open in order to get rid of traces of ethanol. To this 100
.mu.L solution, RNAse A was added (final concentration of 20 g/mL),
and incubated at 60.degree. C. for about one hour. Genomic DNA
concentration was measured using nanodrop spectrophotometer
(Nanodrop).
[0309] To identify correct integration, PCR primers were designed
which hybridise in the AAVS genomic locus beyond the left and right
homology arms. These were paired up with insert specific primers.
At the 5' end the primers were:
TABLE-US-00003 AAVS-Left-arm-junction-PCR-Forw (9625) (SEQ ID NO:
74) 5' CCGGAACTCTGCCCTCTAAC BSD_Junction PCR-rev (9626): (SEQ ID
NO: 75) 5' TAGCCACAGAATAGTCTTCGGAG
[0310] These give a product of 1.1 kb where correct integration
occurs. 8/9 clones arising from AAVS directed integration gave a
band of correct size (FIG. 7a, b). 2 blasticidin resistant clones
derived without TALENs did not give a product (FIG. 11a) indicative
of random integration. At the 3' end primers were:
TABLE-US-00004 Donor_plasmid_seq_PDGFRTM-2 Forw (SEQ ID NO: 76) 5'
ACACGCAGGAGGCCATCGTGG AAVS1_right arm_junction_PCR_rev (SEQ ID NO:
77) 5' TCCTGGGATACCCCGAAGAG
[0311] These give a product of 1.5 kb with correct integration. 7/9
clones arising from AAVS directed integration gave a band of
correct size. 2 blasticidin resistant clones derived without TALENs
did not give a product (FIG. 11b). Thus the majority of blasticidin
resistant cells arise from correct integration into the AAVS locus
whereas blasticidin resistant colonies arising in the absence of
TALENs are not correctly integrated.
Example 6. Construction of an scFv Display Library from a Selected
Population from Phage Display and Selection Via Mammalian
Display
[0312] scFv formatted soluble antibodies have previously been
expressed from the vector pBIOCAM5-3F where expression is driven by
the CMV promoter and the vector provides a C-terminal fusion
partner, consisting of human Fc, His6 and 3.times.FLAG, to the
antibody gene [105, 126]. This was modified to create the vector
pBIOCAM5newNot where the Not1 site was embedded within the Fc
region of the antibody (as shown in FIG. 8). This was used as a
starting point to create the vector pD6 (FIG. 8) for expression of
scFv-Fc fusions tethered to the cell surface. Primers (2598 and
2619) were designed to allow amplification of the CMV
promoter-scFv-Fc expression cassette from pBIOCAM5newNotPrimer 2598
hybridises upstream of the CMV promoter and places a Pac1 site
(bold text) at the end.
TABLE-US-00005 2598: (SEQ ID NO: 78)
TTTTTTTTAATTAAGATTATTGACTAGTTATTAATAGTAATCAATTACGG GGTC
[0313] Primer 2619 hybridises near the end of the Fc domain and
introduces a slice donor site and Pme1 site (bold text) at the
beginning of the intron.
TABLE-US-00006 2619: (SEQ ID NO: 79)
TTTTTTGTTTAAACTTACCTTGGATCCCTTGCCGGGGCTCAGGCTCAGGG AC
[0314] The resulting PCR product is compatible with the Pac1 and
Pme1 sites of pD2 (FIG. 3).
Digestion of pD2 with Pac1 and Pme1 removes: pEF
promoter-leader--light chain-CMV promoter-leader-heavy chain
Cloning of the Pac1/Pme1 cut PCR product insets: CMV
promoter-leader-Nco1/Not1 sites-human Fc.
[0315] Cloning in this way positions the scFv-Fc cassette
appropriately for splicing to the downstream trans-membrane domain
previously described for IgG presentation on the cell surface in
pD2. The final vector pD6 is shown in FIG. 8, the sequence ofD6
from Nco1 to Pme1 sites is shown.
[0316] Phage display selections were carried out using the
McCafferty phage display library [7] using beta-galactosidase
(Rockland, Cat B000-17) and CD229 (R and D Systems, Cat 898-CD-050)
as antigens. Methods for selection and sub-cloning were essentially
as described previously [6, 7, 118, 127]. scFv genes from
populations arising from one or two rounds of selection on
beta-galactosidase and two rounds of selection on CD229 were
recovered by PCR. Primers M13Leadseq hybridises within the
bacterial leader sequence preceding the scFv gene and Notmycseq
hybridises in the myc tag following the scFv gene in the phage
display vector [127].
TABLE-US-00007 M13Leadseq (SEQ ID NO: 80) AAA TTA TTA TTC GCA ATT
CCT TTG GTT GTT CCT Notmycseq (SEQ ID NO: 81) GGC CCC ATT CAG ATC
CTC TTC TGA GAT GAG
[0317] PCR product was digested with Nco1 and Not1, the digested
insert was gel purified. The digested product was ligated into the
Nco1 and Not 1 sites of the bacterial expression plasmid pSANG10-3F
and antibody expressed and screened as described [127]. After 2
rounds of selection on beta-galactosidase and CD229, 40/190 (21%)
and 35/190 (18%) clones were found to be positive by ELISA.
[0318] 550 ng of Nco/Not cut insert was also ligated into the Nco1
and Not 1 sites of pD6 (2.4 .mu.g) to create a construct expressing
a fusion between the scFv and the Fc region of human IgG2. Ligated
DNA was transformed into electro-competent NEB5alpha cells (New
England Biolabs, Cat C2989) which generated a library size of
2-3.times.10.sup.7 clones for each population. DNA was prepared and
was co-transfected into 100 mls HEK293 cells grown in Freestyle
medium as described above using 0.3 .mu.g donor DNA (pD6-library)
per 10.sup.6 cells. Cells were co-transfected with 0.5 .mu.g each
of "AAVS-SBI" TALENs (pZT-AAVS1 L1 TALE-N and pZT-AAVS1 R1 TALE,
Cat No GE601A-1 System Biosciences).
[0319] 24 hours after transfection the volume of the bulk culture
was doubled and 24 hours later blasticidin (10 .mu.g/ml) was added.
Medium was refreshed every 3-4 days and after 6 days blasticidin
concentration was increased to 20 .mu.g/ml.
[0320] In order to determine the library size, 20,000 cells were
plated in a 10 cm petri dish (tissue culture treated) 24 hours
after transfection and were grown in 10% foetal bovine serum
(10270-106, Gibco) and 1% Minimal Essential medium non-essential
amino acid (MEM_NEAA #11140-035 Life Technologies). 10 .mu.g/ml
blasticidin was added after another 24 hours and medium was changed
every 2 days. After 8 days plates were stained with 2% methylene
blue (in 50% methanol). Results are shown in Table 2. This shows
that libraries of around 3.times.10.sup.6 clones (representing 3%
of transfected cells) were obtained for the 3 populations.
TABLE-US-00008 TABLE 2 Determination of scFv-Fc library size. No
colonies/ No colonies/ Sample 20,000 cells 10.sup.6 cells Library
size .beta.-galactosidase Rd1 546 27,300 2.7 .times. 10.sup.6
.beta.-galactosidase Rd2 654 32,700 3.2 .times. 10.sup.6 CD229 Rd2
556 27,800 2.8 .times. 10.sup.6
[0321] The protocol for labelling and flow sorting
10-20.times.10.sup.6 cells is shown below. Initial analysis was
carried out 13 days post-transfection using only 10.sup.6
cells/sample and with reduced incubation volumes (reagent volumes
that are 1/10th of those shown).
[0322] FIG. 9 shows that at 13 days post-transfection at least
43-46% of cells express scFv-Fc fusion on the cell surface and this
can be detected using either FITC or phycoerythrin-labelled anti-Fc
antibodies. Binding of biotinylated beta-galactosidase is also
detected within this population using either FITC or
phycoerythrin-labelled streptavidin. Using streptavidin-FITC 11.8%
and 39% of the cell were positive for both antibody expression and
antigen binding using libraries derived from output populations
arising from 1 or 2 rounds of phage display selection respectively.
For CD229 derived from 2 rounds of phage display, 66% of cells were
positive for scFv-Fc and 24% of these were positive for CD229
binding (15% of the total population).
[0323] At 20 days after transfection cells were labelled according
to the protocol below (using biotinylated
antigen/phycoerythrin-labelled streptavidin and FITC-labelled anti
human Fc).
1. Harvest, wash and adjust cells in 15-20.times.10.sup.6 cells per
sample. Spin down cells at 250 g for 4', RT, wash cells with 1 ml
PBS+0.1% BSA (4.degree. C.), spin down cells at 250 g for 4', RT,
resuspend in 1 ml PBS+1% BSA 2. Add biotinylated antigen to a final
conc. 100 nM and incubate 30' at 4.degree. C. 3. Wash the cells 2
times lml of 0.1% BSA by centrifugation at 1500 rpm for 5 minutes
4. Add either:
[0324] 10 .mu.l of FITC-labelled streptavidin (1 .mu.g/ml, Sigma
Cat S3762) and 20 .mu.l of phycoerythrin-labelled anti human Fc
(200 .mu.g/ml, BioLegend Cat. 409304), or:
[0325] 20 .mu.l phycoerythrin-labelled streptavidin (200 .mu.g/ml,
Biolegend Cat 405203) and 20 .mu.l of FITC-labelled anti human Fc
(200 .mu.g/ml, Biolegend Cat 409310) PBS+1% BSA, for 15 at
4.degree. C. in the dark
5. Wash the cells 2 times 1 ml of 0.1% BSA by centrifugation at
1500 rpm for 5 minutes 6. resuspend them in 500 .mu.l ice cold
PBS+1% BSA 7. Add 20 ul of 7AAD/vial for viability staining
[0326] For sorting cells were gated on the basis of cell size,
granularity, pulse width and viability (via 7-AAD staining, forward
scatter and side scatter. Results are shown in FIGS. 9c and f In
total 10 million cells were sorted and 3.1% and 7% of doubly
positive cells were collected for libraries derived from output
populations arising from 2 rounds of CD229 (CD229 R2) selection and
1 round of .beta.-galactosidase selection (.beta.-galR1)
respectively.
[0327] Selected cells from the .beta.-galR1-derived cells were
grown for a further 20 days and re-analysed (FIG. 9h). This shows
that the majority of cells now express scFv-Fc and bind
.beta.-galactosidase. This figure also shows that the proportion of
double positive cells within the unselected population has not
diminished 42 days after transfection (FIG. 9k).
[0328] Genomic DNA was prepared from 150,000-10.sup.6 sorted cells.
Genomic DNA was prepared using method described earlier or using a
GenElute mammalian genomic DNA miniprep kit (Sigma G1N10).
scFv genes were PCR amplified from genomic DNA using the following
primers:
TABLE-US-00009 2623 (SEQ ID NO: 82) TAAAGTAGGCGGTCTTGAGACG 2624
(SEQ ID NO: 83) GAAGGTGCTGTTGAACTGTTCC
[0329] PCR reactions were carried out using Phusion polymerase (NEB
Cat M0532S) in manufacturer's buffer containing 0.3 uM of each
primer and 3% DMSO. 100-1000 ng of genomic DNA was used as template
in a 50 ul reaction. 30 cycles were carried out at 98.degree. C.
for 10 secs, 55.degree. C. for 25 secs, 72.degree. C. for 45 secs.
This gave a product of 1.4 kB which was digested with Nco1 and
Not1. A band of approximately 750-800 bp was generated and gel
purified before cloning into pSANG10. Ligated DNA was transformed
into BL21 cells (Edge Bio Ultra BL21 (DE3) competent cells, Cat.
45363). In this way scFv fragments derived from the sorted
population can be expressed in bacteria as described previously [7,
127].
[0330] As an alternative to isolating the antibody gene and
expressing in an alternative vector/host combination, it is
possible to derive secreted antibody directly from the selected
cells either following single cell cloning or using a sorted
population to generate a polyclonal antibody mix. To exemplify this
culture supernatant was taken from sorted cells (from .beta.-galR1
cells) after 7 days in culture. This was shown to be positive in
ELISA using plates coated with (3-galactosidase (see Example 13 and
FIG. 19b).
Example 7. Construction and Selection from an IgG Display Library
from a Selected Population from Phage Display
[0331] DNA fragments encoding scFv, representing the round 1 and 2
antibody phage display outputs of selections against
.beta.-galactosidase and CD229, were generated as described in
Example 6. The scFv populations were converted to IgG format
according to Example 14 and as detailed in the method below.
[0332] A DNA insert encoding the human kappa light chain constant
domain (CL), polyadenylation sequence (pA), CMV promoter and signal
peptide from murine V.sub.H chain (represented between the Not1 and
Nco1 sites of pD2 shown in FIG. 21b) was PCR amplified from plasmid
pD2 with primers 2595 (GAGGGCTCTGGCAGCTAGC) (SEQ ID NO: 84) and
2597 (TCGAGACTGTGACGAGGCTG) (SEQ ID NO: 85). PCR reactions were
carried out using KOD hot start polymerase (Novagen Cat 71086-4) in
manufacturer's buffer containing 0.25 .mu.M of each primer. 10 ng
of pD2 plasmid DNA was used as template in a 50 ul reaction. 25
cycles were carried out at 98.degree. C. for 10 secs, 55.degree. C.
for 25 secs, 72.degree. C. for 40 secs. This gave a product of 1.8
kB which was digested with Nco1 and Not1 and gel purified (FIG. 20a
represented as CL-pA-CMV-SigP insert in FIG. 21b).
[0333] DNA fragments encoding scFv, representing the round 1 and 2
antibody phage display outputs of selections against
.beta.-galactosidase and CD229, were generated as described in
Example 6. FIG. 20b shows scFv populations selected against
.beta.-galactosidase and CD229 separated by 1% agarose gel
electrophoresis.
[0334] Ligations between scFv insert and CL-pA-CMV-SigP insert were
performed by incubating NcoI/NotI digested scFv insert (1 .mu.g)
with NcoI/NotI digested CL-pA-CMV-SigP insert (1 .mu.g) with T4 DNA
ligase (1.5 al, Roche, 10-481-220-001) in manufacturer's buffer in
a total volume of 40 .mu.l to form the "mini-circle" depicted in
FIG. 21c. Ligations were incubated at 16.degree. C. for 16 hours,
purified by spin column, digested with NheI and XhoI and the 2.6 kb
product (depicted in FIG. 21d) purified by electrophoretic
separation on 1% agarose gel (FIG. 20c).
[0335] The DNA insert depicted in FIG. 21d encoding
V.sub.L-C.sub.L-pA-CMV-SigP-V.sub.H (0.5 .mu.g) was ligated with
NheI/XhoI digested, gel purified vector pD2 (0.7 .mu.g) (FIG. 21e)
with T4 DNA ligase (1.5 .mu.l, Roche, 10-481-220-001) in
manufacturer's buffer in a total volume of 40 .mu.l to produce the
targeting vector depicted in FIG. 21f. This encodes populations of
antibodies formatted as IgGs, originating from first or second
round antibody phage display selections to (3-galactosidase or
CD229. Ligations were incubated at 16.degree. C. for 16 hours,
purified by spin column and eluted with HPLC grade water.
[0336] Ligated DNA was transformed into electro-competent NEB5alpha
cells (New England Biolabs, Cat C2989) which generated a library
size of 1-4.times.10.sup.5 clones for each population. DNA was
prepared and was co-transfected into 100 mls HEK293 cells grown in
Freestyle medium as described above using 0.3 Lg donor DNA
(pD6-library) per 10.sup.6 cells. Cells were co-transfected with
0.5 .mu.g each of"AAVS-SBI" TALENs (pZT-AAVS1 L1 TALE-N and
pZT-AAVS1 R1 TALE, Cat No GE601A-1 System Biosciences).
[0337] 24 hours after transfection the volume of the bulk culture
was doubled and 24 hours later blasticidin (10 .mu.g/ml) was added.
Medium was refreshed every 3-4 days and after 6 days blasticidin
concentration was increased to 20 ag/ml.
[0338] In order to determine the library size, 250,000 cells were
plated in a 10 cm petri dish (tissue culture treated) 24 hours
after transfection and were grown in 10% foetal bovine serum
(10270-106, Gibco) and 1% Minimal Essential medium non-essential
amino acid (MEM_NEAA #11140-035 Life Technologies). 10 .mu.g/ml
blasticidin was added after another 24 hours and medium was changed
every 2 days. After 8 days plates were stained with 2% methylene
blue (in 50% methanol). Results are shown in Table 3. This shows
that libraries of between 5.times.10.sup.5 and 9.times.10.sup.5
clones (representing 0.5% to 0.9% of transfected cells) were
obtained for the 3 populations.
TABLE-US-00010 TABLE 3 Determination of size of mammalian display
libraries formatted as IgG. No. colonies/ No. colonies/ Library
Sample 0.25 .times. 10.sup.6 cells 10.sup.6 cells size
(.times.10.sup.5) .beta.-galactosidase Rd1 1337 5348 5.3
.beta.-galactosidase Rd2 1972 7888 7.9 CD229 Rd2 2175 8700 8.7
[0339] The bulk of the population of cells transfected with the
outputs from 1 or 2 rounds of selection on .beta.-galactosidase
were selected in blasticidin containing medium as described
earlier. After 19 days 10-20.times.10.sup.6 cells were labelled and
flow sorting carried out as described in example 6. Sorted cells
were grown for 17 days and re-analysed by flow cytometry (FIG. 10).
This showed that the majority of cells were now double positive for
IgG expression and binding to .beta.-galactosidase.
[0340] Genomic DNA was prepared from the sorted cells and DNA
encoding the IgG insert was isolated by PCR. The IgG-encoding
insert was amplified using KOD polymerase (Merck, cat. no.
71086-3), with annealing temperature of 60.degree. C. and employing
30 cycles. Manufacturer provided buffer with 5% DMSO was used with
0.3 .mu.M of primers 2597 (SEQ ID NO: 54) and 2598 (SEQ ID NO: 47).
The product of desired size was gel purified. The gel purified
product was then used for nested PCR using KOD polymerase (Merck,
cat. no. 71086-3) in manufacturer's buffer with 5% DMSO using 0.3
.mu.M of primer 2625 (SEQ ID NO: 55) in combination with either
primer 1999 (SEQ ID NO: 56) (for R1 sample), or 2595 (SEQ ID NO:
53) (for 4R1 and 5R1), using annealing temperature of 60.degree. C.
employing 30 cycles. These nested PCR products were gel purified
and subjected to double digestion with NheI-HF (NEB, cat. no.
R3131S) and XhoI (NEB, cat. no. R0146S) in order to ligate them
with similarly double digested pINT3 (FIG. 1) for expression of
soluble IgG formatted binders. Primer sequences are:
TABLE-US-00011 2597: (SEQ ID NO: 85) AGGGGTTTTATGCGATGGAGTT 2598:
(SEQ ID NO: 78) GTTACAGGTGTAGGTCTGGGTG 2625: (SEQ ID NO: 86)
CCTTGGTGCTGGCACTCGA 1999: (SEQ ID NO: 87)
AAAAAGCAGGCTACCATGAGGGCCTGGATCTTCTTTCTCC 2595: (SEQ ID NO: 84)
GAGGGCTCTGGCAGCTAGC
Example 8. Construction and Selection from a Naive scFv Library
[0341] Schofield et al. [7] describe the construction of a phage
display library (McCafferty library") wherein antibody genes from
the B-lymphocytes of a number of human donors were first cloned
into an "intermediate library" before re-cloning into the final
functional phage display library. This same intermediated library
and the same methodology was used to generate a new library (IONTAS
library) of 4.times.10.sup.10 clones. Plasmid DNA was prepared from
this library taking care to ensure sufficient representation of the
library within the bacterial inoculation. A number of PCR reactions
were set up using a total of 2 ug of DNA template. The PCR product
was digested with Nco1 and Not 1 gel purified and ligated as
described in Example 6. 9.3 ug of pD6 and 0.93 ug of PCR insert
were ligated overnight, the ligation reaction cleaned up using
phenol chloroform extraction and the DNA electroporated into
DHSalpha cells as described previously [7]. As a result a library
of 2.4.times.10.sup.8 clones was created within the scFv-Fc display
vector. DNA was prepared from this "naive library" cloned in pD6
and transfected into 1 litre of HEK293F cells (Life Technologies)
grown in Freestyle medium (as described above). 0.3 ug pD6-library
DNA, 0.5 ug of each "AAVS-SBI" TALEN pair. 24 hours after
transfection the culture volume was doubled and 48 hour after
transfection Blasticidin selection was commenced as described
above. The library size was determined by plating aliquots of the
culture 24 hours after transformation and selecting on blasticidin
as described above. A library of 0.9.times.10.sup.7 clones was
created.
[0342] A number of antigens were biotinylated using EZ-Link
Sulfo-NHS-LC-Biotin kit (Pierce Cat. No. 21327) according to
manufacturer's instructions. Antigens were bovine thyroglobulin
(Calbiochem Cat. 609310), human CD28-Fc chimera (R and D Systems,
Cat 342-CD-200) and mouse EphB4-Fc chimera (R and D Systems, Cat.
446-B4-200). Biotinylated .beta.-galactosidase (Rockland Cat.
B000-17) was also used.
[0343] Transfected cells were selected in blasticidin in liquid
culture as described above for 17 days. Cells were harvested,
washed and adjusted to 15-20.times.10.sup.6 cells per sample. Cells
were prepared as described and biotinylated antigen added to a
concentration of 500 nM. Labelling and flow sorting a as described
above. Using control cells incubated with only the
phycoerythrin-labelled anti-Fc antibody a "gate" was created which
included 0.05% of these cells. Using the same gate for labelled
cells between 0.28-0.51% of cells were included (FIG. 11). These
were collected and grown to allow additional rounds of sorting and
amplification of scFv genes from the naive library.
Example 9. Creation of a Cell Line with Multiple "Landing Sites" to
Compare Nuclease and Recombinase-Directed Approaches to Genomic
Integration
[0344] To allow comparison of integration methods based on either
genomic cleavage or recombinase-mediated integration an
AAVS-directed targeting vector (pD4) was constructed which
introduces an intron with multiple "landing sites" (Example 3).
These include an FRT site recognized by Flp recombinase and a pair
of a lox2272/loxP sites recognized by Cre recombinase. To allow
targeted cleavage, pD4 also includes a sequence from GFP for which
a TALEN pair have been designed [128] and an I-Sce 1 meganuclease
site to allow endonuclease-directed integration. A compatible
incoming donor plasmid was constructed (pD5) with appropriate
recognition sites such that nuclease or recombinase directed
integration causes activation of a promoter-less blasticidin gene
and integration an antibody expression cassette.
[0345] The organisation of the plasmid and the sequence of pD4 is
shown in FIG. 12b. An intermediate plasmid pD3 was first created
which encompasses a GFP gene under the control of the CMV promoter
followed by a puromycin/Thymidine kinase gene fusion under the
control of the PGK promoter (FIG. 12a). This was created by
digesting pBIOCAM1-newNot with Sac1 (at the end of the CMV
promoter) and BstB1 (between the Neo gene and the poly A site, FIG.
1a). This removed the neomycin expression cassette and allows
replacement with a synthetic insert encompassing an enhanced Green
Fluorescent Protein (EGFP) gene under the control of the CMV
promoter. This GFP construct was fused at the C terminus to
residues 422-461 of a mutated mouse ornithine decarboxylase PEST
sequence. This PEST sequence is incorporated in plasmid
pZsGreenl-DR (Clontech) and has been shown to reduce the half-life
of the fused GFP to 1 hour. A cassette encoding a PGK promoter,
Puromycin/Thymidine kinase gene fusion (Puro deltaTK) and polyA
cassette was excised from the plasmid pFLEXIBLE [129] using Xmnl
and Fsel and was cloned into Smal and Fsel sites present in the
original synthetic insert. The resultant plasmid (called pD3)
encodes a CMV-driven GFP gene and a puromycin resistance gene
driven from a PGK promoter.
[0346] To create the final targeting vector pD4, the CMV promoter
was removed and AAVS homology arms were inserted. An 850 bp section
of the AAVS locus was PCR amplified to create an AAVS left homology
arm flanked by an EcoR1 at the 5' end and an Mre1 at the 3' end.
This was cloned into the ECoR1/Mre1 site of pD3 thereby removing
the CMV promoter. An Nsi1 site was also incorporated at the 3' end
of this AAVS left homology arm. The neighbouring Mre1 and Nsi1
sites were used to introduce a synthetic fragment fusing an intron
to the EGFP gene as shown in FIG. 13. The synthetic intron
preceding the EGFP gene incorporates:
a FRT recognition site for Flp recombinase a lox 2272 recombination
site an I-SceI meganuclease site GFP TALEN recognition site a T2A
ribosomal stalling sequence [130]
[0347] The AAVS right homology arm was generated by PCR to create a
Hpa1 and BstZ171 sites at 5' and 3' ends. This fragment was cloned
into the Hpa1 and BstZ171 sites of pD3. The resulting plasmid pD4,
encodes a puromycin resistance cassette ("Puro deltaTK") and can be
used to introduce a "landing sites" into the AAVS locus
incorporating various nuclease and recombinase sites for
comparison. The sequence of pD4 is shown in FIG. 13 (SEQ ID NO: 5,
SEQ ID NO: 6 and SEQ ID NO: 7). The AAVS left and right targeting
arms are shown in detail in FIG. 3 and are therefore abbreviated in
FIG. 13.
[0348] The intron introduced into pD4 contains a TALEN recognition
sites originating from GFP [128]. The eGFP directed TALEN pair
(eGFP-TALEN-18-Left and eGFP-TALEN-18-right) recognise the sequence
shown below (all sequences and primers are presented in a 5' to 3'
direction) where capitals represent the recognition site of left
and right TALENs and the lower case shows the spacer sequence. The
right hand TALEN recognises the complement of the sequence shown.
The first base pair is equivalent of the minus16 position of the
sequence relative to the initiating ATG sequence of GFP (shown bold
in the spacer).
TABLE-US-00012 (SEQ ID NO: 88)
TCCACCGGTCGCCAccatggtgagcaagggCGAGGAGCTGTTCA
[0349] The plasmid pD4 also incorporates an I-SceI meganuclease
site and an FRT site recognised by Flp recombinase. Finally pD4
incorporates lox 2272 and loxP (which are mutually incompatible)
flanking the GFP and puromycin expression cassettes. Incorporation
of these same 2 loxP sites flanking the donor plasmid (pD5 below)
affords an opportunity to substitute the integrated cassette
(including the PGK puro delta TK cassette) replacing it with an
incoming cassette driving expression of blasticidin and antibodies
via recombinase mediated cassette exchange.
Creation of a Cell Line by Transfection of pD4.
[0350] HEK293F cells were resuspended at 10.sup.6 cells/ml and
DNA:polyethylene imine (PolyPlus) added at a ratio of 1:2 (w/w).
Cells were transfected with 0.6 .mu.g/ml of pD4 and were
co-transfected with either the "original AAVS" TALEN pair or
pcDNA3.0 as a control (0.6 g/ml). pD3 which expresses EGFP from the
CMV promoter was included in the experiment as a transfection
control and showed 35% transfection efficiency. After 24 hours
transfected cells were plated at 0.5.times.10.sup.6 cells/10 cm
petri dish (tissue culture treated) and were grown in 10% foetal
bovine serum (10270-106, Gibco) and 1% Minimal Essential medium
non-essential amino acid (MEM_NEAA #11140-035 Life Technologies). 5
.mu.g/ml puromycin was added after another 24 hours and medium was
changed every 2 days. After 5 days untransfected cells or cells
transfected with pD3 only were dead. After 12 days there were
approximately 200 colonies on cells transfected with pD4 only and
approximately 400 colonies on cells transfected with pD4 and the
AAVS TALEN pair.
[0351] The puromycin resistant population arising from transfection
with pD4 and the AAVS TALEN pair was analysed for correct
integration. In addition a single colony was picked from this
population (clone 6F) and compared with a colony from the puromycin
resistant population arising from pD4 transfection in the absence
of the AAVS TALEN pair. To identify correct integration, PCR
primers were designed which hybridise in the AAVS genomic locus
beyond the left and right homology arms. These were paired up with
insert specific primers. At the 5' end the primers were:
TABLE-US-00013 AAVS1_HA-L_Nested_Forw1 (SEQ ID NO: 89)
GTGCCCTTGCTGTGCCGCCGGAACTCTGCCCTC EGFP_Synthetic_gene_Rev_Assembly
(SEQ ID NO: 90) TTCACGTCGCCGTCCAGCTCGAC Purotk_seq_fow2 (SEQ ID NO:
91) TCCATACCGACGATCTGCGAC AAVS1_Right_arm_Junction_PCR_Rev (SEQ ID
NO: 77) TCCTGGGATACCCCGAAGAG
[0352] FIG. 14 shows that clone 6F and the population are correct
at both left and right ends but the clone picked from the non-AAVS
directed population is negative. Thus PCR analysis indicates that
the accuracy of integration of the donor cassette is greater when
directed by AAVS TALEN cleavage of genomic DNA.
[0353] pD4 introduces a promoter-less, in-frame GFP gene driven
from the AAVS promoter. Flow cytometry of the puromycin resistant
population showed an absence of GFP expression. This failure to
express could be due to the combination of a short half-life (from
the murine ornithine decarboxylase PEST sequence element) combined
with reduced expression arising from the use of the T2A promoter.
In fact it was found that addition of the T2A element in front of a
promoterless blasticidin element (as described for pD2) reduced the
number of blasticidin resistant colonies by 4 fold. Despite the
absence of GFP expression, the integration of multiple landing
sites still affords an opportunity for comparison of
recombinase-directed versus DNA cleavage directed genomic
integration.
Example 10. Construction of a Vector for Inserting an Antibody
Cassette (pD5) into the "Multiple Landing" Site
[0354] Following introduction of the "multiple landing site" intron
into the AAVS locus, it is possible to introduce an antibody
cassette via nuclease-directed or recombinase-directed means. To do
this a donor plasmid pD5 was created where the expression cassette
is flanked by left and right homology arms which are equivalent to
the sequences flanking the GFP TALEN cleavage site introduced into
pD4. pD5 does not itself incorporate an intact GFP TALEN
recognition site and integration is driven by homologous
recombination. Homology-directed integration of the donor plasmid
will lead to introduction of a blasticidin gene which lacks a
promoter but is preceded by a splice acceptor site that creates an
in-frame fusion with the upstream exon from the AAVS locus as
described earlier. Integration into the AAVS locus will cause
expression of the promoter-less blasticidin gene. The inserted
cassette also encodes an IgG formatted antibody heavy and light
chains under the control of pEF and CMV promoters respectively as
described above. pD5 incorporates an I-SceI meganuclease site which
can lead to cleavage of the incoming donor affording an opportunity
for NHEJ (see Example 12). An FRT site is also incorporated into
the donor plasmid pD5 allowing recombinase-directed incorporation
of the promoter-less blasticidin gene and antibody expression
cassettes at the same locus. As discussed above Cre recombinase
will act on the loxP sites in donor and genomic DNA to direct
recombinase-mediated cassette exchange.
[0355] The sequence of pD5 is shown in FIG. 15. The sequence at the
5' end of the GFP TALEN site is from the AAVS locus. A 267 bp
section of the AAVS locus upstream of the TALEN cleavage site was
generated by PCR. Primers were used which created an EcoR1 and an
Mfe 1 site at the 5' and 3' end and the product was cloned into the
ECoR1 site of pD1-D1.3. The EcoR1 site is re-created at the 5' end.
During cloning of the left homology arm an Nsi1 and Pac1 was also
inserted at the 3' end. A right homology arm, incorporating
approximately 700 bp equivalent to the sequence 3' of the GFP TALEN
was created by PCR assembly. PCR primers introduce BstZ171 sites at
5' and at the 3' end of the assembled fragment and this was cloned
into the BstZ171 site of pD1-D1.3. The PCR primers also introduced
a Hpa1 site at the 5' end.
[0356] A PCR fragment encompassing the intron (which incorporates
recognition sites for GFP TALEN, I-Scelendonuclease, Flp
recombinase and Cre recombinase), a splice acceptor region, a
Blasticidin resistance gene and poly A site (described above) was
created with Nsi1 site at the 5' end and a Pac1 site at the 3' end.
This was cloned into the Nsi1 and Pac1 site of the plasmid
described above to create pD5-D1.3 (sequence shown in FIG. 15 and
plasmid structure shown in FIG. 18a).
Example 11 Comparison of Nuclease-Directed and Flp-Directed
Integration of an Antibody Expression Cassette
[0357] The Flp-In system which has previously been used for
recombinase-mediated integration of antibody expression cassettes
[18] uses a mutant Flp recombinase (in the plasmid pOG44) which
possesses only 10% of the activity at 37.degree. C. of the native
Flp recombinase [19]. A variant of Flp recombinase (Flpe) with
better thermostability and activity at 37.degree. C. than wild type
has been identified [19, 20]. This was further improved by codon
optimization to create Flpo [131] encoded within plasmid
cCAGGS-Flpo (Genebridges Cat. A203). The effect of both variants of
Flp recombinase (encoded within pOG44 and cCAGGS-Flpo) was
compared. Recombination directed by Cre recombinase was also
examined by co-transfecting cells with a plasmid which encodes Cre
recombinase [132] (pCAGGS-Cre, Genebridges Cat. A204). In each
vector the recombinase is expressed under the control of the
chicken-1-actin promoter and a CMV immediate early enhancer. An
SV40 Large T nuclear localization sequence is used for nuclear
localisation [20]. In the original vectors (cCAGGS-Flpo and
pCAGGS-Cre) recombinase expression was linked to a puromycin
resistance gene by an internal ribosomal entry site (IRES) which
was removed using standard molecular biology techniques.
[0358] An experiment was carried out to compare the efficiencies of
genomic cleavage-directed versus recombinase directed integration
of an antibody cassette. The outcome was assessed in 2 ways:
1. Measuring the number of blasticidin-resistant colonies arising
from integration of a promoter-less blasticidin gene 2. Assessing
the extent of antibody expression achieved by the different
approaches.
[0359] As described in Example 9, the recognition sites for Cre
recombinase (lox2272 and loxP) and Flp recombinase (FRT) were
previously integrated into the AAVS locus within clone 6F. In
addition recognition sites for a GFP TALEN pair and for the
meganuclease I-SceI are also present within the same intron. The
donor plasmid pD5-D 1.3 carries the same recognition sites (apart
from GFP TALEN) within an intron upstream of a promoter-less
blasticidin gene. Correct integration will lead to activation of
the blasticidin gene. pD5-D1.3 also encodes an IgG formatted D1.3
antibody gene which will be expressed on the cell surface.
[0360] Co-transfection of pD5-D1.3 with pOG44 or pCAGGS-Flpo
(encoding 2 variants of Flp recombinase) should result in
integration of the entire pD5 plasmid into the FRT site of clone
6F. The donor plasmid pD5-D1.3 also has lox2272 site within the
synthetic intron upstream of the blasticidin gene and a loxP site
at the end of the antibody expression cassette. Under the action of
Cre recombinase expressed from pCAGGS-Cre, recombinase-mediated
cassette exchange should result in the integration of the
blasticidin and antibody expression cassettes into the lox2722 and
loxP sites within clone 6F.
[0361] The efficiency of vector integration using
recombinase-directed approaches with genomic cleavage-directed
approaches was compared using a pair of TALENs (eGFP-TALEN-18-Left
and eGFP-TALEN-18-right) directed towards a sequence from GFP
(Reyon et al., 2012). In the case of GFP TALENs the element between
the left and right homology arms will be integrated following
genomic cleavage by TALENs.
[0362] To allow comparison with I-SceI meganuclease a codon
optimised gene encoding I-Sce1 was constructed (FIG. 16). This gene
has an N terminal HA epitope tag/SV40 nuclear localisation signal
(NLS) at the N terminus and is flanked by Nco1 and Xba1 sites at
the 5' and 3' termini. The gene was cloned into the vector
pSF-CMV-F1-Pac1 (Oxford Genetics OG111) where expression is driven
from the CMV promoter.
[0363] Transfections were carried out using the clone 6F with a
correctly integrated "multiple landing site". Cells were suspended
at 10.sup.6/ml and transfected with 50 ng of pD5-D1.3 donor
plasmid/10.sup.6 cells along with enzyme encoding plasmids (Table
4A).
[0364] After 24 hours transfected cells were plated at
0.5.times.10.sup.6 cells/10 cm petri dish (tissue culture treated)
and were grown in 10% foetal bovine serum (10270-106, Gibco) and 1%
Minimal Essential medium non-essential amino acid (MEM_NEAA
#11140-035 Life Technologies). 5 ug/ml blasticidin was added after
another 24 hours and medium was changed every 2 days. After 12 days
plates were stained with 2% methylene blue (in 50% methanol) and
colony numbers counted (Table 2). In a direct comparison between
Flp recombinase, Cre recombinase and TALEN, the greatest number of
colonies were obtained through use of the GFP TALEN where there was
a 9-fold increase compared with "donor only" (Table 4A). It also
appears that the use of the optimised Flpo gene actually resulted
in a reduction of the number of blasticidin resistant colonies
compared to "donor only" control, presumably through toxicity of
the enhanced activity Flp recombinase. There was also an increase
in colony number using pCAGGS-Cre compared to the donor only
control.
[0365] A second experiment was carried out comparing GFP TALEN with
both enhanced Flp (from cCAGGS-Flpo) as well as the low activity
Flp enzyme encoded within pOG44 from the Flp-In system (as used by
Zhou et al. [17, 18, U.S. Pat. No. 7,884,054]. These were compared
with GFP TALEN and Cre recombinase (Table 4B). Cells were
transfected with the amounts DNA shown per million cells.
0.25.times.10.sup.6 cells were plated out and the number of
blasticidin resistant colonies determined as described above. Cells
were also selected for blasticidin resistance in liquid culture for
30 days before determining the proportion of cells which expressed
surface IgG (as described above). Table 4B shows that the TALEN was
superior to the other approaches in terms of number of resistant
colonies. Again the use of optimised Flp within cCAGGS-Flpo
actually caused a reduction in number of blasticidin resistant
colonies compared to "donor only" controls. Cre recombinase again
led to an increase in blasticidin colony count compared with
control whereas the Flp gene within pOG44 showed only a marginal
increase compared with control.
TABLE-US-00014 TABLE 4 Comparison of TALE nuclease-directed and
recombinase- directed integration approaches. A. pD5-D1.3 donor
Blasticidin (ng/10.sup.6 resistant colonies Enzyme plasmid Amount
cells) (colonies/10.sup.6 cells) GFP TALEN pair 0.575 .mu.g each 50
152 (304) pCAGGS-Flp.sub.o 1.15 .mu.g 50 1 (2) pCAGGS-Cre 1.15
.mu.g 50 57 (114) control 1.15 .mu.g 50 17 (34) (pCDNA3.0) B.
Blasticidin colonies per 10.sup.6 cells Sample pD5-D1.3 (percentage
Percentage 2 .mu.g each per Donor Blasticidin blasticidin positive
10.sup.6 cells .mu.g/10.sup.6 cells colonies resistant) in flow GFP
TALEN pair 0.6 270 1080 (1.1%) 95.6 cCAGGS-Flp.sub.o 0.6 35 140
(0.14% Too few cells pOG44 0.6 96 384 (0.38%) 6.4 pCAGGS-Cre 0.6
180 720 (0.72%) 4 Control 0.6 81 324 (0.32%) 37.3 (PCDNA3.0) C.
Sample 2 .mu.g each pD5-DL3 Donor Blasticidin Percentage per
10.sup.6 cells .mu.g/10.sup.6 cells colonies positive in flow GFP
TALEN 2 210 95.3 GFP TALEN 6 120 73.6 cCAGGS-F1p.sub.o 2 62 Too few
cells cCAGGS-F1p.sub.o 6 41 Too few cells pOG44 7 178 35.6 pOG44 6
63 58.7 pCAGGS-Cre 2 84 40.9 pCAGGS-Cre 6 52 Too few cells Control
2 340 65.6 (PCDNA3.0) Control 6 82 55.5 (PGDN A3.0)
[0366] With the addition of more donor DNA (Table 4C) there was an
increase in colony numbers at intermediate levels (2 .mu.g/million
cells) and a decline at higher levels across the board (6
.mu.g/million cells). None of the other samples achieved levels of
antibody display seen with the GFP TALEN directed integration.
[0367] Cells were also selected for blasticidin resistance in
liquid culture and the cells stained for antibody expression as
described above. TALEN-directed integration gave a significantly
higher proportion of antibody-positive cells compared with the
other approaches. Cells transfected with cCAGGS-Flpo and with high
concentrations of pCAGGS-Cre were not healthy and there were
insufficient numbers to carry out flow cytometry.
[0368] The comparison was extended to included I-Sce endonuclease.
A synthetic gene encoding I-SceI was synthesised (FIG. 16) and
cloned into the Nco1/Xba 1 site of pSF-CMV-f1-Pac1 (Oxford
Genetics). Cells were suspended at 10.sup.6/ml and transfected for
each ml of cells (10.sup.6 cells/ml) with 300 ng of pD5-D1.3 donor
plasmid along with plasmids encoding enzymes (1 ug/10.sup.6 cells).
Next day 0.05 ml of cells were plated and selected in blasticidin
and stained after 14 days as described. Table 5 shows that the
highest number of blasticidin resistant colonies came from I-SceI
meganuclease followed by the eGFP TALEN pair. Both Cre and Flp
recombinase (encoded within pOG44) gave numbers slightly higher
than the "donor only" control. As before transfection with the Flpe
encoding plasmids actually reduced colony numbers compared to
"donor only".
TABLE-US-00015 TABLE 5 Comparison of meganuclease-directed and
recombinase- directed integration approaches Blasticidin colonies
per Sample pD5-D1.3 10.sup.6 cells Percentage Percentage 2 .mu.g
each Donor (percentage positive positive per 10.sup.6
.mu.g/10.sup.6 Blasticidin blasticidin in flow in flow cells cells
colonies resistant) d 7 d 13 GFP TALEN pair 0.6 90 1800 27.6 55%
I-Scel 150 3000 29.9 47% meganuclease pOG44 0.6 60 1200 2.79 6.5%
pCAGGS-Cre 0.6 56 1120 2.95 6.6% cCAGGS-Flp.sub.o 0.6 4 80 5.9 Too
(low few cell nos) cells Control 40 800 3.3 4.9% (PCDNA3.0) (SBI
AAVS 251 (x2) 10040 (1%) ND ND into WT HEKs
[0369] After transfection the bulk of cells were selected for
blasticidin resistance in liquid culture and after 7 and 13 days
were stained with an anti-Fc phycoerythrin labelled antibody as
described above. FIG. 17 (summarised in Table 5) shows
significantly higher antibody expression was achieved for cells
transfected with I-SceI endonuclease and eGFP TALEN (47% and 55%
respectively) compared to "donor only" (4.9%). In contrast the
percentage of antibody positive cells when cells were
co-transfected with plasmids encoding Flp recombinase (pOG44) or
Cre recombinase were 6.6 and 6.5% respectively. The proportion of
antibody positive cells continues to increase with continued
selection in blasticidin and achieves 85-90% antibody positive in
the case of the I-SceI and EGFP TALEN transfected samples when
assayed on day 19. Thus meganucleases provide an alternative
approach to effect nuclease-directed integration of
antibody-encoding transgenes.
Example 12. Nuclease-Directed Integration of an Antibody Cassette
can Occur by Both Homologous Recombination and NHEJ
[0370] The efficiency of integration of transgenes into cellular
DNA can be enhanced by the introduction of double stranded breaks
(DSBs). Endogenous DNA repair mechanisms in eukaryotic cells
include homologous recombination non-homologous end joining (NHEJ)
and variants of these. All provide a means for introducing genes
encoding binders within a library. Homologous recombination
provides a precise join between the regions of homology and the
inserted transgene but require the provision of regions of homology
in the donor plasmid. DNA for homologous recombination can be
provided as linear or circular DNA. With NHEJ the ends of DNA are
directly re-ligated without the need for a homologous template.
This approach to DNA repair is less accurate and can lead to
insertions or deletions. NHEJ nonetheless provides a simple means
of integrating in-frame exons into intron or allows integration of
promoter:gene cassettes into the genome. Use of non-homologous
methods allows the use of donor vectors which lack homology arms
thereby simplifying the construction of donor DNA.
[0371] Clone 6F has GFP TALEN and I-SceI nuclease recognition sites
integrated into the genome and these will be cleaved when these
nucleases are provided. The donor vector pD5 does not have a GFP
TALE nuclease recognition site but has homology arms flanking the
cleavage site and so is expected to integrate by homologous
recombination only. Cleavage of genomic DNA at the neighbouring
I-SceI meganuclease will also lead to integration of the pD5
elements by homologous recombination. pD5 however also has an
I-SceI meganuclease site which can be cleaved in vivo when I-SceI
is provided. This will create a linear DNA product which can
potentially be integrated by NHEJ. As described earlier there may
even be efficiency advantages using in vivo cleavage of donor DNA
when NHEJ is used.
[0372] FIG. 18a represents the incoming pD5-D1.3 donor DNA and FIG.
18b represents the genomic locus of clone 6F cells incorporating
the "multiple landing" site. FIG. 18c represents the consequence of
homologous recombination between pD5-D1.3 (FIG. 18a) and the
multiple landing site of clone 6F (FIG. 18b). FIG. 18d in contrast
represents the consequence of NHEJ. In this case extra DNA from the
backbone of the incoming plasmid is incorporated (represented by a
double arrow). Flp mediated recombination at the "multiple landing"
site will lead to a similar product. In order to determine which
route is being used with the samples described in Example 11 (shown
in FIG. 17) genomic DNA was prepared from the blasticidin selected
population as described before. A reverse PCR primer (J44) was
designed which hybridizes to the integrated PGK promoter. This was
used in conjunction with either J48 which hybridises at the end of
the IgG protein. Primers J44 and J48 were designed to reveal
homologous recombination producing a band of 1928 bp when I-SceI is
responsible for integration (indicated by arrow in FIG. 18e).
(Potentially a band of 5131 bp could be produced by this primer
pair when NHEJ has occurred but this longer product was not visible
in the genomic PCRs of this experiment.)
[0373] Primer J46 was designed to hybridise within the
.beta.-lactamase gene within the vector backbone. Primers J44 and
J46 are anticipated to produce a band of 1800 bp when NHEJ has
occurred. A similar sized band is expected where Flp recombinase
has led to recombinase-mediated integration.
TABLE-US-00016 J44: (SEQ ID NO: 92) AAAAGCGCCTCCCCTACCCGGTAGAAT
J46: (SEQ ID NO: 93) GGCGACACGGAAATGTTGAATACTCAT J48: (SEQ ID NO:
94) CACTACACCCAGAAGTCCCTGAGCCTG
[0374] FIG. 18e clearly reveals that homologous recombination
occurs only with the samples treated with GFP TALEN and I-SceI
meganuclease ((i and ii compared with iii and iv). In contrast NHEJ
only occurs when cleavage is effected by I-SceI meganuclease (FIG.
18e v.) but not GFP TALEN (FIG. 18e vi). As expected a similar size
band is found in the sample treated with Flp recombinase (FIG. 18e
vii). Thus this experiment reveals nuclease-directed integration of
an antibody cassette can occur by both homologous recombination and
NHEJ.
Example 13. Generation of Secreted and Membrane Bound Antibody
Fragments from the Same Cell
[0375] As described above, mammalian display vectors pD2 and pD5
were constructed with an exon encoding a transmembrane domain
flanked by two ROX recognition sites recognised by Dre recombinase
[88]. In order to determine whether it was possible to convert from
a membrane bound form to a secreted form, the blasticidin resistant
population arising from transfection with pD2-D1.3/AAVS TALEN pair
was re-transfected with the plasmid encoding Dre recombinase
(pCAGGs-Dre). This was based on the plasmid pCAGGs-Dre-IRES puro
[88] which drives the Dre recombinase gene from a CAGGs promoter
(GeneBridges A205). The puromycin resistance gene was removed using
standard molecular biology techniques. After 22 days of blasticidin
selection, cells were set up at 0.5.times.10.sup.6 cells/ml and
transfected as described earlier with 0.5 .mu.g pCAGGs-Dre per
10.sup.6 cells. After 6 days supernatants were collected, antibody
purified using protein A and samples run on an SDS-PAGE gel and
stained with Coomassie blue. FIG. 19a shows that secreted antibody
was found in the supernatant even without transfection with the Dre
recombinase gene. This may arise from alternative splicing where
the exon encoding the transmembrane domain is skipped.
Alternatively antibody in the culture supernatant could arise from
cleavage of the membrane bound antibody. Transfection of Dre
recombinase increased the level of secreted antibody (FIG.
19a).
[0376] Production of secreted scFv-Fc fusion was also demonstrated
in the experiment describe in example 7 (FIG. 9h). Antibody scFv
populations selected by 1 round of phage display on 3-galactosidase
were introduced into the pD6 vector and integrate into the AAVS
locus of HEK293 cells using the AAVS TALEN. Antigen binding cells
were sorted by flow sorting and selected cells were grown for 7
days post-sorting without a change of medium to allow antibody to
accumulate. ELISA plates were coated with either
.beta.-galactosidase (10 ug/ml) or BSA (10 ug/ml) overnight.
Culture supernatants from 7 day cultures were mixed with a 50%
volume of 6% Marvel-PBS and the sample tested in triplicate. A 1/10
dilution was also tested. Detection of bound scFv-Fc fusion was
performed using anti-Human IgG-Eu (Perkin Elmer Cat 1244-330). FIG.
19b shows that antibody binding can be detected directly from
culture supernatants either neat or with a 1/10 dilution. This
illustrates that both surface display and antibody secretion can be
achieved within the same cells without additional steps. It will be
possible to derive secreted antibody directly from the selected
cells either following single cell cloning or using a sorted
population as shown here generate a polyclonal antibody mix.
Example 14. A Simple Method for Conversion of scFvs to IgG or Fab
Format
[0377] A novel method was invented to effect the conversion of
antibodies formatted as scFv to an IgG format as described in
Example 7. This conversion is a necessary process during antibody
drug discovery projects employing scFv antibody phage display
libraries where an IgG or Fab formatted antibody is required as the
final format. Current methods are tedious and involve individual
cloning of the variable heavy (V.sub.H) and variable light
(V.sub.L) chains into suitable expression vectors. Furthermore
conversion of a population of scFvs "en masse" is not possible
because the link between the V.sub.H and V.sub.L chains is lost.
This is a problem because both the V.sub.H and V.sub.L chains
contribute to antigen binding specificity. The current inability to
easily convert populations of scFv to Ig or Fab format limits the
ability to screen large numbers of antibodies in the final format
they will be used in the clinic. The ability to screen recombinant
antibodies in Ig or Fab format for target binding, cell reporter
screens and biophysical properties and function including
aggregation state is a necessary step to choose candidate antibody
drugs as clinical candidates. The greater number of antibodies
tested at this stage, in IgG or Fab format, the greater the chance
of selecting the best antibody drug candidate.
[0378] Described here is a method to convert single chain antibody
(scFv) populations to immunoglobulin (Ig) or Fab format in such a
way that the original variable heavy (V.sub.H) and variable light
(V.sub.L) chain pairings are maintained. The method allows one to
convert monoclonal, oligoclonal or polyclonal scFvs simultaneously
to Ig or Fab format. Preferably, the method proceeds via the
generation of a non-replicative "mini-circle" DNA. Preferably the
complete conversion process entails a single transformation of
bacteria such as E. coli to generate a population of bacterial
colonies each harbouring a plasmid encoding a unique Ig or Fab
formatted recombinant antibody. This is distinct from alternative
methods requiring two separate cloning and transformation steps
[117].
[0379] More broadly this aspect of the invention relates to a
method of converting a genetic construct with 3 linked genetic
elements A, B and C (represented by the V.sub.H, linker and V.sub.L
respectively in the case of an scFv) to a format where the order of
the flanking elements (A and C) are reversed, in a single cloning
step. The intermediate element could be retained but most usefully
the method permits the replacement of this intermediate element by
a new element D (to give C-D-A). In the example of conversion of a
scFv to an IgG or Fab then C is an antibody V.sub.L domain and A is
a V.sub.H domain. In this example element D encapsulate a light
chain constant domain, poly A site, promoter and leader sequence
fused to the V.sub.H (element A). In the process the product
(C-D-A) is re-cloned allowing the flanking sequences to also be
changed. In the scFv to IgG conversion example the V.sub.L element
is preceded by a promoter and leader sequence and the V.sub.H is
followed by a C.sub.H1-C.sub.H2-C.sub.H3 domain in the case of IgG
formatted antibodies and by a CH1 domain in the case of Fab
formatted antibodies. The method could be applied more broadly
where elements A and C (using above nomenclature) could represent
other genetic elements, e.g., in construction of proteins with
circularly permutation where the original N and C termini are fused
and novel internal termini are engineered.
[0380] FIG. 21 illustrates the conversion process schematically
using scFv to IgG conversion as an example. A DNA insert (a)
encoding the antibody VH and VL domains is ligated with DNA
fragment (b) encoding a constant light (CL) chain, a
polyadenylation sequence (pA), a cytomegalovirus (CMV) promoter and
a signal peptide (SigP). DNA fragment (b) could also encode any
promoter in place of the CMV promoter. Also the pA-CMV cassette
could be replaced by an internal ribosomal entry sites (IRES) [119]
or a 2A type "self-cleaving" small peptide [130, 133]. The joining
of DNA molecules (a) and (b) to create a non-replicative DNA
"mini-circle" (c) is facilitated by a "sticky-end" ligation. In
FIG. 21, Nco1 and NotI sites are employed because these were used
in the creation of the McCafferty phage display library [7] however
any suitable restriction sites could be used to create the
non-replicative "mini-circle" c. After ligation, the "mini-circle"
c is linearized with restriction enzymes NheI and XhoI, the
recognition sites of which flank the linker between the V.sub.H and
V.sub.L domains. NheI and XhoI were chosen to illustrate this
invention because they were used in the creation of the McCafferty
phage display library [7], however any suitable restriction sites
could be used.
[0381] Linearised product d is then purified and ligated with the
digested vector (e). The vector (e) includes a CMV or pEF promoter
and signal sequence upstream of the NheI site and encodes the
antibody constant heavy (C.sub.H) domains 1 to 3 downstream of the
XhoI site. The vector would also encode a bacterial origin or
replication and antibiotic resistance marker (not shown) to enable
selection and replication of the resultant plasmid DNA in bacteria.
The product of ligation of insert (d) with vector (e) would result
in plasmid f, which can be used to transform bacteria and growth
with a suitable selectable marker would allow the production and
purification of plasmid DNA by standard methods. Purified plasmid f
can be introduced into mammalian cells [134] for heterologous Ig
antibody expression. Alternatively DNA encoding CH.sub.1-3 in
vector (e), could be replaced with DNA encoding a single C.sub.H1
domain for Fab expression.
[0382] In the detailed method description below used to illustrate
this invention, the insert b contains either a CMV promoter or a
P2A peptide which enables expression of the separate antibody light
and heavy chains from a single messenger RNA (mRNA). The method is
non-obvious and was refined after several experimental attempts.
For example, initially linearisation of the DNA "mini-circle" (c)
was attempted by PCR. However this resulted in the amplification of
homo-dimer side-products, resulting in a low yield of the desired
product (d). In contrast, direct digestion of the DNA "mini-circle"
(c) provided sufficient material (d) to allow the method to be
successfully implemented. Secondly, in an attempt to prevent
undesired homo-dimer product, insert (a) was initially
dephosphorylated. However, this required careful control to prevent
"end" digestion resulting in product lacking the desired
"sticky-ends" for ligation. The optimal method does not include
dephosphorylation to maximise the proportion of ligation competent
product. Lastly, careful control of the ratios used in the ligation
of DNA inserts (a) and (b) was required to maximise the yield of
the DNA "mini-circle" (c).
1. Preparation of PCR scFv Inserts From a bacterial glycerol stock,
harbouring plasmid DNA encoding scFv scrape into 50 ul of water.
Dilute this 1 in 10. Use 5 ul of this for PCR reaction containing
forward primer pSANG10pelB (CGCTGCCCAGCCGGCCATGG SEQ ID NO: 95)
(2.5 .mu.l, 5 M), reverse primer 2097 (GATGGTGATGATGATGTGCGGATGCG
SEQ ID NO: 96), (2.5 .mu.l, 5 M), 10.times.KOD buffer (KOD hot
start kit from Merck, 71086-4), dNTPs (5 .mu.l, 2 mM), MgSO4 (2
.mu.l, 25 mM), KOD hot start polymerase (2.5 units) in a total
volume of 50 .mu.l. Cycling conditions were 94.degree. C. for 2 min
then 25 cycles of 94.degree. C. 30 sec, 54.degree. C. for 30 sec
then 72.degree. C. for 1 min. PCR clean up was performed by spin
column (Qiagen or Fermentas) and the PCR reactions eluted in 90
.mu.l. FIG. 22a shows 1 .mu.l of PCR reaction loaded on a 1%
agarose TBE gel. Purified scFv DNA (80 .mu.l, 8 .mu.g) was digested
by the addition of buffer 4 (New England Biolabs), BSA (0.1 mg/ml)
and 40 units of NcoI-HF and NotI-HF in a total volume of 100 .mu.l
and incubated for 2 hours at 37.degree. C. Inserts were purified
with a Qiagen PCR cleanup kit, eluted in 30 .mu.l and the DNA
concentration measured by measuring the absorbance at 260 nM with a
nanodrop spectrophotometer (Thermo).
2. Ligation of DNA Inserts (FIGS. 21a and b)
[0383] A ligation reaction is performed to produce the DNA
"mini-circle" (FIG. 21c). The ligation reaction contains insert b
(125 ng), scFv insert a (FIG. 21) (125 ng), 10.times. ligation
buffer (Roche T4 DNA ligase kit, 1.5 ul), T4 DNA ligase (1 unit) in
a total volume of 15 .mu.l. Incubate 1-2 hr 21.degree. C. Water (35
.mu.l) was added to the ligation mix and purified with a Qiagen PCR
cleanup kit and eluted in 30 .mu.l.
3. Digestion of DNA "Mini-Circle" (FIG. 21c) with Xho1/Nhe1
[0384] Purified ligation reaction (28 .mu.l) is digested by the
addition of buffer 4 (New England Biolabs, 3.5 .mu.l), BSA (0.1
mg/ml) and 10 units of NcoI-HF and NotI-HF in a total volume of 35
.mu.l and incubated for 2 hours at 37.degree. C. This is then
purified by separation on a 1% agarose TBE (FIG. 22b).
Alternatively FIG. 22c shows a linearised "mini-circle" containing
a P2A sequence in place of a CMV promoter. DNA band at 2.6 kb (FIG.
22b) is excised and purified with Qiagen gel extraction kit and
eluted in 30 .mu.l.
4. Ligation of Linearised DNA "Mini-Circle" d with pINT3 (XhoI/NheI
Cut) Vector and Transformation of E. coli DH5a.
[0385] A standard ligation was set-up with pINT3 cut vector (50
ng), linearised "mini-circle" d (20 ng), 10.times. ligase buffer
(Roche, 1.5 .mu.l) and 1 unit of T4 DNA ligase (NEB) in a final
volume of 15 .mu.l. Incubation was at 21.degree. C. for 2 hrs.
Transformation of E. coli DH5alpha chemically competent cells,
subcloning efficiency, (Invitrogen, cat. 18265017) was according to
the manufacturer's instructions. 80 .mu.l of chemically competent
DH5a cells were added to 6 .mu.l ligation mix, placed on ice for 1
hour and heat shocked at 42.degree. C. 1 min, ice for 2 min and
then transferred to a 14 ml polypropylene tube containing 900 .mu.l
SOC media and incubated at 37.degree. C. for 1 hour and plated on
LB amp plates.
Example 15: Construction of Large Display Libraries in Mammalian
Cells by Nuclease-Directed Integration Using Flow
Electroporation
[0386] Electroporation is an efficient way of introducing DNA, RNA
and protein into cells and electroporation flow systems allow for
efficient introduction of DNA into large numbers of mammalian
cells. For example the "MaxCyte STX Scalable Transfection System"
(Maxcyte) permits the electroporation of 10.sup.10 cells within 30
mins, creating the potential for transfecting up to 10.sup.11 cells
in a day. Cells and DNA are mixed and passed from a reservoir to an
electroporation chamber, electroporated, pumped out and the process
repeated with a fresh aliquot of cells and DNA. The same method can
be applied for introduction of DNA, RNA, protein or mixtures
thereof into cultured cells (e.g., human HEK293 cells or Jurkat
cells) or primary cells e.g. human lymphocytes [135]. Flow
electroporation has been used to efficiently introduce DNA, RNA and
protein into a large number of primary and cultured cells.
[0387] Here we exemplify the use of such a system to introduce
donor DNA, encoding antibody genes, by co-transfecting with DNA
encoding a pair of TALE nucleases targetted to the human AAVS locus
of human HEK293 cells and Jurkat cells.
[0388] The distribution of the 2 different antibody specificities
was determined by flow cytometry using fluorescently-labelled
antigen. The generation of antibodies recognising the FGF receptors
FGFR1 or FGFR2 has been described previously [105]. Clones
.alpha.-FGFR1_A and .alpha.-FGFR2_A (described therein) were cloned
into pD6 as described in example 6. In addition a population of
scFv antibodies selected from the "McCafferty" phage display
library [7] using one round of phage display on
.beta.-galactosidase (.beta.-gal) were also cloned into this vector
(as described in example 6).
[0389] HEK293 cells were centrifuged and re-suspended in a final
volume of 10.sup.8 cells/ml in the manufacturer's electroporation
buffer (Maxcyte Electroporation buffer, Thermo Fisher Scientific
Cat. No. NC0856428)). An aliquot of 4.times.10.sup.7 cells (0.4 ml)
was added to the electroporation cuvette with 100 .mu.g DNA (i.e.,
2.5 .mu.g/10.sup.6 cells). The amounts of the different components
used are shown below. Donor DNA encoding antibodies .alpha.-FGFR1_A
and .alpha.-FGFR2_A was provided as an equimolar mix with the total
amount per 10.sup.6 cells shown in Table 6 below. DNA encoding
AAVS-SBI TALENs (pZT-AAVS1 L1 and pZT-AAVS R1 Systems Bioscience
Cat. No. GE601A-1) was used as an equimolar mix with the total
amount per 10.sup.6 cells shown in Table 6 below. In samples
without added TALENs, the input DNA was brought to 2.5
.mu.g/10.sup.6 cells using control plasmid pcDNA3.0.
[0390] The percentage transfection efficiency was calculated by
counting the number of blasticidin colonies achieved for a given
input of total cells. The fold difference compared to negative
controls (i.e., no TALEN DNA added) is shown in brackets. Finally,
the number of transformed colonies achievable by running a full
cycle of the Maxcyte system, involving electroporation of 10.sup.10
cells is calculated in the last column. This represents a single
cycle of approximately 30 minutes, giving the potential to run
multiple cycles in a day. Thus, the daily output could be 5-10-fold
higher. Large scale fermentation and culture systems such as
Wavebag system (GE Healthcare) or the Celltainer system (Celltainer
Biotech) can be used to generate cells for transfection and can be
used to cultivate the resulting libraries.
TABLE-US-00017 TABLE 6 Electroporation of HEK293 cells
.alpha.FGFR1_A/ .alpha.FGFR2_A ng TALEN ng donor DNA/10.sup.6 No
clones per Sample DNA/10.sup.6 cells cells % transfection 10.sup.10
cells 1 580 1920 5.1 (51x) 5.1 .times. 10.sup.8 1b 580 640 3.3
(33x) 3.3 .times. 10.sup.8 2 580 -- 0.1 -- 0.1 .times. 10.sup.8 3
194 1920 2.7 (89x) 2.7 .times. 10.sup.8 4 194 -- 0.03 0.03 .times.
10.sup.8 5 1185 1315 5.8 (25x) 5.8 .times. 10.sup.8 6 1185 -- 0.23
0.23 .times. 10.sup.8 7 1825 675 6.1 (11x) 6.1 .times. 10.sup.8 8
1825 -- 0.57 0.57 .times. 10.sup.8 9 580 (FGFR1 1920 5.3 5.3
.times. 10.sup.8 alone) 10 580 (FGFR2 1920 5.3 5.3 .times. 10.sup.8
alone) .beta.-galactosidase ng TALEN donor DNA/ DNA/10.sup.6 No
clones per Sample 10.sup.6 cells cells % transfection 10.sup.10
cells 11 580 1920 4.5 4.5 .times. 10.sup.8 12 580 -- 0.21 0.21
.times. 10.sup.8 13 1185 1315 5.5 5.5 .times. 10.sup.8 14 1185 --
0.21 0.21 .times. 10.sup.8
[0391] This example demonstrates that it is possible to make very
large libraries of cells with integrated antibody cassettes. The
transfection efficiency ranged from 2.7 to 6.1%. In the case of the
.beta.-galactosidase selected population (sample 13) a library of
5.5.times.10.sup.8 clones can be created in a single flow
electroporation session. With more than one session in a day, a
library of 2-5.times.10.sup.9 clones can be generated.
[0392] After 13 days of blasticidin selection (10 .mu.g/ml), cells
were labelled with phycoerythrin labelled anti-Fc antibody
(Biolegend, Cat. No. 409304) as described earlier. Of the antibody
population selected on .beta.-galactosidase, 34-36% of cells were
positive for Fc expression and 11-13% were positive for binding of
Dyelight-633-labelled antigen at 10 nM concentration.
[0393] Where FGFR binding clones were used, 98-99% of cells were
positive for Fc expression. Use of a mixture of .alpha.-FGFR1_A and
.alpha.-FGFR2_A antibodies affords an opportunity to examine the
proportion of cells containing multiple integration events. For an
individual cell with a correctly-integrated cassette (e.g.,
.alpha.-FGFR1_A) there is approximately a 50:50 chance that a
second integration will be of the alternative specificity (i.e.,
.alpha.-FGFR2_A). If there are frequent multiple integrations, then
the proportion of double-positive clones will be high, however, the
proportion of double-positive clones was not found to be high,
illustrating the fidelity of the nuclease-directed library
integration system in generating one antibody gene/per cell. The
ability of the surface displayed anti-FGFR antibodies to
specifically bind their appropriate antigen was confirmed.
Expression of antigen was from a plasmid pTT3DestrCD4(d3+4)-His10
[134] encoding mouse Fgfrl ectodomain (ENSMUSP00000063808). This
was used to transfect HEK293 suspension cells and secreted
Fgfrl-rCd4-His10 purified by immobilised metal affinity
chromatography as described previously [134]. Mouse Fgfr2
ectodomain was PCR amplified from IMAGE clone 9088089 using
primers:
TABLE-US-00018 2423 (SEQ ID NO: 97)
(TTTTTTCCATGGGCCGGCCCTCCTTCAGTTTAGTTGAG) and 2437 (SEQ ID NO: 98)
(TTTTTTGCGGCCGCGGAAGCCGTGATCTCCTTCTCTCTC),
digested with Nco1iNotI and cloned into expression plasmid pBIOCAM5
[126]. Fgfr2-Fc was expressed by transient transfection of HEK293
cells as described previously [134] and purified by affinity
chromatography.
[0394] Transfected populations were probed for dual binding using
both of the labelled antigens and the proportion of double
positives was low. In this experiment the optimal balance of
library size (2.7.times.10.sup.8 clones/per Maxcyte session) with
low percentage of double positives (3.5%) was found using 197 ng
donor DNA per 10.sup.6 cells (FIG. 23).
[0395] This proportion of double positives could represent
mis-incorporation of a second antibody cassette but given the
efficiency of nuclease-directed integration of the library it is
also possible that both alleles (the AAVS locus in this example)
could be targeted with incoming binders in a proportion of cells.
The presence of two different antibody genes within a cell in
itself does not prevent the isolation of binders or their encoding
genes but this can be circumvented by first modifying the target
cell at a single locus to introduce a single nuclease targeting
site, e.g., a pre-integrated SceI meganuclease site as demonstrated
in example 9.
Example 16. Recovery of Genes Encoding Binders from Sorted Library
Populations
[0396] Phage display selections were carried on
.beta.-galactosidase (as described in example 6) and antibody
populations from 1-2 rounds of selection were cloned into vector
pD6 and introduced into the AAVS locus of HEK293 cells as described
in example 6. .beta.-galactosidase was labelled using Lightning
Link Dyelight-633 (Innova Bioscience Cat. No 325-0010) according to
manufacturer's instructions. Transfected cell populations were
selected for 25 days in blasticidin (10 .mu.g/ml) and were labelled
with 10 nM Dyelight-633 labelled .beta.-galactosidase and
phycoerythrin-labelled anti-Fc (Biolegend Cat. No. 409304). Cells
were incubated with antibodies for 30 minutes at 4.degree. C.,
washed twice in PBS/0.1% BSA, re-suspended in PBS/0.1% BSA and
double-positive cells sorted using a flow sorter.
[0397] Sorted cells were expanded and a second round of sorting was
carried out using 10 nM antigen. Cells were grown and either
genomic DNA or mRNA was isolated from the sorted, selected
populations.
[0398] Where binders encompassing different chains (e.g., IgG
formatted antibodies) are present on the same genomic sequence
(e.g., by introduction on the same plasmid) but are transcribed
into different mRNAs it may be optimal to recover the separate
genes encoding the multimeric binder by amplification from genomic
DNA. As an alternative, binders encompassing multiple protein
chains can be encoded on the same mRNA through the use of "internal
ribosome entry sequence" (IRES) elements or sequences such as viral
P2A or T2A sequences that promote translational stalling/protein
cleavage [133, 136]. In this case and in the case of binders
encoded on a single protein chain it will also be possible to
isolate the encoded genes from mRNA.
[0399] Genomic DNA was prepared using the "DNeasy blood and tissue
kit" (QIAGEN Cat. No. 69504). mRNA was prepared using an "Isolate
II RNA mini kit" (Bioline Bio-52072). For amplification from
genomic DNA a PCR reaction was set up using Phusion polymerase with
the "2.times.Phusion GC" mix according to manufacturer's
instructions. Primers which flank the Nco1 and Not1 cloning sites,
e.g., primers 2622 (GAACAGGAACACGGAAGGTC) (SEQ ID NO: 99) and 2623
(TAAAGTAGGCGGTCTTGAGACG) (SEQ ID NO: 82) were used to amplify the
antibody cassette (98.degree. C. 10 secs, 58.degree. C. 20 secs,
72.degree. C. 90 secs for 35 cycles.
[0400] Genes encoding selected scFv genes were amplified from mRNA.
Total RNA was isolated from the sorted cells using the "Isolate II
RNA mini kit" (Bioline Cat No Bio-52072). cDNA was synthesized from
2 g RNA using Superscript II reverse transcriptase (Life
Technologies, Cat no 180064-022). The selected scFv genes were then
amplified from the cDNA by PCR using KOD Hot Start DNA polymerase
(Merck Millipore Cat. No. 71086-3) using primers which flank the
Nco1 and Not1 cloning sites. In this case, primers:
TABLE-US-00019 41679 (SEQ ID NO: 100) ATGAGTTGGAGCTGTATCATCC and
2621 (SEQ ID NO: 101) GCATTCCACGGCGGCCGC
were used to amplify the antibody cassette (95.degree. C. for 20
seconds, 60.degree. C. for 10 seconds, 70.degree. C. for 15
seconds, for 25 cycles). PCR products were digested with Nco1 and
Not 1 before cloning into the Nco/Not1 site of bacterial antibody
expression vector pSANG10. Construction of pSANG10, methods for
bacterial expression and screening by ELISA are described in Martin
et al 2006 [127].
[0401] ELISA screening of the population from 1 round of selection
by phage display revealed that 0/90 of the clones were positive. In
contrast, when this same population was further subjected to
mammalian display and the scFv gene population was recovered and
screened, 27/90 clones (30%) were positive in ELISA. This
illustrates that it is possible to carry out mammalian display on a
library and recover an enriched population of binders.
[0402] Example 15 describes the introduction of the population
selected by 1 round of phage display on .beta.-galactosidase into
HEK cells using flow electroporation. The mammalian display cell
population was selected in blasticidin as before and was subjected
to flow sorting using 10 nM of labelled .beta.-galactosidase. After
9 days growth 75% of cells were found by flow cytometry to be
positive for .beta.-galactosidase binding using 10 nM
.beta.-galactosidase). These were sorted and expanded further. To
illustrate the potential to drive stringency, labelling was carried
out using either 1 nM or 10 nM antigen concentrations. 20.3% and
55.9% of cells respectively were sorted from each population. After
sorting, mRNA was prepared immediately from the sorted population
without additional cell culture. Following cloning, expression in
bacteria and ELISA screening (as before) it was found that the
success rate in ELISA increased with increasing stringency during
flow sorting. The clones displaying highest signal level came from
this group and the number of positives clones was also improved
(FIG. 24). This illustrates the ability to drive stringency of
selection within display populations, reflected in the better
performance of the resulting antibodies.
Example 17. Incorporation of T Cell Receptors (TCRs) Genes for
Mammalian Library Production by Nuclease-Directed Integration
[0403] The methods described herein have application beyond display
of antibodies. To demonstrate the potential for screening libraries
of T-cell receptors using nuclease-directed integration, a vector
construct (pINT20) allowing expression of T-cell receptors was
constructed.
[0404] pINT20 (FIG. 25a) is a dual promoter vector for targeting
the human AAVS locus. It has left and right homology arms as
presented in FIG. 3. The left AAVS homology arm is flanked by
unique AsiSI and Nsi1 sites and is followed by a splice acceptor
and a puromycin gene. The sequence between the end of the left
homology arm and the splice acceptor is the same as previously
described and the puromycin gene commences with an ATG in frame
with the upstream exon ((FIG. 25B and as also shown for the
blasticidin gene in FIG. 3). Correct nuclease-directed integration
will lead to in-frame splicing of a puromycin gene which is spliced
to an endogenous upstream exon which itself driven by the
endogenous AAVS promoter allowing selection of clones with correct
integration. The puromycin resistance gene is followed by an SV40
polyadenylation site. The right AAVS homology arm is flanked by
unique BstZ171 and Sbf1 sites.
[0405] pINT20 is configured with a pEF promoter (from pSF-pEF,
Oxford Genetics Cat. No. OG43), which allows genes of interest to
be cloned into Nhe1/Kpn1 sites. The Nhe1 site is preceded by a
secretion leader sequence and the Kpn1 site is followed by the
polyadenylation signal of bovine growth hormone (bGH poly A) as
shown previously in FIG. 2. Downstream is a CMV promoter (from
pSF-CMV-f1-Pac1, Oxford Genetics Cat No OG111) allowing cloning via
Nco and Not (as shown in FIG. 2) or Hind3. The Nco1 site is
preceded by a secretion leader sequence and the cassette is
followed by a bGH polyA site.
[0406] To exemplify display and enrichment of T cell receptors
(TCRs) a TCR recognising a cancer marker described by Li et al.
(2005) and later by Zhao et al. (2007)[137, 138] was used. This TCR
called c12c2 recognises the peptide SLLMWITQV (SE ID NO: 102)
presented on HLA-A2 with an affinity of 450 nM. This peptide
represents residues 157-165 from NY-ESO-1 (NY-ESO-1 157-165). This
is an affinity-matured variant of a parental antibody called 1G4
with affinity of 32 .mu.M.
[0407] A second TCR was used which recognises another cancer
marker. The parental MEL5 TCR recognises the peptide MART-1 26-35
("Melanoma antigen recognised by T cells-1") presented on HLA-A2
with peptide sequence ELAGIGILTV (SEQ ID NO: 103). This TCR was
affinity matured by phage display to give clone a24/017 with 0.5 nM
affinity described in Madura et al. (2013) [139]. The structure of
the complex between TCR and MHC:peptide complex has been solved
(pdb code 4JFH) and the clone is hereafter named as "4JFH". The
same parental TCR has also been engineered based on design by
Pierce et al. (2014) [140] and the structure of the complex
solved.
[0408] According to Debets and colleagues the attachment of the CD3
domain as shown tends to cause association of the heterologous gene
even in the presence of native human TCRs [141, 142]. The CD3 .xi.
element used is composed of an extracellular domain, a
transmembrane domain and a complete cytoplasmic domain. In addition
substitution of human constant domains by mouse constant domains in
the heterologous genes also tends to drive their association over
association with endogenous human constant domains [143]. Finally
the use of mouse constant domains offers the option of detecting
the heterologous TCR chains against a background of human TCRs.
These elements were incorporated into the design of the TCR
expression cassette.
[0409] Two synthetic genes were designed and synthesised giving
rise to gene constructs with the following structure:
Human TCR V.alpha.-mouse .alpha. constant-human CD3 .xi. Human TCR
V.beta.-mouse .beta. constant-human CD3 .xi.
[0410] The sequence of the synthetic gene incorporating the .alpha.
chain and the .beta. chain constructs incorporating the variable
domains of c12/c2 is shown in FIGS. 25c and d. These are cloned
into the Nhe1/Kpn1 sites and the Nco1/Hind3 sites of pINT20
respectively. The construct encoding this TCR is called
pINT20-c12/c2. In the first instance the synthetic gene was
designed to incorporate a V.alpha. C.alpha. domain (flanked by Nhe1
and Not 1 sites) and V.beta. domain (flanked by Nco1/Xho1 sites
encoding the TCR c12/c2). These elements can then be replaced by
alternative TCRs using these restriction sites.
[0411] Two additional synthetic genes were made encoding the
V.alpha. and V .beta. domains of 4JFH [139] (FIGS. 25e and f). The
construct encoding this TCR is called pINT20-4JFH.
[0412] 10.sup.7 HEK293 cells were transfected using 3 .mu.g of
pINT20-c12/c2 and pINT20-4JHF as donor DNA (300 ng donor DNA per
10.sup.6 cells) in the ratios shown in Table 7. pINT20-c12/c2 is
referred to as TCR1 and pINT20-4JHF is referred to as TCR2. 5 g
each of pZT-AAVS1 L1 and pZT-AAVS R1 TALENs were added to 10.sup.7
cells (500 ng each per 10.sup.6 cells) with the exception of sample
6 where this was replaced by 10 .mu.g of control DNA (pcDNA3.0).
DNA was introduced by polyethyleneimine transfection, as described
above.
TABLE-US-00020 TABLE 7 Sample TCR1/TCR2 ratio 1 1:100 2 100:1 3
50:50 4 100% TCR1 5 100% TCR2 6 50:50 (no TALE nuclease)
[0413] Following 12 days in selection (0.25 .mu.g/ml puromycin)
cells were labelled with target antigen. The peptide:MHC complexes
recognised by the TCRs described above are presented as a
phycoerythrin labelled pentamer (ProImmune). c12/c2 recognises
peptide SLLMWITQV presented on HLA-A2 representing NY-ESO-1 157-165
(Proimmune product code 390). 4JHF (also known as
.alpha.24/.beta.17) recognises peptide ELAGIGILTV presented on
HLA-A2 representing MelanA/MART 26-35 (Proimmune product code 082).
In each case the MHC:peptide complex was labelled with
phycoerythrin and used according to manufacturer's instructions.
FIG. 26 (a-d) shows that each TCR is specific for the expected MHC:
peptide complex (a, d) and fails to bind to the non-cognate peptide
in complex (FIG. 26b, c). DNA encoding each TCR was mixed with a
100-fold excess of DNA encoding the other (samples 1-2, Table 7).
HEK293 cells were transfected and selected in puromycin. Sorting of
antigen positive cells was performed after 14 days of puromycin
selection (FIG. 26g, h).
[0414] In order to quantitate the level of enrichment within the
flow-sorted output populations, the TCR genes were recovered by PCR
amplification and the relative amounts of each TCR species
determined following cloning. Total RNA was isolated from the
sorted population. cDNA synthesis was performed as described in
example 16. Primers to amplify the TCR alpha and beta chain were
1999/2782 and 41679/2789 respectively (Table 8). PCR amplification
employed KOD hot start polymerase using the manufacturer's
recommended protocol (EMD Millipore, 71086, EMD Millipore). PCR
reaction conditions were 95.degree. C. (2 mins) and 25 cycles of
95.degree. C. (20 s), 60.degree. C. (10 s), 70.degree. C. (15 s)
followed by 70.degree. C. (5 mins). The amplified TCR alpha and
beta chains were digested with NheI/NotI or NcoI/XhoI and
sub-cloned into vectors with compatible sites (in this case
pBIOCAM1-Tr-N NheI/NotI or pBIOCAM2-Tr-N(NcoI/XhoI) cut vectors
respectively). PCR of individual clones were amplified with a c2c12
(TCR1) specific TCR alpha primer (2781) or a 4JFH (TCR2) specific
TCR alpha primers (4JFH-V.alpha.-F) and vector specific primers to
assay for TCR clone identity. After sorting of the sample 1 (Table
7) where a ratio of 1:100 TCR1/TCR2 donor plasmid was employed
(Table 7) with enrichment for TCR1 specific clones using peptide
SLLMWITQV presented on HLA-A2 (Proimmune, product code 390), the
proportion of TCR1 clones, as determined by colony PCR, increased
to 11/15 (73%). Enrichment by sorting the sample 2 where a ratio of
100:1 TCR1/TCR2 donor plasmid was employed (Table 7), with peptide
ELAGIGILTV presented on HLA-A2 (Proimmune product code 082)
resulted in an increase of the proportion of TCR2 clones to 4/15
(27%), determined by colony PCR.
[0415] To demonstrate library selection using nuclease-directed
integration, a mutant library based on c12/c2 was created by
cloning a repertoire of genes encoding mutant TCR alpha chains
along with a repertoire of genes encoding mutant TCR beta chains.
Such a library could be created using oligonucleotide-directed
mutagenesis approaches. e.g., methods based on Kunkel mutagenesis
[144]. As an alternative and by way of example, a PCR assembly
approach was used to create a mutant TCR alpha chain (as a
Nhe1/Kpn1 fragment) and a mutant TCR beta chain (as a Kpn/Hind 3
fragment, including the CMV promoter) which is cloned into the
Nhe1/Hind 3 site of pINT20. This was done using primers shown in
Table 8.
TABLE-US-00021 TABLE 8 Primers used in library construction and
clone recovery 4JFH-V.alpha.-F ACACACGCTAGCCAGAAAGAGGTGGAACAG (SEQ
ID NO: 104) 1999 AAAAAGCAGGCTACCATGAGGGCCTGGATC (SEQ ID NO: 87)
TTCTTTCTCC 2770 CAAAGAACAGCTCGCCGGTSNNCCCGASSN (SEQ ID NO: 105)
NGGAGCTGGCGCAAAAGTAC 2771 CTCGCCCGAAGGTGGGAATGTANGWTCCSN (SEQ ID
NO: 106) NSNNAAGTGGGCGCACGGCGCAC 2780 CTGGCAGCTAGCAAGCAGGAAG (SEQ
ID NO: 107) 2781 TACATTCCCACCTTCGGGCGAG (SEQ ID NO: 108) 2782
TTTTTTGCGGCCGCGGACAGGTTCTG (SEQ ID NO: 109) 2783
CGTAAGCTGGTACCTTATTATCTAGGG (SEQ ID NO: 110) 2785
CCCTAGATAATAAGGTACCAGCTTACG (SEQ ID NO: 111) 2787
ACCGGCGAGCTGTTCTTTG (SEQ ID NO: 112) 2788
AGTGACAAGCTTTTATTATCTGGGTG (SEQ ID NO: 113) 2789
CAGGTCCTCGAGCACTGTC (SEQ ID NO: 114) 41679 ATGAGTTGGAGCTGTATCATCC
(SEQ ID NO: 100) (N = A, C, G, T. S = C OR G, W = A OR T)
[0416] A mutant oligonucleotide was designed (primer 2771) which
randomised 2 amino acid positions within CDR3 of the c12/c2 alpha
chain and provide the option of either serine or threonine at
another position (primer 2771 is also represented by the lower
strand of FIG. 25g). Primer 2771 was used in conjunction with
primer 2780 to create mutant TCR alpha repertoire going from the
Nhe1 cloning site incorporating the region of CDR3 mutagenesis with
an invariant sequence at the end. Primer 2781 is complementary to
the invariant 5' end of primer 2771. PCR with primers 2781 and 2783
provided the remainder of the TCR alpha-CD3 zeta cassette up to the
Kpn1 site. PCR assembly of the 2 PCR fragments is used to create
the TCR alpha-CD3 zeta fragment which can be cloned into pINT20
following digestion with Nhe1 and Kpn1.
[0417] A second mutant oligonucleotide (primer 2770) was designed
which randomised 2 amino acid positions within CDR3 of the c12/c2
beta chain and provide the option of either valine or leucine at
another position (primer 2770 represented by the lower strand of
FIG. 25h). Primer 2770 was used in conjunction with primer 2785 to
create a mutant TCR beta repertoire from the Kpnl cloning site
incorporating the region of CDR3 mutagenesis with an invariant
sequence at the end. Primer 2787 is complementary to the invariant
5' end of primer 2700. PCR with primers 2787 and 2788 provided the
remainder of the TCR beta-CD3 zeta cassette up to the Hind 3 site.
PCR assembly of these 2 PCR fragments is used to create a TCR
beta-CD3 zeta fragment which can be cloned into pINT20. A complete
repertoire incorporating mutations at both CDR 3 of alpha and beta
chains was created by cloning of the Nhe1/Kpn fragment, the
Kpn1/Hind3 fragment into Nhe1/Hind3 digested pINT20. Following
ligation the library was cloned into electrocompetent DH10B cells.
Plasmid DNA was prepared and the DNA transfected into HEK293 cells
along with vectors encoding TALE nuclease as described earlier (an
equimolar mix of pZT-AAVS1 L1 and pZT-AAVS R1 Systems Bioscience
Cat. No. GE601A-1).
[0418] Following ligation of the mutant alpha and beta chains of
the c12/c2 mutant library into pINT20, DNA was electroporated into
DH10B cell, plasmid DNA was prepared and the library was
co-transfected with TALE nuclease targeting the AAVS locus.
Transfection was performed using Maxcyte electroporation. Growth
and selection were as described above. Quantitation of the library
size, by titration of transfected cells and plate-based selection
in puromycin, indicated that a library size 5.times.10.sup.5 was
created. After puromycin selection for 11 days cells were labelled
with an APC-labelled antibody specific to the .beta. chain of the
mouse TCR (Life Technologies Cat H57-957). FIG. 26i-j shows 38% of
clones in the population express a T cell receptor. Of this TCR
positive population, 13% also bound peptidel (5% of the total
population). By this approach clones with improved expression or
peptide:MHC binding activity can be isolated.
[0419] From FIG. 26 it can be seen that each T cell receptor
recognised only its cognate antigen. Furthermore when a mixture of
the two different specificities are used it is possible to
distinguish each of them through the labelled antigen. This
approach also allows TCR clones with improved affinity (or
expression) to be distinguished within the mutant TCR library by
identifying a subset of the library that was labelled to a greater
extent than the parental clone.
[0420] T cell receptor genes were also introduced into Jurkat cells
by electroporation. Jurkat cells were centrifuged and re-suspended
in a final volume of 10.sup.8 cells/ml in the manufacturer's
electroporation buffer (Maxcyte Electroporation buffer, Thermo
Fisher Scientific Cat. no NC0856428)). An aliquot of
4.times.10.sup.7 cells (0.4 ml) was added to the OC400
electroporation cuvette with 40 .mu.g DNA (i.e., 1 .mu.g/10.sup.6
cells). DNA consisted of a mixture of donor plasmid DNA
(pINT20-c12/c2 or pINT20-4JHF or pINT20-c12/c2 TCR library, 9.2
.mu.g) and an equimolar mix of DNA (30.8 .mu.g total) of DNA
encoding the AAVS-SBI TALENs (pZT-AAVS1 L1 and pZT-AAVS R1 Systems
Bioscience Cat No GE601A-1). In samples without added TALENs the
input DNA was brought to 1 jg/10.sup.6 cells using control plasmid
pcDNA3.0. An alternative method of introducing T cell receptor
genes into Jurkat cells used the 4D-Nucleofector (Lonza). Here, the
transfection protocol followed the manufacturer's instructions
according to the SE cell-line kit (Lonza, Cat. PBC1-02250).
Briefly, 2 .mu.g of DNA, consisting of a mixture of donor plasmid
DNA (pINT20-c12/c2 or pINT20-4JHF or pINT20-c12/c2 TCR library,
0.46 .mu.g) and an equimolar mix of DNA (1.54 .mu.g total) of DNA
encoding the AAVS-SBI TALENs (pZT-AAVS1 L1 and pZT-AAVS R1 Systems
Bioscience Cat No GE601A-1) was transfected per 10.sup.6 Jurkat
cells. The pulse code setting was C.sub.L120 and cell type
programme was specific for Jurkat E6.1 (ATCC) cells.
[0421] FIG. 26 demonstrates that TCR expression was achieved and
recognition of the appropriate peptide:MHC molecule was achieved.
This was dependent on the use of TALE nuclease (compare FIGS. 26m
and n). Signalling through the introduced TCR was also achieved
using the relevant peptide:MHC molecule.
[0422] pINT20-c12/c2 transfected Jurkat cells or untransfected
Jurkat cells were plated in a 96-well plate at a density of
1.times.10.sup.6/ml, 200 .mu.l per well. Cells were stimulated with
either 2 .mu.l or 6 .mu.l per well of PE labelled peptide 1-MHC
pentamer (ProImmune) or 2 .mu.g/ml anti-human CD3 (BD Pharmingen,
Cat 555329) in the presence and absence of anti-human CD28 (BD
Pharmingen Cat 555725) at 2 .mu.g/ml. After a 24 hour incubation at
37.degree. C. and 5% CO.sub.2, the activation of Jurkat cells was
detected by investigating CD69 expression. Cells were stained with
50l PBS+1% BSA+0.5 .mu.l of anti-human CD69-APC (Invitrogen, Cat.
MHCD6905) per well for 45 minutes at 4.degree. C.
[0423] FIG. 26 (sample o and p) demonstrates up-regulation of CD69
upon stimulation with CD3. The figure also shows the effect of
adding 2 .mu.l (q) or 6 .mu.l (r) of peptidel:MHC. A population of
double-positive cells which bind peptide:MHC and express CD69 is
obvious. This example shows cells incubated in the presence of CD28
but the same effect was observed in the absence of CD28 (not
shown).
[0424] This example demonstrates the potential of nuclease-directed
integration of libraries of an alternative type of binder, i.e., T
cell receptors. We demonstrate that it is possible to express and
detect TCR expression on the cell surface using specific
antibodies. We also demonstrate that these T cell receptors
specifically recognise their respective targets. We have also
constructed a mutant library allowing selection of improved
binders. Finally we have demonstrated that library screening based
on activation of TCR signalling in T cells is possible. Here we
have used a cultured human T cell line. It is also possible to
introduce DNA into primary T cells by Maxcyte electroporation.
Methods for the isolation and preparation of primary T lymphocytes
are known to those skilled in the art (e.g., Cribbs et al., 2013,
Oelke et al. 2003 [145, 146]. Exposure of TCR transfected
lymphocytes to multimeric peptide:MHC can then be used to achieve
activation either through exposure to peptide:MHC multimers [146]
or to antigen presenting cells loaded with the appropriate peptide
[146, 147]. Activation can be detected either through expression of
reporter genes or through up-regulation of endogenous genes such as
CD69 [104, 148].
Example 18. Display of Libraries of Chimeric Antigen Receptors on
Mammalian Cells
[0425] Activation of T cells normally occurs through interaction of
the T Cell Receptor (TCR) with specific peptide:MHC complexes. This
in turn leads to signalling directed via CD3 and other T cell
signalling molecules. As an alternative to target recognition
directed by the TCR it has been shown that alternative binding
molecules such as single chain Fvs can be presented on T cells as
fusions to downstream signalling molecules in a way that re-directs
T cell activation to the molecule recognised by the scFv (or
alternative binder). In this way T cell activation is no longer
limited to the molecular recognition directed towards peptide:MHC
complexes by TCRs but can be directed to other cell surface
molecules. This alternative format wherein a non-TCR binding entity
is fused to a signalling component is referred to as a "chimeric
antigen receptor" (CAR). In the case of T cells this has been shown
to be an important and valuable means of re-directing T cell
activation.
[0426] For any given target it is still not clear what the optimal
epitope or affinity features should be for incorporation into a CAR
[103]. Features of the CAR design such as linker length, or choice
of transmembrane domain may in turn affect what constitutes an
optimal epitope. The combination of antigen density on target and
non-target cells together with the choice of signalling domain
could affect the optimal affinity requirements. The ability to
present libraries of chimeric antigen receptors on T cells affords
an opportunity to identify optimal binding specificity, binder
format, linker length/sequence, variants of fused signalling
module, etc., either alone or in combination. Here we demonstrate
the utility of nuclease-directed integration for construction of
libraries of chimeric antigen receptors in mammalian cells. The
vector pINT21 (FIG. 27a) is a single CMV promoter vector for
convenient expression and secretion of binders such as scFvs
flanked by Nco1/Not1 restriction sites to allow in frame expression
with an upstream leader sequence and a downstream fusion partner
(as shown earlier in FIG. 8). The CAR expression cassette in pINT21
is flanked by AAVS homology arms as described earlier in FIG.
3.
[0427] The vector pINT21-CAR1 (FIG. 27a, c) fuses binders such as
single chain Fvs to the transmembrane domain and intracellular
domain of CD3.xi. (FIG. 27c and as described for TCR expression in
FIG. 25). This format is often referred to as a "first generation"
chimeric antigen receptor. Signalling domains from other
co-stimulatory molecules have also been used to provide additional
signals and these have been shown to give improved signalling.
These have been referred to as second and third generation chimeric
antigen receptors. For example pINT21-CAR2 (FIG. 27b, d)fuses the
binder (conveniently cloned in this case as an Nco1/Not 1 fragment
to a previously described second generation domain (WO 2012/079000
A1) consisting of:
The hinge and transmembrane domain from CD8 4-1BB signalling domain
CD3.xi. signalling domain
[0428] By way of example a number of different binder groups were
cloned into the Nco/Not1 sites of pINT20-CAR1 and pINT20-CAR2. CD19
has previously been used in a number of different studies to target
B-cell malignancies references in Sadelain et al. (2013) [103]. A
previously described anti-CD19 antibody (WO 2012/079000 A1) (called
FMC63) was prepared as a synthetic scFv gene in either a
VH-linker-VL configuration or a VL-linker-VH configuration (FMC63
H-L or FMC L-H respectively, FIG. 27E shows the sequence of FMC63
H-L. FMC63 L-H was configured with the variable domains in
VL-linker-VH configuration flanked by Nco1 and Not1 at 5' and 3'
ends respectively.
[0429] As controls, scFvs with alternative binding specificities
were also cloned into pINT20. These include anti-FGFR1_A [105] and
an anti-desmin control antibody [7]. In addition, Adhirons [152]
recognising lox1 (FIG. 29a, b) were introduced as an example of an
alternative format of binder configured as a CAR fusion (see
example 19 for description).
[0430] To demonstrate the creation of libraries of binders
presented in a CAR format, populations of scFv-formatted antibodies
selected on mesothelin and CD229 were cloned. Mesothelin is a cell
surface glycoprotein which is highly expressed in a number of
cancers including mesothelioma. A number of antibody-based formats
are under development and in clinical trial including CARs directed
to mesothelin [149]. CD229 represents another potential
tumour-associated antigen which could be targeted by immune therapy
in cancers such as chronic lymphocytic leukaemia and multiple
myeloma [150, 151].
[0431] A population of antibodies recognising either mesothelin or
CD229 was created by selection using the "McCafferty" phage display
library as described in example 6 and ref 7). Two rounds of
selection were carried out and the scFv-encoding genes recovered
using primers M13leadseq and Notmycseq (example 6). Products were
cut with Nco1/Not 1, gel purified and cloned into pINT21-CAR2.
These were directed into the AAVS locus of HEK293 cells by TALE
nuclease cleavage to generate a library of 4.8.times.10.sup.5 for
CD229 and 6.4.times.10.sup.5 for mesothelin (representing a
30.times. and 53.times. increase in library compared to samples
transfected in the absence of TALE nuclease).
[0432] pINT20-CAR1 and pINT20-CAR2 were introduced into HEK293
cells by PEI transfection. Here donor plasmid DNA (pINT20-CAR1 or
pINT20-CAR2, 6 .mu.g was mixed with an equimolar mix of DNA (20
.mu.g total) of DNA encoding the AAVS-SBI TALENs (pZT-AAVS1 L1 and
pZT-AAVS R1 Systems Bioscience Cat No GE601A-1) in Freestyle 293
media (Lifetech, Cat. 12338-026), linear PEI (52 al, 1 mg/ml,
Polysciences Inc.) added and incubated at room temperature for 10
mins. The mixture is then added to 20 ml HEK293 suspension cells
(1.times.10.sup.6 cell/ml) in a 125 ml vented Erlenmeyer flask.
pINT20-CAR1 and pINT20-CAR2 were also introduced into Jurkat cells
by electroporation. Jurkat cells were centrifuged and re-suspended
in a final volume of 10.sup.8 cells/ml in the manufacturer's
electroporation buffer (Maxcyte Electroporation buffer, Thermo
Fisher Scientific Cat. no NC0856428)). An aliquot of
4.times.10.sup.7 cells (0.4 ml) was added to the OC400
electroporation cuvette with 40 .mu.g DNA (i.e., 1 .mu.g/10.sup.6
cells). DNA consisted of a mixture of donor plasmid DNA
(pINT20-CAR1 and pINT20-CAR2, 9.2 .mu.g) and an equimolar mix of
DNA (30.8 jg total) of DNA encoding the AAVS-SBI TALENs (pZT-AAVS1
L1 and pZT-AAVS R1 Systems Bioscience Cat No GE601A-1). In samples
without added TALENs, the input DNA was brought to 1 .mu.g/10.sup.6
cells using control plasmid pcDNA3.0.
[0433] Fluorescent labelling of the various antigens was performed
using Lightning-Link Rapid Dye-Light 633 conjugation kit (Innova
Biosciences, cat. 325-0010). Preparation of FGFR1 and FGFR2 is
described in example 15. Lox1 and CD229 were from R and D Systems
(Cat. Nos. 1798-LX-050, and 898-CD050 respectively), CD19-Fc and
mesothelin were from (AcroBiosystems Cat. No. CD9-H5259 and.
MSN-H526.times. respectively).
[0434] FIG. 28b illustrates display of nuclease-directed anti-FGFR1
antibody [105] within a second generation CAR construct
(pINT21-CAR2-FGFR1_A). FIG. 28d also illustrates display of an
alternative scaffold molecule (an Adhiron ref [152]) as a fusion
with a second generation chimeric antigen receptor
(pINT21-CAR2-lox1). FIGS. 28f and g also illustrate positive clones
within a library of scFvs selected on mesothelin or CD229.
[0435] In this example CARs were introduced into HEK cells and
Jurkat cells but this could equally be done by introducing the
constructs into primary cells such as human T lymphocytes (e.g., as
described by Sadelain et al. (2013) [103], for example using
electroporation [135]. Expression constructs for CAR expression in
lymphocytes may be further optimised, e.g. by optimising mRNA
stability and translation through variation in 5' and
3'untranslated regions, poly A length etc., as has previously been
described [135]. Signalling of CAR constructs introduced into
primary T lymphocytes or T lymphocyte cell lines can be induced by
exposure to cells expressing target antigen or using multimeric
antigen, e.g. antigen immobilised on a surface or presented on
beads [104, 148].
Example 19. Display of Libraries of Alternative Scaffolds
Constructed in Mammalian Cells Via Nuclease-Directed
Integration
[0436] The method described for constructing libraries of binders
can be employed beyond display of antibodies and T cell receptors.
A number of alternative scaffolds have been described allowing
construction of libraries of variants from which novel binding
specificities have been isolated, e.g., Tiede et al. (2014) [152]
and references therein. In the example described by Tiede et al.
(2014) a stable, versatile scaffold based on a consensus sequence
from plant-derived phytocystatins was used. This scaffold is
referred to as an Adhiron and FIG. 29a shows a synthetic gene
encoding an Adhiron which was selected to bind to lox1 (WO
2014125290 A1). FIG. 29B shows an alternative lox1 binder (lox1B).
Both were synthesised and cloned into the Nco1/Not1 site of
pINT20_CAR2 to create a fusion with the downstream partner.
[0437] A library can be constructed by randomising loop residues
(e.g., by Kunkel mutagenesis or PCR assembly as described above in
example 17). By way of example, FIG. 29c shows the design of a
mutant oligonucleotides useful to create a library following the
same approach as described in example 17. In this case,
randomisation is achieved by introducing a variable number of NNS
residues, although alternative strategies known to those skilled in
the art could be used.
[0438] As another example FIGS. 29d and e demonstrates the means to
create a library of binders by nuclease-directed integration based
on a knottin scaffold [156]. Knottins are peptides of approximately
30 amino acids which are stabilised by three disulphide bonds, with
one threaded through the other two to create a "knotted" structure.
FIG. 29d shows the trypsin binding knottin MCoTI-II with an Nco1
site at the 5' end and a Not site at the 3' end allowing in-frame
expression with the vectors described herein. As an example for
library construction the 6 amino acids of the first loop
(underlined in FIG. 29d) can be mutated with variable number of
amino acids. FIG. 29e illustrates a mutagenic strategy replacing
the 6 amino acids of loop 1 with 10 randomised amino acids using
the codons VNS (where V=A, C or G and S.dbd.C or G). The VNS codon
encompasses 24 codons encoding 17 amino acids which exclude
cysteines. This strategy is for illustrative purposes and
alternative mutagenic strategies will be known to those skilled in
the art.
Example 20. Nuclease-Directed Introduction of Antibody Libraries
Using CRISPR/Cas9
[0439] Nuclease-directed integration via CRISPR/Cas9 was
demonstrated using the "Geneart CRISPR nuclease vector kit"
(Lifetech A21175). In this system, a U6 RNA polymerase III promoter
drives expression of a target complementary CRIPSR RNA (crRNA)
which is linked to a trans-activating crRNA (tracrRNA). The crRNA
and tracrRNA together make up a guide RNA which directs the
cleavage specificity of a Cas9 protein encoded on the same "GeneArt
CRISPR nuclease vector" (see manufacturer's instructions). The
vector is provided as a linearised plasmid into which a short
double-stranded oligonucleotide with appropriate 3' overhangs is
cloned. Cleavage specificity is then determined by the sequence of
the cloned segment. Two different targeting sequences were designed
to direct cleavage to the human AAVS locus describe above.
[0440] The sequences were:
TABLE-US-00022 CRISPR1 double-stranded DNA insert: (SEQ ID NO: 115)
5' GGGGCCACTAGGGACAGGATGTTTT (SEQ ID NO: 116) 3'
GTGGCCCCCGGTGATCCCTGTCCTAC CRISPR2 double-stranded DNA insert: (SEQ
ID NO: 117) 5' GTCACCAATCCTGTCCCTAGGTTTT (SEQ ID NO: 118) 3'
GTGGCCAGTGGTTAGGACAGGGATC
[0441] The resulting guide RNAs target cleavage within the same
region of the AAVS locus as the TALE nucleases described above
(with CRISPR2 being in the reverse orientation from CRISPR1). Thus
the AAVS homology arms used previously to direct integration of the
expression cassette can be used for integration directed by these
CRISPR guide RNAs. Linearised vector and double stranded
oligonucleotides were ligated and transformed into electrocompetent
DH10B cells. Cloning of the correct insert was confirmed by
sequencing and plasmid DNA was prepared. The Cas9/CRISPR2 construct
(encompassing the CRISPR2 oligonucleotide) was transfected together
with donor DNA encoding a .beta.-galactosidase library selected by
1 round of phage display selection (example 15). These were
transfected into HEK293 cells using Maxcyte electroporation system
with the OC-400 assembly. 4.times.10.sup.7 cells were transfected
with 23.2 .mu.g of donor DNA representing a population of scFv
genes selected by one round of phage display on
.beta.-galactosidase cloned into pD6. Cells were co-transfected
with either 77 .mu.g of Cas9-CRISPR2 plasmid, or 77 .mu.g TALEN
plasmid (38.5 .mu.g each of pZT-AAVS1 L1 and pZT-AAVS) or 77 .mu.g
control plasmid
TABLE-US-00023 TABLE 9 Transfection Number of clones Sample no
Nuclease used efficiency % per 10.sup.10 cells 1 Cas9/CRISPR 2
10.53 (29x) 10 .times. 10.sup.8 2 AAVS TALEN 5.1 (14x) 5.1 .times.
10.sup.8 3 None (pCDNA3.0) 0.36 0.36 .times. 10.sup.8
[0442] Titration of the number of transformants formed by
Cas9/CRISPR2 transfection (by measuring blasticidin resistance
colonies) revealed that 1053 blasticidin resistant colonies were
generated from plating 10,000 cells, equating to a transfection
efficiency of 10.5% (Table 9). In the case of TALE
nuclease-directed a transfection efficiency of 5.1% was achieved.
In contrast, in the absence of the Cas9/CRISPR2 construct only
0.36% transfection efficiency was achieved.
[0443] As an alternative to transfection using plasmid DNA to
introduce the Cas9 protein and guide RNA into cells it is also
possible to directly introduce a nucleoprotein complex consisting
of Cas9 protein (Toolgen, Inc.) and a guide RNA. Guide RNA was
prepared by Toolgen, Inc. using in vitro transcription from a T7
promoter as a single transcript which included the TRACR sequence
(italicized) preceded by sequence complimentary to the target DNA
(in bold) as shown below.
TABLE-US-00024 CRISPR 1 RNA (SEQ ID NO: 119): 5'
GGGGGGCCACUAGGGACAGGAUGUUUUAGAGCUAGAAAUAGCAAGUU
AAAAUAAGGCUAGUCCGUUAUCAACUUGAAAAAGUGGCACCGAGUCGGUG CUUUU CRISPR 2
RNA (SEQ ID NO: 120): 5'
GGGUCACCAAUCCUGUCCCUAGGUUUUAGAGCUAGAAAUAGCAAGUU
AAAAUAAGGCUAGUCCGUUAUCAACUUGAAAAAGUGGCACCGAGUCGGUG CUUUU
[0444] 6.6 .mu.g of Cs9 protein, 4.6 g of RNA and 10 g of donor DNA
(encoding the anti-FGFR1_A antibody in pD6) were introduced into
10.sup.7 HEK293 cells by Maxcyte electroporation as described
above. Transfection efficiencies of 2.2% and 2.9% were achieved for
CRISPR 1 and CRISPR2 RNA respectively with 0.7% and 0.8% in the
absence of added Cas9:RNA protein complex.
[0445] These guide RNAs target the same sequences encoded by
CRISPR1 and CRISPR2. As an alternative, crRNA and tracrRNA can be
made by chemical synthesis (e.g., GE Dharmacon).
Example 21: Nuclease Mediated Antibody Gene Insertion by Ligation
or Microhomology-Mediated End-Joining (MMEJ)
[0446] Although homologous recombination (HR) is useful for the
precise insertion of large DNA fragments, this requires the
construction of large targeting vectors incorporating long homology
arms. This can make the construction of large libraries more
difficult due to the reduced transformation efficiency of larger
DNA constructs. Alternatively, simple ligation reactions can occur
between the chromosomal DNA and targeting vector, if a nuclease
recognition sequence is incorporated into the targeting vector. The
ligation reactions can either be "sticky-end" employing, for
example, zinc finger nucleases (Orlando et al., 2010) [45] or
TALENs (Cristea et al., 2013) [22] which can make double-strand
breaks (DSBs) that leave 5' overhangs or "blunt-end" employing
CRISP/Cas9 ribonucleoprotein. An example of nuclease gene
integration by ligation using I-SceI meganuclease was shown by the
construction of vector pD7-Sce1. pD7 is derived from pD6 (FIG. 8)
but the left and right AAVS homology arms were replaced with short
double stranded oligonucleotides. The left AAVS homology arm of the
pD vector series is flanked by EcoR1 and Nsi1 restriction enzymes
(see FIG. 3). To convert pD6 to pD7-Sce1, this was replaced by a
double-stranded oligonucleotide insert formed by primers 2778 and
2779 encoding an I-SceI meganuclease recognition sequence with
"sticky ends" compatible with the "sticky ends" formed by
EcoRI/NsiI. The right hand AAVS homology arm is flanked by Asc1 and
Mlu1 restriction sites (FIG. 3). The right homology arm was
replaced by a double-stranded oligonucleotide insert with "sticky
ends" compatible with the "sticky ends" formed by AscI/MluI
digestion and is formed by primers 2723 and 2724.
TABLE-US-00025 TABLE 10 Primers for pD7 and pINT19 construction
2723 CGCGCCAGAAGTCTCACCAAGCCCA (SEQ ID NO: 121) 2724
CGCGTGGGCTTGGTGAGACTTCTGG (SEQ ID NO: 122) 2768
AATTCTCCCCTCCACCCCACAGTAGGGA (SEQ ID NO: 123)
CAGTGGGGCCAGGATTGGTGACAGAAAA TGCA 2769 TTTTCTGTCACCAATCCTGGCCCCACTG
(SEQ ID NO: 124) TCCCTACTGTGGGGTGGAGGGGAG 2778
AATTCTAGGGATAACAGGGTAATATGCA (SEQ ID NO: 125) 2779
TATTACCCTGTTATCCCTAG (SEQ ID NO: 126) 2808
AATTCTTTTCTGTCACCAATCCTGGGGC (SEQ ID NO: 127)
CACTAGGGACACTGTGGGGTGGAGGGGA TGCA 2809 TCCCCTCCACCCCACAGTGTCCCTAGTG
(SEQ ID NO: 128) GCCCCAGGATTGGTGACAGAAAAGAATT G
[0447] Antibodies recognising Fgfr1 and Fgfr2 (example 15) were
cloned into pD7 to create pD7-SceI anti-Fgfr1 and pD7-SceI
anti-Fgfr2 respectively. These were co-transfected with the I-SceI
expression plasmid (example 11, FIG. 16) into the HEK293 clone 6F
cell line (see example 11) which contains an integrated I-SceI
recognition site.
[0448] Ligation of DSBs in the chromosome and targeting vector
generated by zinc finger nuclease or TALE nucleases can also be
achieved. By inverting the zinc finger nuclease or TALEN
recognition sites on the targeting vector this can ensure that the
product of the insertion is no longer a target for cleavage in a
method termed "Obligate ligation-gated recombination" or ObLiGaRe
(Maresca et al., 2013) [153]. pD7-ObLiGaRe vectors can be generated
in the same way as described above for the creation of pD7-Scel. In
this case, the left hand homology arm is replaced by an
oligonucleotide consisting of primers 2808 and 2809 encoding an
inverted TALEN recognition site (shown in bold) and spacer region.
The right hand homology arm is replaced with primers 2723 and 2809
as described above.
[0449] An alternative to simple ligation reactions between DSBs in
the chromosome and targeting vector, mediated by non-homologous end
joining (NHEJ) is microhomology-mediated end-joining (MMEJ). MMEJ
is a DSB repair mechanism that uses microhomologous sequences
between 5 to 25 bp for error-prone end joining (McVey and Lee,
2008) [154]. A strategy for precise gene integration has been
devised where the genomic sequence and the targeting vector contain
the same TALE nuclease pair recognition sequence, but a different
vector spacer sequence in which the anterior and posterior half are
switched. The genomic sequence and vector can be cut by the same
TALEN pair and MMEJ takes place via the microhomologous DNA ends.
The resultant integrated targeting vector is no longer a target for
TALE nuclease because of the shortened spacer region which are not
optimal for TALE nuclease cleavage (Nakade et al., 2014) [155].
[0450] The MMEJ AAVS targeting vector pD7-MMEJ can be generated in
the same way as described above for the creation of pD7-Scel. In
this case the left hand homology arm is replaced by an
oligonucleotide consisting of primers 2768 and 2769 encoding the
TALEN recognition site (shown in bold) and switched spacer region
(underlined). The right hand homology arm is replaced with primers
2723 and 2809 as described above.
Example 22: Design of Primers for Creation of Single (CMV) Promoter
Cassette Flanked by ROSA26 Arms (pINT19-ROSA)
[0451] This example is intended to demonstrate that antibody or
alternative binding molecule genes can be integrated into the
genome of mammalian cells by nuclease directed methods and the
resultant clones screened for a desired function by either reporter
or phenotypic screening. An example of this was previously
demonstrated where antibody genes were integrated into the
chromosome of mouse embryonic stem (ES) cells and individual ES
colonies screened for their ability to maintain pluripotency when
subjected to differentiation conditions [105]. Antibody genes
recovered from ES colonies which maintained a pluripotent phenotype
were shown to block the FGFR1/FGF4 signaling pathway. A problem
with this previously reported method is that homologous
recombination can result in small library sizes, thus limiting its
ability to directly screen for rare clones present in large binding
molecule libraries. Nuclease-mediated gene integration methods for
antibody and binding molecule gene integration are more efficient
resulting in larger library size generation and thus more likely to
generate mammalian cell libraries capable of identifying functional
antibodies by phenotypic or reporter cell screening.
[0452] The donor targeting vector pINT 19 is designed to integrate
antibody genes into the mouse ROSA26 locus by nuclease directed
methods for direct functional screening. pINT19 is a single CMV
promoter vector for scFv-Fc fusion expression. The expression
cassette is flanked by mouse ROSA26 arms. Since the upstream exon
is untranslated, the puromycin gene is preceded by a splice
acceptor and further down has a KOZAK sequence leading into the
puromycin gene.
[0453] The AAVS left homology arm and puromycin resistance gene of
pINT 18 was replaced by a cassette encoding the ROSA26 left
homology, splice acceptor, optimized kozak consensus sequence and
puromycin resistance gene. The ROSA26 left homology arm was
initially amplified from pGATOR (Melidoni et al., 2013 (105)) as
two fragments which knocked out an internal NotI site. The two
fragments, generated by primers J60/2716 and 2715/2706 were
combined in an assembly PCR with primers J60 and 2706 and digested
with AsiSI and NsiI. The splice acceptor was amplified from pGATOR
using primers 2709 and 2710 and the puromycin resistance cassette
amplified with primers 2745 (which included a region homologous to
the splice acceptor and optimized Kozak consensus) and J59. The
splice acceptor region and puromycin resistance cassette were
combined in assembly PCR using primers 2709 and J59 and digested
with Nsi1 and Bgl2. The ROSA26 left arm homology and splice
acceptor-puromycin cassettes were ligated with pINT 18 (AsiS
1/Bgl2) vector.
[0454] To complete the ROSA targeting vector the right hand ROSA26
homology arm, downstream of CMV-scFv-Fc cassette, was introduced to
replace the pINT18 AAVS right homology arm. This was performed by
PCR of the ROSA right homology arm, present in pGATOR (Melidoni et
al., 2013) using primers J61 and J62 to amplify a fragment with
BstZ171 at one end and Sbf1 at the other. Primer 61 was positioned
to exclude an endogenous Sbf1 site 65 bp up from ROSA ZFN cleavage
site. FIG. 31 shows the sequences of ROSA26 left and right homology
arms.
TABLE-US-00026 TABLE 11 Primers for nuclease-directed targeting of
the mouse ROSA 26 locus J60 ACACACGGTACCGCGATCGCGCTGATT
AsiSI-rosa26-L-F (SEQ ID NO: 129) GGCTTCTTTTCCTC 2706
TTTTTTATGCATTCTAGAAAGACTGGA NsiI-rosa26-L-R (SEQ ID NO: 130)
GTTGCAGA 2715 GAGCGTCCGCCCACCCTC ROSA-Left- (SEQ ID NO: 131)
NotI_knockout_F 2716 GAGGGTGGGCGGACGCTC ROSA-Left- (SEQ ID NO: 132)
NotI_knockout_R 2709 TTTTTTATGCATTAAGGGATCTGTAGG Splice_acceptor-
(SEQ ID NO: 133) GCGCAG F-NsiI 2710 GTGAATTCCTAGAGCGGCCTC
Splice_acceptor- (SEQ ID NO: 134) R 2745
GAGGCCGCTCTAGGAATTCACGCCGCC Overlap-Puro- (SEQ ID NO: 135)
ACCATGACCGAGTACAAGCCCAC F+kozak J59 AAAAAAAGATCTGTGTGTTTCGAATCA
Bgl2-Puro-R (SEQ ID NO: 136) GGCACCGGGCTTGCGGGTCAT J61
ttttttGTATACGGGAATTGAACAGGT ROSA-Right_F- (SEQ ID NO: 137)
GTAAAATTG BstZ171 J62 TTTTTTCCTGCAGGAGGTTGGATTCTC ROSA-Right_R-
(SEQ ID NO: 138) AATACATCTATTGTTG SbfI 2701
GCCGACGTCTCGTCGCTGATGTTTT (SEQ ID NO: 139) 2702
ATCAGCGACGAGACGTCGGCCGGTG (SEQ ID NO: 140) 2703
CGCCCATCTTCTAGAAAGACGTTTT (SEQ ID NO: 141) 2704
GTCTTTCTAGAAGATGGGCGCGGTG (SEQ ID NO: 142)
[0455] Integration of pINT19, encoding antibody or alternative
binding molecules, into the mouse ROSA26 locus could be achieved by
nuclease-directed introduction of antibody libraries using
CRISPR/Cas9 as described in Example 20. Here, nuclease-directed
integration via CRISPR/Cas9 could be demonstrated using the
"Geneart CRISPR nuclease vector kit" (Lifetech A21175). In this
system, a U6 RNA polymerase III promoter drives expression of a
target complementary CRIPSR RNA (crRNA) which is linked to a
trans-activating crRNA (tracrRNA). The crRNA and tracrRNA together
make up a guide RNA which directs the cleavage specificity of a
Cas9 protein encoded on the same "GeneArt CRISPR nuclease vector"
(see manufacturer's instructions). The vector is provided as a
linearised plasmid into which a short double-stranded
oligonucleotide with appropriate 3' overhangs is cloned. Cleavage
specificity is then determined by the sequence of the cloned
segment. 2 different targeting sequences were designed to direct
cleavage to the mouse ROSA26 encoded by primers 2701/2702 and
2703/2704 (see Table 10).
[0456] As an alternative, Zinc finger nucleases which cleave within
the ROSA26 locus have been described [34]. These could be
constructed in an appropriate expression vector as described for
Sce-I meganuclease (FIG. 16, example 11).
[0457] Nuclease-mediated integration of donor plasmid pINT 19 would
give rise to clones expressing secreted antibody which could bind
endogenous receptor or ligand resulting in either antagonism
[105,107] or agonism [47,106,108] of receptor signalling pathways.
To enable the linkage between cellular phenotype and the functional
activity of the secreted antibody, cells can be plated at a low
density in semi-solid media so that individual colonies propagate
and antibody expression can be initiated via an inducible promoter
[105]. Alternatively, a constitutive promoter could be employed for
antibody gene expression. The semi-solid media would maintain an
elevated local concentration of the endogenously-expressed
antibody, so that any phenotypic change specific to a colony
arising from an individual cell would be caused by the unique
antibody expressed from that particular clone. If a rapid response
reporter, such as Rex or Nanog promoter fused to a reporter gene,
was employed it would be possible to plate cells at a low density
in semi-solid media, harvest and then screen by flow cytometry.
Alternatively, the stop codon downstream of the antibody gene in
pINT 19 could be replaced by a transmembrane domain to enable
tethering of the antibody to the cell surface. The stop codon
downstream of the antibody gene in pINT19 could also be replaced by
an endoplasmic reticulum (ER) retention signal sequence to enable
retention of antibodies in the ER and potential down-regulation of
an endogenously expressed target receptor or any secreted protein
or peptide. pINT19 is designed specifically to target the mouse
ROSA26 locus and can be employed for phenotypic screening of
antibodies or alternative binding molecules in mouse ES cells.
However, nuclease-directed antibody or binder molecule gene
integration methods could also be applied to other functional
screens such as those described using the lentiviral approach
[47,106,107,108].
REFERENCES
[0458] All references listed below and others cited anywhere in
this disclosure are incorporated herein by reference in their
entirety. [0459] 1 Russell, S. J., Hawkins, R. E., & Winter, G.
(1993). Retroviral vectors displaying functional antibody
fragments. Nucleic Acids Res, 21(5), 1081-1085. [0460] 2 Boublik,
Y., Di Bonito, P., & Jones, I. M. (1995). Eukaryotic virus
display: Engineering the major surface glycoprotein of the
Autographa californica nuclear polyhedrosis virus (AcNPV) for the
presentation of foreign proteins on the virus surface. Nature
Biotechnology, 13(10), 1079-1084. [0461] 3 Mottershead, D. G.,
Alfthan, K., Ojala, K., Takkinen, K., & Oker-Blom, C. (2000).
Baculoviral display of functional scFv and synthetic IgG-binding
domains. Biochemical and Biophysical Research Communications,
275(1), 84-90. [0462] 4 Oker-Blom, C., Airenne, K. J., &
Grabherr, R. (2003). Baculovirus display strategies: Emerging tools
for eukaryotic libraries and gene delivery. Briefings in Functional
Genomics and Proteomics, 2(3), 244-253. [0463] 5 Edwards, B. M.,
Barash, S. C., Main, S. H., Choi, G. H., Minter, R., Ullrich, S.,
et al. (2003). The Remarkable Flexibility of the Human Antibody
Repertoire; Isolation of Over One Thousand Different Antibodies to
a Single Protein, BLyS. Journal of Molecular Biology, 334(1),
103-118. doi: 10.1016/j.jmb.2003.09.054 [0464] 6 Pershad, K.,
Pavlovic, J. D., Graslund, S., Nilsson, P., Colwill, K.,
Karatt-Vellatt, A., et al. (2010). Generating a panel of highly
specific antibodies to 20 human SH2 domains by phage display.
Protein Engineering, Design and Selection, 23(4), 279-288.
doi:10.1093/protein/gzq003 [0465] 7 Schofield, D. J., Pope, A. R.,
Clementel, V., Buckell, J., Chapple, S., Clarke, K. F., et al.
(2007). Application of phage display to high throughput antibody
generation and characterization. Genome Biol, 8(11), R254. [0466] 8
Salema, V., Marin, E., Martinez-Arteaga, R., Ruano-Gallego, D.,
Fraile, S., Margolles, Y., et al. (2013). Selection of Single
Domain Antibodies from Immune Libraries Displayed on the Surface of
E. coli Cells with Two 13-Domains of Opposite Topologies. PLoS ONE,
8(9), e75126. doi: 10.1371/journal.pone.0075126.s007 [0467] 9 Chao,
G., Lau, W. L., Hackel, B. J., Sazinsky, S. L., Lippow, S. M.,
& Wittrup, K. D. (2006). Isolating and engineering human
antibodies using yeast surface display. Nat Protoc, 1(2), 755-768.
[0468] 10 Higuchi, K., Araki, T., Matsuzaki, O., Sato, A., Kanno,
K., Kitaguchi, N., & I to, H. (1997). Cell display library for
gene cloning of variable regions of human antibodies to hepatitis B
surface antigen. J Immunol Methods, 202(2), 193-204. [0469] 11 Ho,
M., Nagata, S., & Pastan, I. (2006). Isolation of anti-CD22 Fv
with high affinity by Fv display on human cells. Proc Natl Acad Sci
USA, 103(25), 9637-9642. doi: 10.1073/pnas.0603653103 [0470] 12 Ho,
M., & Pastan, I. (2009). Display and selection of scFv
antibodies on HEK-293T cells. Methods Mol Biol, 562, 99-113.
doi:10.1007/978-1-60327-302-2_8 [0471] 13 Akamatsu, Y., Pakabunto,
K., Xu, Z., Zhang, Y., & Tsurushita, N. (2007). Whole IgG
surface display on mammalian cells: Application to isolation of
neutralizing chicken monoclonal anti-IL-12 antibodies. Journal of
Immunological Methods, 327(1-2), 40-52. doi:
10.1016/j.jim.2007.07.007 [0472] 14 Beerli, R. R., Bauer, M.,
Buser, R. B., Gwerder, M., Muntwiler, S., Maurer, P., et al.
Isolation of human monoclonal antibodies by mammalian cell display.
Proc Natl Acad Sci USA. 2008 Sep. 23; 105(38):14336-41. doi:
10.1073/pnas.0805942105 [0473] 15 Breous-Nystrom, E., Schultze, K.,
Meier, M., Flueck, L., Holzer, C., Boll, M., et al. (2013).
Retrocyte DisplayA.RTM. technology: Generation and screening of a
high diversity cellular antibody library. Methods, 1-11. doi:
10.1016/j.ymeth.2013.09.003 [0474] 16 Grindley, Whiteson &
Rice. Mechanisms of Site-Specific Recombination. Annu Rev Biochem
2006 75:567-605 [0475] 17 Zhou, C., Jacobsen, F. W., Cai, L., Chen,
Q., & Shen, W. D. (2010). Development of a novel mammalian cell
surface antibody display platform. mAbs, 2(5), 508-518 [0476] 18
Li, C. Z., Liang, Z. K., Chen, Z. R., Lou, H. B., Zhou, Y., Zhang,
Z. H., et al. (2012). Identification of HBsAg-specific antibodies
from a mammalian cell displayed full-length human antibody library
of healthy immunized donor. Cellular and Molecular Immunology,
9(2), 184-190. [0477] 19 Buchholz, F., Ringrose, L., Angrand, P.
O., Rossi, F., & Stewart, A. F. (1996). Different
thermostabilities of FLP and Cre recombinases: Implications for
applied site-specific recombination. Nucleic Acids Res, 24(21),
4256-4262. [0478] 20 Schaft, J., Ashery-Padan, R., Van Hoeven, F.
D., Gruss, P., & Francis Stewart, A. (2001). Efficient FLP
recombination in mouse ES cells and oocytes. Genesis, 31(1), 6-10.
[0479] 21 Moehle, E. A., Moehle, E. A., Rock, J. M., Rock, J. M.,
Lee, Y.-L., Lee, Y. L., et al. (2007). Targeted gene addition into
a specified location in the human genome using designed zinc finger
nucleases. Proc Natl Acad Sci USA, 104(9), 3055-3060.
doi:10.1073/pnas.0611478104 [0480] 22 Cristea, S., Freyvert, Y.,
Santiago, Y., Holmes, M. C., Urnov, F. D., Gregory, P. D., &
Cost, G. J. (2013). In vivo cleavage of transgene donors promotes
nuclease-mediated targeted integration. Biotechnology and
Bioengineering, 110(3), 871-880. doi: 10.1002/bit.24733 [0481] 23
Letourneur, F., & Malissen, B. (1989). Derivation of a T cell
hybridoma variant deprived of functional T cell receptor alpha and
beta chain transcripts reveals a nonfunctional alpha-mRNA of BW5147
origin. European Journal of Immunology, 19(12), 2269-2274.
doi:10.1002/eji. 1830191214 [0482] 24 Kanayama, N., Todo, K., Reth,
M., & Ohmori, H. (2005). Reversible switching of immunoglobulin
hypermutation machinery in a chicken B cell line. Biochem Biophys
Res Commun, 327(1), 70-75. doi:10.1016/j.bbrc.2004.11.143 [0483] 25
Lin, W., Kurosawa, K., Murayama, A., Kagaya, E., & Ohta, K.
(2011). B-cell display-based one-step method to generate chimeric
human IgG monoclonal antibodies. Nucleic Acids Research, 39(3),
e14-e14. doi:10.1093/nar/gkq1122 [0484] 26 Adachi, N., So, S.,
Iiizumi, S., Nomura, Y., Murai, K., Yamakawa, C., et al. (2006).
The human pre-B cell line Nalm-6 is highly proficient in gene
targeting by homologous recombination. DNA and Cell Biology, 25(1),
19-24. doi:10.1089/dna.2006.25.19 [0485] 27 Palacios, R., &
Steinmetz, M. (1985). IL3-Dependent mouse clones that express B-220
surface antigen, contain Ig genes in germ-line configuration, and
generate B lymphocytes in vivo. Cell, 41(3), 727-734 [0486] 28
Barretina, J., Caponigro, G., Stransky, N., Venkatesan, K.,
Margolin, A. A., Kim, S., et al. (2012). The Cancer Cell Line
Encyclopedia enables predictive modelling of anticancer drug
sensitivity. Nature, 483(7391), 603-607. doi:10.1038/nature 11003
[0487] 29 Forbes, S. A., Bindal, N., Bamford, S., Cole, C., Kok, C.
Y., Beare, D., et al. (2011). COSMIC: mining complete cancer
genomes in the Catalogue of Somatic Mutations in Cancer. Nucleic
Acids Research, 39(Database issue), D945-50. doi:10.1093/nar/gkq929
[0488] 30 Silva, G., Poirot, L., Galetto, R., Smith, J., Montoya,
G., Duchateau, P., & Paques, F. (2011). Meganucleases and other
tools for targeted genome engineering: Perspectives and challenges
for gene therapy. Current Gene Therapy, 11(1), 11-27 [0489] 31
Epinat, J. C., Silva, G. H., Paques, F., Smith, J., &
Duchateau, P. (2013). Engineered meganucleases for genome
engineering purposes. Topics in Current Genetics (Vol. 23, pp.
147-185). [0490] 32 Szczepek, M., Brondani, V., Bichel, J.,
Serrano, L., Segal, D. J., & Cathomen, T. (2007).
Structure-based redesign of the dimerization interface reduces the
toxicity of zinc-finger nucleases. Nature Biotechnology, 25(7),
786-793. doi: 10.1038/nbt1317 [0491] 33 Doyon, Y., Vo, T. D.,
Mendel, M. C., Greenberg, S. G., Wang, J., Xia, D. F., et al.
(2011). Enhancing zinc-finger-nuclease activity with improved
obligate heterodimeric architectures. Nat Methods, 8(1), 74-79.
doi: 10.1038/nmeth. 1539 [0492] 34 Perez-Pinera, P., Ousterout, D.
G., Brown, M. T., & Gersbach, C. A. (2012). Gene targeting to
the ROSA26 locus directed by engineered zinc finger nucleases.
Nucleic Acids Research, 40(8), 3741-3752. doi:10.1093/nar/gkr1214
[0493] 35 Urnov, F. D., Miller, J. C., Lee, Y.-L., Beausejour, C.
M., Rock, J. M., Augustus, S., et al. (2005). Highly efficient
endogenous human gene correction using designed zinc-finger
nucleases. Nature, 435(7042), 646-651. doi:10.1038/nature03556
[0494] 36 Maresca, M., Lin, V. G., Guo, N., & Yang, Y. (2013).
Obligate ligation-gated recombination (ObLiGaRe): custom-designed
nuclease-mediated targeted integration through nonhomologous end
joining. Genome Research, 23(3), 539-546. doi:10.1101/gr.145441.112
[0495] 37 Bogdanove, A. J., & Voytas, D. F. (2011). TAL
effectors: customizable proteins for DNA targeting. Science,
333(6051), 1843-1846. doi:10.1126/science. 1204094 [0496] 38 Reyon,
D., Tsai, S. Q., Khgayter, C., Foden, J. A., Sander, J. D., &
Joung, J. K. (2012). FLASH assembly of TALENs for high-throughput
genome editing. Nature Biotechnology, 30(5), 460-465.
doi:10.1038/nbt.2170 [0497] 39 Boissel, S., Jarjour, J., Astrakhan,
A., Adey, A., Gouble, A., Duchateau, P., et al. (2013). megaTALs: a
rare-cleaving nuclease architecture for therapeutic genome
engineering. Nucleic Acids Research. doi:10.1093/nar/gkt1224 [0498]
40 Beurdeley, M., Bietz, F., Li, J., Thomas, S., Stoddard, T.,
Juillerat, A., et al. (2013). Compact designer TALENs for efficient
genome engineering. Nature Communications, 4, 1762. doi:
10.1038/ncomms2782 [0499] 41 Sampson, T. R., & Weiss, D. S.
(2014). Exploiting CRISPR/Cas systems for biotechnology. BioEssays:
News and Reviews in Molecular, Cellular and Developmental Biology,
36(1), 34-38. doi: 10.1002/bies.201300135 [0500] 42 Shalem, O.,
Sanjana, N. E., Hartenian, E., Shi, X., Scott, D. A., Mikkelsen, T.
S., et al. (2014). Genome-scale CRISPR-Cas9 knockout screening in
human cells. Science, 343(6166), 84-87. doi: 10.1126/science.
1247005 [0501] 43 Wang, T., Wei, J. J., Sabatini, D. M., &
Lander, E. S. (2014). Genetic screens in human cells using the
CRISPR-Cas9 system. Science, 343(6166), 80-84. doi:10.1126/science.
1246981 [0502] 44 Beard, C., Hochedlinger, K., Plath, K., Wutz, A.,
& Jaenisch, R. (2006). Efficient method to generate single-copy
transgenic mice by site-specific integration in embryonic stem
cells. Genesis, 44(1), 23-28. [0503] 45 Orlando, S. J., Santiago,
Y., DeKelver, R. C., Freyvert, Y., Boydston, E. A., Moehle, E. A.,
et al. (2010). Zinc-finger nuclease-driven targeted integration
into mammalian genomes using donors with limited chromosomal
homology. Nucleic Acids Research, 38(15), e152.
doi:10.1093/nar/gkq512 [0504] 46 Cadinanos, J; Bradley, A. (2007)
Nucleic Acids Res. 35(12):e87 [0505] 47 Zhang, H., Yea, K., Xie,
J., Ruiz, D., Wilson, I. A., & Lerner, R. A. (2013). Selecting
agonists from single cells infected with combinatorial antibody
libraries. Chemistry & Biology, 20(5), 734-741.
doi:10.1016/j.chembiol.2013.04.012 [0506] 48 Porteus, M. H., &
Baltimore, D. (2003). Chimeric nucleases stimulate gene targeting
in human cells. Science, 300(5620), 763. doi:10.1126/science.
1078395 [0507] 49 Rouet, P., Smih, F., & Jasin, M. (1994).
Introduction of double-strand breaks into the genome of mouse cells
by expression of a rare-cutting endonuclease. Molecular and
Cellular Biology, 14(12), 8096-8106. [0508] 50 Jasin, M. (1996).
Genetic manipulation of genomes with rare-cutting endonucleases.
Trends in Genetics, 12(6), 224-228 [0509] 51 Davis, L., &
Maizels, N. (2011). DNA nicks promote efficient and safe targeted
gene correction. PLoS ONE, 6(9), e23981.
doi:10.1371/journal.pone.0023981 [0510] 52 Fujioka, K., Aratani,
Y., Kusano, K., & Koyama, H. (1993). Targeted recombination
with single-stranded DNA vectors in mammalian cells. Nucleic Acids
Res, 21(3), 407-412 [0511] 53 Khan, I. F., Hirata, R. K., &
Russell, D. W. (2011). AAV-mediated gene targeting methods for
human cells. Nat Protoc, 6(4), 482-501. doi: 10.1038/nprot.2011.301
[0512] 54 Deyle, D. R., & Russell, D. W. (2009).
Adeno-associated virus vector integration. Current Opinion in
Molecular Therapeutics, 11(4), 442-447 [0513] 55 Benatuil, L.,
Perez, J. M., Belk, J., & Hsieh, C. M. (2010). An improved
yeast transformation method for the generation of very large human
antibody libraries. Protein Engineering, Design and Selection,
23(4), 155-159. [0514] 56 Feldhaus, M. J., Siegel, R. W., Opresko,
L. K., Coleman, J. R., Feldhaus, J. M., Yeung, Y. A., et al.
(2003). Flow-cytometric isolation of human antibodies from a
nonimmune Saccharomyces cerevisiae surface display library. Nat
Biotechnol. [0515] 57 Zhao, A., Nunez-Cruz, S., Li, C., Coukos, G.,
Siegel, D. L., & Scholler, N. (2011). Rapid isolation of
high-affinity human antibodies against the tumor vascular marker
Endosialin/TEM1, using a paired yeast-display/secretory scFv
library platform. Journal of Immunological Methods, 363(2),
221-232. doi:10.1016/j.jim.2010.09.001 [0516] 58 Skerra, A. (2007).
Alternative non-antibody scaffolds for molecular recognition. Curr
Opin Biotechnol, 18(4), 295-304. doi:10.1016/j.copbio.2007.04.010
[0517] 59 Gebauer, M., & Skerra, A. (2009). Engineered protein
scaffolds as next-generation antibody therapeutics. Current Opinion
in Chemical Biology, 13(3), 245-255. doi:
10.1016/j.cbpa.2009.04.627 [0518] 60 Haan & Maggos (2004)
BioCentury, 12(5): A1-A6 [0519] 61 Koide et al. (1998) Journal of
Molecular Biology, 284: 1141-1151. [0520] 62 Nygren et al. (1997)
Current Opinion in Structural Biology, 7: 463-469 [0521] 63 Wess,
L. In: BioCentury, The Bernstein Report on BioBusiness, 12(42),
A1-A7, 2004 [0522] 64 Chang, H.-J., Hsu, H.-J., Chang, C.-F., Peng,
H.-P., Sun, Y.-K., Yu, H.-M., et al. (2009). Molecular Evolution of
Cystine-Stabilized Miniproteins as Stable Proteinaceous Binders.
Structure, 17(4), 620-631. doi: 10.1016/j.str.2009.01.011 [0523] 65
Ward, E. S. et al., Nature 341, 544-546 (1989) [0524] 66 McCafferty
et al Nature, 348, 552-554 (1990) [0525] 67 Holt et al Trends in
Biotechnology 21, 484-490 (2003) [0526] 68 Bird et al, Science,
242, 423-426, (1988) [0527] 69 Huston et al, PNAS USA, 85,
5879-5883, (1988) [0528] 70 Holliger, P. et al, PNAS USA 90
6444-6448, (1993) [0529] 71 Reiter, Y. et al, Nature Biotech, 14,
1239-1245, (1996) [0530] 72 Holliger & Hudson, Nature
Biotechnology 23(9): 1126-1136 (2005) [0531] 73 Knappik et al. J.
Mol. Biol. 296, 57-86 (2000) [0532] 74 Krebs et al. Journal of
Immunological Methods 254, 67-84 (2001) [0533] 75 Holliger and
Bohlen Cancer and metastasis rev. 18: 411-419 (1999) [0534] 76
Holliger, P. and Winter G. Current Opinion Biotechnol 4, 446-449
(1993) [0535] 77 Glennie M J et al., J. Immunol. 139, 2367-2375
(1987) [0536] 78 Repp R. et al., J. Hemat. 377-382 (1995) [0537] 79
Staerz U. D. and Bevan M. J. PNAS 83 (1986) [0538] 80 Suresh M. R.
et al.,
Method Enzymol. 121: 210-228 (1986) [0539] 81 Merchand et al.,
Nature Biotech. 16:677-681 (1998) [0540] 82 Ridgeway, J. B. B. et
al, Protein Eng., 9, 616-621, (1996) [0541] 83 Marks, J. D.,
Hoogenboom, H. R., Bonnert, T. P., McCafferty, J., Griffiths, A.
D., & Winter, G. (1991). By-passing immunization. Human
antibodies from V-gene libraries displayed on phage. J Mol Biol,
222(3), 581-597. [0542] 84 Gronwald, R. G. K., Grant, F. J.,
Haldeman, B. A., Hart, C. E., O'Hara, P. J., Hagen, F. S., et al.
(1988). Cloning and expression of a cDNA coding for the human
platelet-derived growth factor receptor: Evidence for more than one
receptor class (Vol. 85, pp. 3435-3439). Presented at the
Proceedings of the National Academy of Sciences of the United
States of America. [0543] 85 Kumar, N., & Borth, N. (2012).
Flow-cytometry and cell sorting: an efficient approach to
investigate productivity and cell physiology in mammalian cell
factories. Methods, 56(3), 366-374. doi:10.1016/j.ymeth.2012.03.004
[0544] 86 Brezinsky, S. C. G., Chiang, G. G., Szilvasi, A., Mohan,
S., Shapiro, R. I., MacLean, A., et al. (2003). A simple method for
enriching populations of transfected CHO cells for cells of higher
specific productivity. J Immunol Methods, 277(1-2), 141-155. [0545]
87 Pichler, J., Hesse, F., Wieser, M., Kunert, R., Galosy, S. S.,
Mott, J. E., & Borth, N. (2009). A study on the temperature
dependency and time course of the cold capture antibody secretion
assay. Journal of Biotechnology, 141(1-2), 80-83.
doi:10.1016/j.jbiotec.2009.03.001 [0546] 88 Anastassiadis, K., Fu,
J., Patsch, C., Hu, S., Weidlich, S., Duerschke, K., et al. (2009).
Dre recombinase, like Cre, is a highly efficient site-specific
recombinase in E. coli, mammalian cells and mice. Disease Models
& Mechanisms, 2(9-10), 508-515. doi: 10.1242/dmm.003087 [0547]
89 Horlick, R. A., Macomber, J. L., Bowers, P. M., Neben, T. Y.,
Tomlinson, G. L., Krapf, I. P., et al. (2013). Simultaneous surface
display and secretion of proteins from mammalian cells facilitate
efficient in vitro selection and maturation of antibodies. J Biol
Chem, 288(27), 19861-19869. doi:10.1074/jbc.M113.452482 [0548] 90
Biffi, G., Tannahill, D., McCafferty, J., & Balasubramanian, S.
(2013). Quantitative visualization of DNA G-quadruplex structures
in human cells. Nature Chemistry, 5(3), 182-186. doi:
10.1038/nchem. 1548 [0549] 91 Gao, J., Sidhu, S. S., & Wells,
J. A. (2009). Two-state selection of conformation-specific
antibodies. Proc Natl Acad Sci US A, 106(9), 3071-3076.
doi:10.1073/pnas.0812952106 [0550] 92 Gu, G. J., Friedman, M.,
Jost, C., Johnsson, K., Kamali-Moghaddam, M., Pluckthun, A., et al.
(2013). Protein tag-mediated conjugation of oligonucleotides to
recombinant affinity binders for proximity ligation. N Biotechnol,
30(2), 144-152. doi: 10.1016/j.nbt.2012.05.005 [0551] 93 Cho, Y.
K., & Shusta, E. V. (2010). Antibody library screens using
detergent-solubilized mammalian cell lysates as antigen sources.
Protein Eng Des Sel, 23(7), 567-577. doi:10.1093/protein/gzq029
[0552] 94 Tillotson, B. J., Cho, Y. K., & Shusta, E. V. (2013).
Cells and cell lysates: a direct approach for engineering
antibodies against membrane proteins using yeast surface display.
Methods, 60(1), 27-37. doi:10.1016/j.ymeth.2012.03.010 [0553] 95
Kunert, A., Straetemans, T., Govers, C., Lamers, C., Mathijssen,
R., Sleijfer, S., & Debets, R. (2013). TCR-Engineered T Cells
Meet New Challenges to Treat Solid Tumors: Choice of Antigen, T
Cell Fitness, and Sensitization of Tumor Milieu. Frontiers in
Immunology, 4, 363. doi: 10.3389/fimmu.2013.00363 [0554] 96 Liddy,
N., Bossi, G., Adams, K. J., Lissina, A., Mahon, T. M., Hassan, N.
J., et al. (2012). Monoclonal TCR-redirected tumor cell killing.
Nature Medicine, 18(6), 980-987. doi: 10.1038/nm.2764 [0555] 97
Holler, P. D., Holman, P. O., Shusta, E. V., O'Herrin, S., Wittrup,
K. D., & Kranz, D. M. (2000). In vitro evolution of a T cell
receptor with high affinity for peptide/MHC. Proc Natl Acad Sci
USA, 97(10), 5387-5392. doi:10.1073/pnas.080078297 [0556] 98 Weber,
K. S., Donermeyer, D. L., Allen, P. M., & Kranz, D. M. (2005).
Class II-restricted T cell receptor engineered in vitro for higher
affinity retains peptide specificity and function. Proc Natl Acad
Sci USA, 102(52), 19033-19038. doi:10.1073/pnas.0507554102 [0557]
99 Kessels, H. W., van Den Boom, M. D., Spits, H., Hooijberg, E.,
& Schumacher, T. N. (2000). Changing T cell specificity by
retroviral T cell receptor display. Proc Natl Acad Sci USA, 97(26),
14578-14583. doi:10.1073/pnas.97.26.14578 [0558] 100 Chervin, A.
S., Aggen, D. H., Raseman, J. M., & Kranz, D. M. (2008).
Engineering higher affinity T cell receptors using a T cell display
system. Journal of Immunological Methods, 339(2), 175-184. [0559]
101 Crawford, F., Jordan, K. R., Stadinski, B., Wang, Y., Huseby,
E., Marrack, P., et al. (2006). Use of baculovirus MHC/peptide
display libraries to characterize T-cell receptor ligands.
Immunological Reviews, 210, 156-170.
doi:10.1111/j.0105-2896.2006.00365.x [0560] 102 Hinrichs, C. S.,
& Restifo, N. P. (2013). Reassessing target antigens for
adoptive T-cell therapy. Nat Biotechnol, 31(11), 999-1008. doi:
10.1038/nbt.2725 [0561] 103 Sadelain, M., Brentjens, R., &
Riviere, I. (2013). The basic principles of chimeric antigen
receptor design. Cancer Discovery, 3(4), 388-398.
doi:10.1158/2159-8290.CD-12-0548 [0562] 104 Alonso-Camino, V.,
Sanchez-Martin, D., Compte, M., Sanz, L., & Alvarez-Vallina, L.
(2009). Lymphocyte display: a novel antibody selection platform
based on T cell activation. PLoS ONE, 4(9), e7174.
doi:10.1371/journal.pone.0007174 [0563] 105 Melidoni, A. N., Dyson,
M. R., Wormald, S., & McCafferty, J. (2013). Selecting
antagonistic antibodies that control differentiation through
inducible expression in embryonic stem cells. Proceedings of the
National Academy of Sciences, 110(44), 17802-17807. doi:
10.1073/pnas.1312062110 [0564] 106 Zhang, H., Wilson, I. A., &
Lerner, R. A. (2012). Selection of antibodies that regulate
phenotype from intracellular combinatorial antibody libraries.
Proceedings of the National Academy of Sciences, 109(39),
15728-15733. doi:10.1073/pnas.1214275109 [0565] 107 Xie, J., Yea,
K., Zhang, H., Moldt, B., He, L., Zhu, J., & Lerner, R. A.
(2014). Prevention of cell death by antibodies selected from
intracellular combinatorial libraries. Chemistry & Biology,
21(2), 274-283. [0566] 108 Yea, K., Zhang, H., Xie, J., Jones, T.
M., Yang, G., Song, B. D., & Lerner, R. A. (2013). Converting
stem cells to dendritic cells by agonist antibodies from unbiased
morphogenic selections (Vol. 110, pp. 14966-14971). Presented at
the Proceedings of the National Academy of Sciences of the United
States of America. doi: 10.1073/pnas.1313671110 [0567] 109
Kawahara, M., Kimura, H., Ueda, H., & Nagamune, T. (2004).
Selection of genetically modified cell population using
hapten-specific antibody/receptor chimera. Biochem Biophys Res
Commun, 315(1), 132-138. doi:10.1016/j.bbrc.2004.01.030 [0568] 110
Kawahara, M., Shimo, Y., Sogo, T., Hitomi, A., Ueda, H., &
Nagamune, T. (2008). Antigen-mediated migration of murine pro-B
Ba/F3 cells via an antibody/receptor chimera. Journal of
Biotechnology, 133(1), 154-161. doi:10.1016/j.jbiotec.2007.09.009
[0569] 111 Sogo, T., Kawahara, M., Ueda, H., Otsu, M., Onodera, M.,
Nakauchi, H., & Nagamune, T. (2009). T cell growth control
using hapten-specific antibody/interleukin-2 receptor chimera.
Cytokine, 46(1), 127-136. doi:10.1016/j.cyto.2008.12.020 [0570] 112
Kawahara, M., Chen, J., Sogo, T., Teng, J., Otsu, M., Onodera, M.,
et al. (2011). Growth promotion of genetically modified
hematopoietic progenitors using an antibody/c-Mpl chimera.
Cytokine, 55(3), 402-408. doi:10.1016/j.cyto.2011.05.024 [0571] 113
Ueda, H., Kawahara, M., Aburatani, T., Tsumoto, K., Todokoro, K.,
Suzuki, E., et al. (2000). Cell-growth control by monomeric
antigen: the cell surface expression of lysozyme-specific Ig
V-domains fused to truncated Epo receptor. J Immunol Methods,
241(1-2), 159-170. [0572] 114 Kerppola, T. K. (2009). Visualization
of molecular interactions using bimolecular fluorescence
complementation analysis: Characteristics of protein fragment
complementation. Chemical Society Reviews, 38(10), 2876-2886.
[0573] 115 Michnick, S. W., Ear, P. H., Manderson, E. N., Remy, I.,
& Stefan, E. (2007). Universal strategies in research and drug
discovery based on protein-fragment complementation assays. Nature
Reviews Drug Discovery, 6(7), 569-582. doi: 10.1038/nrd231 [0574]
116 Petschnigg, J., Groisman, B., Kotlyar, M., Taipale, M., Zheng,
Y., Kurat, C. F., et al. (2014). The mammalian-membrane two-hybrid
assay (MaMTH) for probing membrane-protein interactions in human
cells. Nat Methods. doi:10.1038/nmeth.2895 [0575] 117 Renaut, L.,
Monnet, C., Dubreuil, O., Zaki, O., Crozet, F., Bouayadi, K., et
al. (2012). Affinity maturation of antibodies. Optimized methods to
generate high-quality scfv libraries and isolate igg candidates by
high-throughput screening. Methods in Molecular Biology (Vol. 907,
pp. 451-461) [0576] 118 Dyson, M. R., Zheng, Y., Zhang, C.,
Colwill, K., Pershad, K., Kay, B. K., et al. (2011). Mapping
protein interactions by combining antibody affinity maturation and
mass spectrometry. Anal Biochem, 417(1), 25-35.
doi:10.1016/j.ab.2011.05.005 [0577] 119 de Felipe P (2002)
Polycistronic viral vectors. Curr Gene Ther 2: 355-378. doi:
10.2174/1566523023347742. [0578] 120 Foote, J., & Winter, G.
(1992). Antibody framework residues affecting the conformation of
the hypervariable loops. J Mol Biol, 224(2), 487-499. [0579] 121
Massie, B., Dionne, J., Lamarche, N., Fleurent, J., &
Langelier, Y. (1995). Improved adenovirus vector provides herpes
simplex virus ribonucleotide reductase R1 and R2 subunits very
efficiently. Nature Biotechnology, 13(6), 602-608. [0580] 122 Kim,
D. W., Uetsuki, T., Kaziro, Y., Yamaguchi, N., & Sugano, S.
(1990). Use of the human elongation factor 1 alpha promoter as a
versatile and efficient expression system. Gene, 91(2), 217-223.
[0581] 123 Holden, P., Keene, D. R., Lunstrum, G. P., Bachinger, H.
P., & Horton, W. A. (2005). Secretion of cartilage oligomeric
matrix protein is affected by the signal peptide. J Biol Chem,
280(17), 17172-17179. [0582] 124 Sadelain, M., Papapetrou, E. P.,
& Bushman, F. D. (2011). Safe harbours for the integration of
new DNA in the human genome. Nature Reviews Cancer, 12(1), 51-58.
[0583] 125 Sanjana, N. E., Cong, L., Zhou, Y., Cunniff, M. M.,
Feng, G., & Zhang, F. (2012). A transcription activator-like
effector toolbox for genome engineering. Nat Protoc, 7(1), 171-192.
doi: 10.1038/nprot.2011.431 [0584] 126 Falk, R., Falk, A., Dyson,
M. R., Melidoni, A. N., Parthiban, K., Young, J. L., et al. (2012).
Generation of anti-Notch antibodies and their application in
blocking Notch signalling in neural stem cells. Methods, 58(1),
69-78. doi: 10.1016/j.ymeth.2012.07.008 [0585] 127 Martin, C. D.,
Rojas, G., Mitchell, J. N., Vincent, K. J., Wu, J., McCafferty, J.,
& Schofield, D. J. (2006). A simple vector system to improve
performance and utilisation of recombinant antibodies. BMC
Biotechnology, 6, 46. [0586] 128 Reyon, D., Tsai, S. Q., Khgayter,
C., Foden, J. A., Sander, J. D., & Joung, J. K. (2012). FLASH
assembly of TALENs for high-throughput genome editing. Nature
Biotechnology, 30(5), 460-465. doi: 10.1038/nbt.2170 [0587] 129 Van
Der Weyden, L., Adams, D. J., Harris, L. W., Tannahill, D., Arends,
M. J., & Bradley, A. (2005). Null and conditional Semaphorin 3B
alleles using a flexible puro.DELTA.tk LoxP/FRT vector. Genesis,
41(4), 171-178 [0588] 130 de Felipe, P., & Ryan, M. D. (2004).
Targeting of proteins derived from self-processing polyproteins
containing multiple signal sequences. Traffic, 5(8), 616-626.
[0589] 131 Raymond, C. S., & Soriano, P. (2007).
High-efficiency FLP and PhiC31 site-specific recombination in
mammalian cells. PLoS ONE, 2(1), e162. doi:
10.1371/journal.pone.0000162 [0590] 132 Kranz, A., Fu, J.,
Duerschke, K., Weidlich, S., Naumann, R., Stewart, A. F., &
Anastassiadis, K. (2010). An improved Flp deleter mouse in C57Bl/6
based on Flp.sub.o recombinase. Genesis, 48(8), 512-520.
doi:10.1002/dvg.20641 [0591] 133 Szymczak A L, Vignali D A (2005)
Development of 2 A peptide-based strategies in the design of
multicistronic vectors. Expert Opin Biol Ther 5: 627-638. doi:
10.1517/14712598.5.5.627 [0592] 134 Chapple, S. D., Crofts, A. M.,
Shadbolt, S. P., McCafferty, J., and Dyson, M. R. (2006).
Multiplexed expression and screening for recombinant protein
production in mammalian cells. BMC biotechnology 6, 49. [0593] 135.
Zhao, Y. et al. Multiple injections of electroporated autologous T
cells expressing a chimeric antigen receptor mediate regression of
human disseminated tumor. Cancer Research 70, 9053-9061 (2010).
[0594] 136 Szymczak, A. L. et al. Correction of multi-gene
deficiency in vivo using a single `self-cleaving` 2A peptide-based
retroviral vector. Nature biotechnology 22, 589-594 (2004). [0595]
137. Li, Y. et al. Directed evolution of human T-cell receptors
with picomolar affinities by phage display. Nat. Biotechnol. 23,
349-354 (2005). [0596] 138. Zhao, Y. et al. High-affinity TCRs
generated by phage display provide CD4+ T cells with the ability to
recognize and kill tumor cell lines. J Immunol. 179, 5845-5854
(2007). [0597] 139. Madura, F. et al. T-cell receptor specificity
maintained by altered thermodynamics. The Journal of biological
chemistry 288, 18766-18775 (2013). [0598] 140. Pierce, B. G. et al.
Computational design of the affinity and specificity of a
therapeutic T cell receptor. PLoS Comput Biol 10, e1003478 (2014).
[0599] 141. Sebestyen, Z. et al. Human TCR that incorporate CD3zeta
induce highly preferred pairing between TCRalpha and beta chains
following gene transfer. J. Immunol. 180, 7736-7746 (2008). [0600]
142. Roszik, J. et al. T-cell synapse formation depends on antigen
recognition but not CD3 interaction: studies with TCR:.xi., a
candidate transgene for TCR gene therapy. Eur. J. Immunol. 41,
1288-1297 (2011). [0601] 143. Cohen, C. J., Zhao, Y., Zheng, Z.,
Rosenberg, S. A. & Morgan, R. A. Enhanced antitumor activity of
murine-human hybrid T-cell receptor (TCR) in human lymphocytes is
associated with improved pairing and TCR/CD3 stability. Cancer
Research 66, 8878-8886 (2006). [0602] 144. Huovinen, T. et al.
Primer extension mutagenesis powered by selective rolling circle
amplification. PLoS ONE 7, e31817 (2012). [0603] 145. Cribbs, A.
P., Kennedy, A., Gregory, B. & Brennan, F. M. Simplified
production and concentration of lentiviral vectors to achieve high
transduction in primary human T cells. BMC biotechnology 13, 98
(2013). [0604] 146. Oelke, M. et al. Ex vivo induction and
expansion of antigen-specific cytotoxic T cells by HLA-Ig-coated
artificial antigen-presenting cells. Nat Med 9, 619-624 (2003).
[0605] 147. Wolff, M. & Greenberg, P. D. Antigen-specific
activation and cytokine-facilitated expansion of na
ive, human CD8+ T cells. Nature Protocols 9, 950-966 (2014). [0606]
148. Lipowska-Bhalla, G., Gilham, D. E., Hawkins, R. E. &
Rothwell, D. G. Isolation of tumor antigen-specific single-chain
variable fragments using a chimeric antigen receptor bicistronic
retroviral vector in a Mammalian screening protocol. Hum Gene Ther
Methods 24, 381-391 (2013). [0607] 149. Kelly, R. J., Sharon, E.,
Pastan, I. & Hassan, R. Mesothelin-targeted agents in clinical
trials and in preclinical development. Mol. Cancer Ther. 11,
517-525 (2012). [0608] 150. Atanackovic, D. et al. Surface molecule
CD229 as a novel target for the diagnosis and treatment of multiple
myeloma. Haematologica 96, 1512-1520 (2011). [0609] 151. Bund, D.,
Mayr, C., Kofler, D. M., Hallek, M. & Wendtner, C.-M. Human Ly9
(CD229) as novel tumor-associated antigen (TAA) in chronic
lymphocytic leukemia (B-CLL) recognized by autologous CD8+ T cells.
Exp. Hematol. 34, 860-869 (2006). [0610] 152. Tiede, C. et al.
Adhiron: a stable and versatile peptide display scaffold for
molecular recognition applications. Protein Eng. Des. Sel. 27,
145-155 (2014). [0611] 153. Maresca, M., Lin, V. G., Guo, N. &
Yang, Y. Obligate ligation-gated recombination (ObLiGaRe):
custom-designed nuclease-mediated targeted integration through
nonhomologous end joining. Genome Res. 23, 539-546 (2013). [0612]
154. McVey, M. & Lee, S. E. MMEJ repair of double-strand breaks
(director's cut): deleted sequences and alternative endings. Trends
Genet. 24, 529-538 (2008). [0613] 155. Nakade, S. et al.
Microhomology-mediated end-joining-dependent integration of donor
DNA in cells and animals using TALENs and CRISPR/Cas9. Nat Commun
5, 5560 (2014). [0614] 156. Chiche, L. et al. Squash inhibitors:
From structural motifs to macrocyclic knottins. Current Protein
& Peptide Science 5, 341-349.
Sequence CWU 1
1
14215396DNAArtificial SequencepD1 dual promoter antibody expression
cassette for surface expression 1ggtaccgaat tccgtgaggc tccggtgccc
gtcagtgggc agagcgcaca tcgcccacag 60tccccgagaa gttgggggga ggggtcggca
attgaaccgg tgcctagaga aggtggcgcg 120gggtaaactg ggaaagtgat
gtcgtgtact ggctccgcct ttttcccgag ggtgggggag 180aaccgtatat
aagtgcagta gtcgccgtga acgttctttt tcgcaacggg tttgccgcca
240gaacacaggt aagtgccgtg tgtggttccc gcgggcctgg cctctttacg
ggttatggcc 300cttgcgtgcc ttgaattact tccacctggc tccagtacgt
gattcttgat cccgagctgg 360agccaggggc gggccttgcg ctttaggagc
cccttcgcct cgtgcttgag ttgaggcctg 420gcctgggcgc tggggccgcc
gcgtgcgaat ctggtggcac cttcgcgcct gtctcgctgc 480tttcgataag
tctctagcca tttaaaattt ttgatgacct gctgcgacgc tttttttctg
540gcaagatagt cttgtaaatg cgggccagga tctgcacact ggtatttcgg
tttttgggcc 600cgcggccggc gacggggccc gtgcgtccca gcgcacatgt
tcggcgaggc ggggcctgcg 660agcgcggcca ccgagaatcg gacgggggta
gtctcaagct ggccggcctg ctctggtgcc 720tggcctcgcg ccgccgtgta
tcgccccgcc ctgggcggca aggctggccc ggtcggcacc 780agttgcgtga
gcggaaagat ggccgcttcc cggccctgct ccagggggct caaaatggag
840gacgcggcgc tcgggagagc gggcgggtga gtcacccaca caaaggaaaa
gggcctttcc 900gtcctcagcc gtcgcttcat gtgactccac ggagtaccgg
gcgccgtcca ggcacctcga 960ttagttctgg agcttttgga gtacgtcgtc
tttaggttgg ggggaggggt tttatgcgat 1020ggagtttccc cacactgagt
gggtggagac tgaagttagg ccagcttggc acttgatgta 1080attctcgttg
gaatttgccc tttttgagtt tggatcttgg ttcattctca agcctcagac
1140agtggttcaa agtttttttc ttccatttca ggtgtcgtga gacgtggcca
ccatgagggc 1200ctggatcttc tttctccttt gcctggccgg gagggctctg
gcagctagcg acatccagat 1260gacccagagc ccaagcagcc tgagcgccag
cgtgggtgac agagtgacca tcacctgtag 1320agccagcggt aacatccaca
actacctggc ttggtaccag cagaagccag gtaaggctcc 1380aaagctgctg
atctactaca ccaccaccct ggctgacggt gtgccaagca gattcagcgg
1440tagcggtagc ggtaccgact acaccttcac catcagcagc ctccagccag
aggacatcgc 1500cacctactac tgccagcact tctggagcac cccaaggacg
ttcggccaag ggaccaaggt 1560ggaaatcaaa cgtaccgcgg ccgccccttc
cgtgttcatc ttccctccct ccgacgagca 1620gctgaagtcc ggcaccgcct
ctgtggtgtg cctgctgaac aacttctacc ctcgggaggc 1680caaggtgcag
tggaaggtgg acaacgccct gcagtccggc aactcccagg aatccgtcac
1740cgagcaggac tccaaggact ctacctactc cctgtcctcc accctgaccc
tgtccaaggc 1800cgactacgag aagcacaagc tgtacgcctg cgaagtgacc
caccagggcc tgtcctctcc 1860cgtgaccaag tccttcaacc ggggcgagtg
ctaataaaag cttacgacgt gatcagcctc 1920gactgtgcct tctagttgcc
agccatctgt tgtttgcccc tcccccgtgc cttccttgac 1980cctggaaggt
gccactccca ctgtcctttc ctaataaaat gaggaaattg catcgcattg
2040tctgagtagg tgtcattcta ttctgggggg tggggtgggg caggacagca
agggggagga 2100ttgggaagac aatagcaggc atgctgggga acattgatta
ttgactagtt attaatagta 2160atcaattacg gggtcattag ttcatagccc
atatatggag ttccgcgtta cataacttac 2220ggtaaatggc ccgcctggct
gaccgcccaa cgacccccgc ccattgacgt caataatgac 2280gtatgttccc
atagtaacgc caatagggac tttccattga cgtcaatggg tggagtattt
2340acggtaaact gcccacttgg cagtacatca agtgtatcat atgccaagtc
cgccccctat 2400tgacgtcaat gacggtaaat ggcccgcctg gcattatgcc
cagtacatga ccttacggga 2460ctttcctact tggcagtaca tctacgtatt
agtcatcgct attaccatag tgatgcggtt 2520ttggcagtac accaatgggc
gtggatagcg gtttgactca cggggatttc caagtctcca 2580ccccattgac
gtcaatggga gtttgttttg gcaccaaaat caacgggact ttccaaaatg
2640tcgtaataac cccgccccgt tgacgcaaat gggcggtagg cgtgtacggt
gggaggtcta 2700tataagcaga gctcgtttag tgaaccgtca gatcctcact
ctcttccgca tcgctgtctg 2760cgagggccag ctgttgggct cgcggttgag
gacaaactct tcgcggtctt tccagtactc 2820ttggatcgga aacccgtcgg
cctccgaacg gtactccgcc accgagggac ctgagcgagt 2880ccgcatcgac
cggatcggaa aacctctcgt gaaaggcgtc taaccagtca cagtcgcaag
2940gtaggctgag caccgtggcg ggcggcagcg ggtggcggtc ggggttgttt
ctggcggagg 3000tgctgctgat gatgtaatta aagtaggcgg tcttgagacg
gcggatggtc gaggtgaggt 3060gtggcaggct tgagatccag ctgttggggt
gagtactccc tctcaaaagc gggcattact 3120tctgcgctaa gattgtcagt
ttccaaaaac gaggaggatt tgatattcac ctggcccgat 3180ctggccatac
acttgagtga caatgacatc cactttgcct ttctctccac aggtgtccac
3240tcccaggtcc aagtttgtgg aaattaatac gacgtggcca ccatgagttg
gagctgtatc 3300atcctcttct tggtagcaac agctacaggt aaggggttaa
cagtagcagg cttgaggtct 3360ggacatatat atgggtgaca atgacatcca
ctttgccttt ctctccacag gcgccatggc 3420ccaggtccaa ctgcaggaga
gcggtccagg tcttgtgaga cctagccaga ccctgagcct 3480gacctgcacc
gtgtctggca gcaccttcag cggctatggt gtaaactggg tgagacagcc
3540acctggacga ggtcttgagt ggattggaat gatttggggt gatggaaaca
cagactataa 3600ttcagctctc aaatccagag tgacaatgct ggtagacacc
agcaagaacc agttcagcct 3660gagactcagc agcgtgacag ccgccgacac
cgcggtctat tattgtgcaa gagagagaga 3720ttataggctt gactactggg
gtcaaggcag cctcgtcaca gtctcgagtg ccagcaccaa 3780gggccccagc
gtgttccctc tggccccctg tagcagaagc accagcgaga gcacagccgc
3840cctgggctgc ctggtcaagg actacttccc cgagcccgtg accgtgtcct
ggaactctgg 3900cgctctgacc agcggcgtgc acacctttcc agccgtgctg
cagagcagcg gcctgtacag 3960cctgagcagc gtggtcaccg tgcccagcag
caacttcggc acccagacct acacctgtaa 4020cgtggaccac aagcccagca
acaccaaggt ggacaagacc gtggaacgga agtgctgcgt 4080ggaatgcccc
ccctgtcccg ctcctccagt ggctggacct tccgtgttcc tgttcccccc
4140aaagcccaag gacaccctga tgatcagccg gacccccgaa gtgacctgcg
tggtggtgga 4200cgtgtcccac gaggaccccg aggtgcagtt caattggtac
gtggacggcg tggaagtgca 4260caacgccaag accaagccca gagaggaaca
gttcaacagc accttccggg tggtgtccgt 4320gctgaccgtg gtgcaccagg
actggctgaa cggcaaagag tacaagtgcg ccgtctccaa 4380caagggcctg
cctgccccca tcgagaaaac catcagcaag accaagggcc agcctcgcga
4440gcctcaggtg tacacactgc cccccagccg ggaagagatg accaagaacc
aggtgtccct 4500gacctgcctc gtgaagggct tctaccccag cgatatcgcc
gtggaatggg agagcaacgg 4560ccagcccgag aacaactaca agaccacccc
ccccatgctg gacagcgacg gctcattctt 4620cctgtacagc aagctgacag
tggacaagag ccggtggcag cagggcaacg tgttcagctg 4680cagcgtgatg
cacgaggccc tgcacaacca ctacacccag aagtccctga gcctgagccc
4740cggcaaggga tccaaggtaa gtttaaacat atatataact ttaaataatt
ggcattattt 4800aaagttacta ctaactaacc ctgattattt aaattttcag
gaacaaaaac tcatctcaga 4860agaggatctg aatgctgtgg gccaggacac
gcaggaggtc atcgtggtgc cacactcctt 4920gccctttaag gtggtggtga
tctcagccat cctggccctg gtggtgctca ccatcatctc 4980ccttatcatc
ctcatcatgc tttggcagaa gaagccacgt tagtaacagg taagagtgta
5040actttaaata atgccaatta tttaaagtta ctgactctct ctgcttacga
cgcttcttct 5100tttttttttc ctgcaggggt agtaatcagc ctcgactgtg
ccttctagtt gccagccatc 5160tgttgtttgc ccctcccccg tgccttcctt
gaccctggaa ggtgccactc ccactgtcct 5220ttcctaataa aatgaggaaa
ttgcatcgca ttgtctgagt aggtgtcatt ctattctggg 5280gggtggggtg
gggcaggaca gcaaggggga ggattgggaa gacaatagca ggcatgctgg
5340ggatggcccg ggcatgataa cttcgtataa tgtatgctat acgaagttat gtatac
53962233PRTArtificial sequenceBM40 Leader, Humanised D1.3 VL and
Human C Kappa sequence 2Met Arg Ala Trp Ile Phe Phe Leu Leu Cys Leu
Ala Gly Arg Ala Leu1 5 10 15Ala Ala Ser Asp Ile Gln Met Thr Gln Ser
Pro Ser Ser Leu Ser Ala 20 25 30Ser Val Gly Asp Arg Val Thr Ile Thr
Cys Arg Ala Ser Gly Asn Ile 35 40 45His Asn Tyr Leu Ala Trp Tyr Gln
Gln Lys Pro Gly Lys Ala Pro Lys 50 55 60Leu Leu Ile Tyr Tyr Thr Thr
Thr Leu Ala Asp Gly Val Pro Ser Arg65 70 75 80Phe Ser Gly Ser Gly
Ser Gly Thr Asp Tyr Thr Phe Thr Ile Ser Ser 85 90 95Leu Gln Pro Glu
Asp Ile Ala Thr Tyr Tyr Cys Gln His Phe Trp Ser 100 105 110Thr Pro
Arg Thr Phe Gly Gln Gly Thr Lys Val Glu Ile Lys Arg Thr 115 120
125Ala Ala Ala Pro Ser Val Phe Ile Phe Pro Pro Ser Asp Glu Gln Leu
130 135 140Lys Ser Gly Thr Ala Ser Val Val Cys Leu Leu Asn Asn Phe
Tyr Pro145 150 155 160Arg Glu Ala Lys Val Gln Trp Lys Val Asp Asn
Ala Leu Gln Ser Gly 165 170 175Asn Ser Gln Glu Ser Val Thr Glu Gln
Asp Ser Lys Asp Ser Thr Tyr 180 185 190Ser Leu Ser Ser Thr Leu Thr
Leu Ser Lys Ala Asp Tyr Glu Lys His 195 200 205Lys Leu Tyr Ala Cys
Glu Val Thr His Gln Gly Leu Ser Ser Pro Val 210 215 220Thr Lys Ser
Phe Asn Arg Gly Glu Cys225 230315PRTArtificial sequenceLeader 1
sequence 3Met Ser Trp Ser Cys Ile Ile Leu Phe Leu Val Ala Thr Ala
Thr1 5 10 154449PRTArtificial sequenceLeader 2 and D1.3 VH
sequence, CH1 domain, Hinge, CH2 and CH3 domains 4Gly Ala Met Ala
Gln Val Gln Leu Gln Glu Ser Gly Pro Gly Leu Val1 5 10 15Arg Pro Ser
Gln Thr Leu Ser Leu Thr Cys Thr Val Ser Gly Ser Thr 20 25 30Phe Ser
Gly Tyr Gly Val Asn Trp Val Arg Gln Pro Pro Gly Arg Gly 35 40 45Leu
Glu Trp Ile Gly Met Ile Trp Gly Asp Gly Asn Thr Asp Tyr Asn 50 55
60Ser Ala Leu Lys Ser Arg Val Thr Met Leu Val Asp Thr Ser Lys Asn65
70 75 80Gln Phe Ser Leu Arg Leu Ser Ser Val Thr Ala Ala Asp Thr Ala
Val 85 90 95Tyr Tyr Cys Ala Arg Glu Arg Asp Tyr Arg Leu Asp Tyr Trp
Gly Gln 100 105 110Gly Ser Leu Val Thr Val Ser Ser Ala Ser Thr Lys
Gly Pro Ser Val 115 120 125Phe Pro Leu Ala Pro Cys Ser Arg Ser Thr
Ser Glu Ser Thr Ala Ala 130 135 140Leu Gly Cys Leu Val Lys Asp Tyr
Phe Pro Glu Pro Val Thr Val Ser145 150 155 160Trp Asn Ser Gly Ala
Leu Thr Ser Gly Val His Thr Phe Pro Ala Val 165 170 175Leu Gln Ser
Ser Gly Leu Tyr Ser Leu Ser Ser Val Val Thr Val Pro 180 185 190Ser
Ser Asn Phe Gly Thr Gln Thr Tyr Thr Cys Asn Val Asp His Lys 195 200
205Pro Ser Asn Thr Lys Val Asp Lys Thr Val Glu Arg Lys Cys Cys Val
210 215 220Glu Cys Pro Pro Cys Pro Ala Pro Pro Val Ala Gly Pro Ser
Val Phe225 230 235 240Leu Phe Pro Pro Lys Pro Lys Asp Thr Leu Met
Ile Ser Arg Thr Pro 245 250 255Glu Val Thr Cys Val Val Val Asp Val
Ser His Glu Asp Pro Glu Val 260 265 270Gln Phe Asn Trp Tyr Val Asp
Gly Val Glu Val His Asn Ala Lys Thr 275 280 285Lys Pro Arg Glu Glu
Gln Phe Asn Ser Thr Phe Arg Val Val Ser Val 290 295 300Leu Thr Val
Val His Gln Asp Trp Leu Asn Gly Lys Glu Tyr Lys Cys305 310 315
320Ala Val Ser Asn Lys Gly Leu Pro Ala Pro Ile Glu Lys Thr Ile Ser
325 330 335Lys Thr Lys Gly Gln Pro Arg Glu Pro Gln Val Tyr Thr Leu
Pro Pro 340 345 350Ser Arg Glu Glu Met Thr Lys Asn Gln Val Ser Leu
Thr Cys Leu Val 355 360 365Lys Gly Phe Tyr Pro Ser Asp Ile Ala Val
Glu Trp Glu Ser Asn Gly 370 375 380Gln Pro Glu Asn Asn Tyr Lys Thr
Thr Pro Pro Met Leu Asp Ser Asp385 390 395 400Gly Ser Phe Phe Leu
Tyr Ser Lys Leu Thr Val Asp Lys Ser Arg Trp 405 410 415Gln Gln Gly
Asn Val Phe Ser Cys Ser Val Met His Glu Ala Leu His 420 425 430Asn
His Tyr Thr Gln Lys Ser Leu Ser Leu Ser Pro Gly Lys Gly Ser 435 440
445Lys560PRTArtificialMyc epitope, PDGFR spacer and PDGFR
Transmembrane region (TM) 5Glu Gln Lys Leu Ile Ser Glu Glu Asp Leu
Asn Ala Val Gly Gln Asp1 5 10 15Thr Gln Glu Val Ile Val Val Pro His
Ser Leu Pro Phe Lys Val Val 20 25 30Val Ile Ser Ala Ile Leu Ala Leu
Val Val Leu Thr Ile Ile Ser Leu 35 40 45Ile Ile Leu Ile Met Leu Trp
Gln Lys Lys Pro Arg 50 55 6061542DNAArtificial SequencepD1-huD1.3
donor construct fragment containing AAVS1 left homology arm, splice
acceptor site, Blasticidin gene, SV40 polyA 6ggtaccgaat tcgccctttg
ctttctctga cctgcattct ctcccctggg cctgtgccgc 60tttctgtctg cagcttgtgg
cctgggtcac ctctacggct ggcccagatc cttccctgcc 120gcctccttca
ggttccgtct tcctccactc cctcttcccc ttgctctctg ctgtgttgct
180gcccaaggat gctctttccg gagcacttcc ttctcggcgc tgcaccacgt
gatgtcctct 240gagcggatcc tccccgtgtc tgggtcctct ccgggcatct
ctcctccctc acccaacccc 300atgccgtctt cactcgctgg gttccctttt
ccttctcctt ctggggcctg tgccatctct 360cgtttcttag gatggccttc
tccgacggat gtctcccttg cgtcccgcct ccccttcttg 420taggcctgca
tcatcaccgt ttttctggac aaccccaaag taccccgtct ccctggcttt
480agccacctct ccatcctctt gctttctttg cctggacacc ccgttctcct
gtggattcgg 540gtcacctctc actcctttca tttgggcagc tcccctaccc
cccttacctc tctagtctgt 600gcaagctctt ccagccccct gtcatggcat
cttccagggg tccgagagct cagctagtct 660tcttcctcca acccgggccc
ctatgtccac ttcaggacag catgtttgct gcctccaggg 720atcctgtgtc
cccgagctgg gaccacctta tattcccagg gccggttaat gtggctctgg
780ttctgggtac ttttatctgt cccctccacc ccacagtggg gcaagatgca
tcttctgacc 840tcttctcttc ctcccacagg gcatggcaaa acctctgagc
caggaagaaa gcacactgat 900tgaaagagca accgctacta tcaacagcat
ccccatctcc gaagactatt ctgtggctag 960tgccgctctg tccagcgacg
ggagaatctt caccggtgtg aacgtctacc actttacagg 1020cggaccatgc
gcagagctgg tggtcctggg gactgcagcc gctgcagccg ctggtaatct
1080gacctgtatc gtggccattg gcaacgaaaa taggggcatc ctgtccccat
gcggcaggtg 1140tcggcaggtg ctgctggatc tgcatcctgg catcaaggca
attgtcaaag actctgatgg 1200acagcctacc gccgtcggta tccgtgaact
gctgcctagc ggctatgtct gggagggata 1260atgagcttgg cttcgaaatg
accgaccaag cgacgcccaa cctgccatca cgagatttcg 1320attccaccgc
cgccttctat gaaaggttgg gcttcggaat cgttttccgg gacgccggct
1380ggatgatcct ccagcgcggg gatctcatgc tggagttctt cgcccacccc
aacttgttta 1440ttgcagctta taatggttac aaataaagca atagcatcac
aaatttcaca aataaagcat 1500ttttttcact gcattctagt tgtggtttaa
ttaagtcaat tc 15427924DNAArtificial SequencepD1-huD1.3 fragment
containing AAVS1 right homology arm 7tggcccgggc atgataactt
cgtataatgt atgctatacg aagttatgta tacggcgcgc 60ccactaggga caggattggt
gacagaaaag ccccatcctt aggcctcctc cttcctagtc 120tcctgatatt
gggtctaacc cccacctcct gttaggcaga ttccttatct ggtgacacac
180ccccatttcc tggagccatc tctctccttg ccagaacctc taaggtttgc
ttacgatgga 240gccagagagg atcctgggag ggagagcttg gcagggggtg
ggagggaagg gggggatgcg 300tgacctgccc ggttctcagt ggccaccctg
cgctaccctc tcccagaacc tgagctgctc 360tgacgcggct gtctggtgcg
tttcactgat cctggtgctg cagcttcctt acacttccca 420agaggagaag
cagtttggaa aaacaaaatc agaataagtt ggtcctgagt tctaactttg
480gctcttcacc tttctagtcc ccaatttata ttgttcctcc gtgcgtcagt
tttacctgtg 540agataaggcc agtagccagc cccgtcctgg cagggctgtg
gtgaggaggg gggtgtccgt 600gtggaaaact ccctttgtga gaatggtgcg
tcctaggtgt tcaccaggtc gtggccgcct 660ctactccctt tctctttctc
catccttctt tccttaaaga gtccccagtg ctatctggga 720catattcctc
cgcccagagc agggtcccgc ttccctaagg ccctgctctg ggcttctggg
780tttgagtcct tggcaagccc aggagaggcg ctcaggcttc cctgtccccc
ttcctcgtcc 840accatctcat gcccctggct ctcctgcccc ttccctacag
gggttcctgg ctctgctctg 900acgcgtgtat actcgatctt tccg
9248132PRTArtificial SequenceSynthetic Blasticidin resistance gene
8Met Ala Lys Pro Leu Ser Gln Glu Glu Ser Thr Leu Ile Glu Arg Ala1 5
10 15Thr Ala Thr Ile Asn Ser Ile Pro Ile Ser Glu Asp Tyr Ser Val
Ala 20 25 30Ser Ala Ala Leu Ser Ser Asp Gly Arg Ile Phe Thr Gly Val
Asn Val 35 40 45Tyr His Phe Thr Gly Gly Pro Cys Ala Glu Leu Val Val
Leu Gly Thr 50 55 60Ala Ala Ala Ala Ala Ala Gly Asn Leu Thr Cys Ile
Val Ala Ile Gly65 70 75 80Asn Glu Asn Arg Gly Ile Leu Ser Pro Cys
Gly Arg Cys Arg Gln Val 85 90 95Leu Leu Asp Leu His Pro Gly Ile Lys
Ala Ile Val Lys Asp Ser Asp 100 105 110Gly Gln Pro Thr Ala Val Gly
Ile Arg Glu Leu Leu Pro Ser Gly Tyr 115 120 125Val Trp Glu Gly
1309734DNAArtificial SequencepD6 vector for scFv-Fc expression,
fragment from Nco1 site to Pme1 site containing a fragment (last 4
amino acids) of the leader sequence, the complete CH2 domain and
CH3 domain 9ggcgccatgg cccaggtcgc ggccgccgtg gaatgccccc cctgtcccgc
tcctccagtg 60gctggacctt ccgtgttcct gttcccccca aagcccaagg acaccctgat
gatcagccgg 120acccccgaag tgacctgcgt ggtggtggac gtgtcccacg
aggaccccga ggtgcagttc 180aattggtacg tggacggcgt ggaagtgcac
aacgccaaga ccaagcccag agaggaacag 240ttcaacagca ccttccgggt
ggtgtccgtg ctgaccgtgg tgcaccagga ctggctgaac 300ggcaaagagt
acaagtgcgc cgtctccaac aagggcctgc ctgcccccat cgagaaaacc
360atcagcaaga ccaagggcca gcctcgcgag cctcaggtgt acacactgcc
ccccagccgg 420gaagagatga ccaagaacca ggtgtccctg acctgcctcg
tgaagggctt ctaccccagc 480gatatcgccg tggaatggga gagcaacggc
cagcccgaga acaactacaa gaccaccccc 540cccatgctgg acagcgacgg
ctcattcttc ctgtacagca agctgacagt ggacaagagc 600cggtggcagc
agggcaacgt gttcagctgc agcgtgatgc acgaggccct gcacaaccac
660tacacccaga agtccctgag cctgagcccc ggcaagggat ccaaggtaag
tttaaacata 720tatataactt taaa 734104PRTArtificial SequenceVector
pD6, fragment at the end of leader sequence 10Gly Ala Met
Ala111219PRTArtificial SequenceVector pD6 CH2 and CH3 domains 11Ala
Pro
Pro Val Ala Gly Pro Ser Val Phe Leu Phe Pro Pro Lys Pro1 5 10 15Lys
Asp Thr Leu Met Ile Ser Arg Thr Pro Glu Val Thr Cys Val Val 20 25
30Val Asp Val Ser His Glu Asp Pro Glu Val Gln Phe Asn Trp Tyr Val
35 40 45Asp Gly Val Glu Val His Asn Ala Lys Thr Lys Pro Arg Glu Glu
Gln 50 55 60Phe Asn Ser Thr Phe Arg Val Val Ser Val Leu Thr Val Val
His Gln65 70 75 80Asp Trp Leu Asn Gly Lys Glu Tyr Lys Cys Ala Val
Ser Asn Lys Gly 85 90 95Leu Pro Ala Pro Ile Glu Lys Thr Ile Ser Lys
Thr Lys Gly Gln Pro 100 105 110Arg Glu Pro Gln Val Tyr Thr Leu Pro
Pro Ser Arg Glu Glu Met Thr 115 120 125Lys Asn Gln Val Ser Leu Thr
Cys Leu Val Lys Gly Phe Tyr Pro Ser 130 135 140Asp Ile Ala Val Glu
Trp Glu Ser Asn Gly Gln Pro Glu Asn Asn Tyr145 150 155 160Lys Thr
Thr Pro Pro Met Leu Asp Ser Asp Gly Ser Phe Phe Leu Tyr 165 170
175Ser Lys Leu Thr Val Asp Lys Ser Arg Trp Gln Gln Gly Asn Val Phe
180 185 190Ser Cys Ser Val Met His Glu Ala Leu His Asn His Tyr Thr
Gln Lys 195 200 205Ser Leu Ser Leu Ser Pro Gly Lys Gly Ser Lys 210
2151260DNAArtificial SequenceFragment of sequence of pD4 vector
preceding and including the start of the AAVS1 left homology arm
12ggtaccgaat tcgccctttg ctttctctga cctgcattct ctcccctggg cctgtgccgc
60133933DNAArtificial sequenceFragment of sequence of vector pD4
including the end of the AAVS1 LHA, FRT site, Lox2272 site, 1-Sce1
meganuclease site, GFP left TALEN binding site, GFP right TALEN
binding site, T2A, GFP, PGK promoter, puromycin delta TK, loxP site
and start of AAVS1 RHA 13ttctgggtac ttttatctgt cccctccacc
ccacagtggg gcaagatgca tgaagttcct 60attccgaagt tcctattctc tagaaagtat
aggaacttcg accataactt cgtataaagt 120atcctatacg aagttatgcg
atcgctcgcg cgtagggata acagggtaat aagtccaccg 180gtcgccacca
tggtgagcaa gggcgaggag ctgttcactt ctgacctctt ctcttcctcc
240cacagggcct agagagatct ggcagcggag agggcagagg aagtcttcta
acatgcggtg 300acgtggagga gaatcccgga ccgatggtga gcaagggaga
agaactcttc accggggtgg 360tgcccatcct ggtcgagctg gacggcgacg
tgaacggcca caagttcagc gtgtccggcg 420agggcgaggg cgatgccacc
tacggcaagc tgaccctgaa gttcatctgc accaccggca 480agctgcccgt
gccctggccc accctcgtga ccaccctgac ctacggcgtg cagtgcttca
540gccgctaccc cgaccacatg aagcagcacg acttcttcaa gtccgccatg
cccgaaggct 600acgtccagga gcgcaccatc ttcttcaagg acgacggcaa
ctacaagacc cgcgccgagg 660tgaagttcga gggcgacacc ctggtgaacc
gcatcgagct gaagggcatc gacttcaagg 720aggacggcaa catcctgggg
cacaagctgg agtacaacta caacagccac aacgtctata 780tcatggccga
caagcagaag aacggcatca aggtgaactt caagatccgc cacaacatcg
840aggacggcag cgtgcagctc gccgaccact accagcagaa cacccccatc
ggcgacggcc 900ccgtgctgct gcccgacaac cactacctga gcacccagtc
cgccctgagc aaagacccca 960acgagaagcg cgatcacatg gtcctgctgg
agttcgtgac cgccgccggg atcactcacg 1020gcatggacga gctgtacaag
aagctgagcc acggcttccc gccggcggtg gcggcgcagg 1080atgatggcac
gctgcccatg tcttgtgccc aggagagcgg gatggaccgt caccctgcag
1140cctgtgcttc tgctaggatc aatgtgtagg tgatcagcct cgactgtgcc
ttctagttgc 1200cagccatctg ttgtttgccc ctcccccgtg ccttccttga
ccctggaagg tgccactccc 1260actgtccttt cctaataaaa tgaggaaatt
gcatcgcatt gtctgagtag gtgtcattct 1320attctggggg gtggggtggg
gcaggacagc aagggggagg attgggaaga caatagcagg 1380catgctgggg
atggcccaat tctaccgggt aggggaggcg cttttcccaa ggcagtctgg
1440agcatgcgct ttagcagccc cgctgggcac ttggcgctac acaagtggcc
tctggcctcg 1500cacacattcc acatccaccg gtaggcgcca accggctccg
ttctttggtg gccccttcgc 1560gccaccttct actcctcccc tagtcaggaa
gttccccccc gccccgcagc tcgcgtcgtg 1620caggacgtga caaatggaag
tagcacgtct cactagtctc gtgcagatgg acagcaccgc 1680tgagcaatgg
aagcgggtag gcctttgggg cagcggccaa tagcagcttt gctccttcgc
1740tttctgggct cagaggctgg gaaggggtgg gtccgggggc gggctcaggg
gcgggctcag 1800gggcggggcg ggcgcccgaa ggtcctccgg aggcccggca
ttctgcacgc ttcaaaagcg 1860cacgtctgcc gcgctgttct cctcttcctc
atctccgggc ctttcgacct gcagccaacg 1920ccaccatggg gaccgagtac
aagcccacgg tgcgcctcgc cacccgcgac gacgtccccc 1980gggccgtacg
caccctcgcc gccgcgttcg ccgactaccc cgccacgcgc cacaccgtcg
2040acccggaccg ccacatcgag cgggtcaccg agctgcaaga actcttcctc
acgcgcgtcg 2100ggctcgacat cggcaaggtg tgggtcgcgg acgacggcgc
cgcggtggcg gtctggacca 2160cgccggagag cgtcgaagcg ggggcggtgt
tcgccgagat cggcccgcgc atggccgagt 2220tgagcggttc ccggctggcc
gcgcagcaac agatggaagg cctcctggcg ccgcaccggc 2280ccaaggagcc
cgcgtggttc ctggccaccg tcggcgtctc gcccgaccac cagggcaagg
2340gtctgggcag cgccgtcgtg ctccccggag tggaggcggc cgagcgcgcc
ggggtgcccg 2400ccttcctgga gacctccgcg ccccgcaacc tccccttcta
cgagcggctc ggcttcaccg 2460tcaccgccga cgtcgaggtg cccgaaggac
cgcgcacctg gtgcatgacc cgcaagcccg 2520gtgccggatc catgcccacg
ctactgcggg tttatataga cggtcctcac gggatgggga 2580aaaccaccac
cacgcaactg ctggtggccc tgggttcgcg cgacgatatc gtctacgtac
2640ccgagccgat gacttactgg caggtgctgg gggcttccga gacaatcgcg
aacatctaca 2700ccacacaaca ccgcctcgac cagggtgaga tatcggccgg
ggacgcggcg gtggtaatga 2760caagcgccca gataacaatg ggcatgcctt
atgccgtgac cgacgccgtt ctggctcctc 2820atatcggggg ggaggctggg
agctcacatg ccccgccccc ggccctcacc ctcatcttcg 2880accgccatcc
catcgccgcc ctcctgtgct acccggccgc gcgatacctt atgggcagca
2940tgacccccca ggccgtgctg gcgttcgtgg ccctcatccc gccgaccttg
cccggcacaa 3000acatcgtgtt gggggccctt ccggaggaca gacacatcga
ccgcctggcc aaacgccagc 3060gccccggcga gcggcttgac ctggctatgc
tggccgcgat tcgccgcgtt tacgggctgc 3120ttgccaatac ggtgcggtat
ctgcagggcg gcgggtcgtg gcgggaggat tggggacagc 3180tttcggggac
ggccgtgccg ccccagggtg ccgagcccca gagcaacgcg ggcccacgac
3240cccatatcgg ggacacgtta tttaccctgt ttcgggcccc cgagttgctg
gcccccaacg 3300gcgacctgta caacgtgttt gcctgggcct tggacgtctt
ggccaaacgc ctccgtccca 3360tgcacgtctt tatcctggat tacgaccaat
cgcccgccgg ctgccgggac gccctgctgc 3420aacttacctc cgggatggtc
cagacccacg tcaccacccc cggctccata ccgacgatct 3480gcgacctggc
gcgcacgttt gcccgggaga tgggggaggc taactgagct ctagagctcg
3540ctgatcagcc tcgactgtgc cttctagttg ccagccatct gttgtttgcc
cctcccccgt 3600gccttccttg accctggaag gtgccactcc cactgtcctt
tcctaataaa atgaggaaat 3660tgcatcgcat tgtctgagta ggtgtcattc
tattctgggg ggtggggtgg ggcaggacag 3720caagggggag gattgggaag
acaatagcag gcatgctggg gatgcggtgg gctctatggc 3780ttctgaggcg
gaaagaacca gctggggctc gagatccact agttctagcc tcgaggctag
3840agcggccggc cctataactt cgtataatgt atgctatacg aagttatcag
gtaagttaac 3900agggcgcgcc cactagggac aggattggtg aca
39331420DNAArtificial SequenceFragment of pD4 including the end of
the AAVS1 right homology arm (RHA) 14tctgctctga cgcgtgtata
201553PRTArtificial SequenceFragment of pD4 comprising T2A and
start of EGFP sequence 15Gly Ser Gly Glu Gly Arg Gly Ser Leu Leu
Thr Cys Gly Asp Val Glu1 5 10 15Glu Asn Pro Gly Pro Met Val Ser Lys
Gly Glu Glu Leu Phe Thr Gly 20 25 30Val Val Pro Ile Leu Val Glu Leu
Asp Gly Asp Val Asn Gly His Lys 35 40 45Phe Ser Val Ser Gly
501669PRTArtificial SequenceFragment of pD4 comprising terminal
part of EGFP sequence and Mu ornithine decarboxylase PEST sequence
16Asn Glu Lys Arg Asp His Met Val Leu Leu Glu Phe Val Thr Ala Ala1
5 10 15Gly Ile Thr His Gly Met Asp Glu Leu Tyr Lys Lys Leu Ser His
Gly 20 25 30Phe Pro Pro Ala Val Ala Ala Gln Asp Asp Gly Thr Leu Pro
Met Ser 35 40 45Cys Ala Gln Glu Ser Gly Met Asp Arg His Pro Ala Ala
Cys Ala Ser 50 55 60Ala Arg Ile Asn Val651718PRTArtificial
SequenceInitial fragment of of purodelta TK sequence encoded by pD4
17Met Gly Thr Glu Tyr Lys Pro Thr Val Arg Leu Ala Thr Arg Asp Asp1
5 10 15Val Pro1815PRTArtificial SequenceTerminal fragment of
puromycin delta TK sequence encoded by pD4 18Cys Asp Leu Ala Arg
Thr Phe Ala Arg Glu Met Gly Glu Ala Asn1 5 10 15191098DNAArtificial
SequenceSequence of donor plasmid for integration into Flp/GFP
TALEN sites (pD5), fragment preceding D1.3 antibody expression
cassette 19ggtaccgaat tcctagctct tccagccccc tgtcatggca tcttccaggg
gtccgagagc 60tcagctagtc ttcttcctcc aacccgggcc cctatgtcca cttcaggaca
gcatgtttgc 120tgcctccagg gatcctgtgt ccccgagctg ggaccacctt
atattcccag ggccggttaa 180tgtggctctg gttctgggta cttttatctg
tcccctccac cccacagtgg ggcaagatgc 240atgaagttcc tattccgaag
ttcctattct ctagaaagta taggaacttc gaccataact 300tcgtataaag
tatcctatac gaagttatgc gatcgctcgc gcgtagggat aacagggtaa
360taagtccacc ggtcgccacc atggtcttct gacctcttct cttcctccca
cagggcatgg 420caaaacctct gagccaggaa gaaagcacac tgattgaaag
agcaaccgct actatcaaca 480gcatccccat ctccgaagac tattctgtgg
ctagtgccgc tctgtccagc gacgggagaa 540tcttcaccgg tgtgaacgtc
taccacttta caggcggacc atgcgcagag ctggtggtcc 600tggggactgc
agccgctgca gccgctggta atctgacctg tatcgtggcc attggcaacg
660aaaatagggg catcctgtcc ccatgcggca ggtgtcggca ggtgctgctg
gatctgcatc 720ctggcatcaa ggcaattgtc aaagactctg atggacagcc
taccgccgtc ggtatccgtg 780aactgctgcc tagcggctat gtctgggagg
gataatgaat gagcttggct tcgaaatgac 840cgaccaagcg acgcccaacc
tgccatcacg agatttcgat tccaccgccg ccttctatga 900aaggttgggc
ttcggaatcg ttttccggga cgccggctgg atgatcctcc agcgcgggga
960tctcatgctg gagttcttcg cccaccccaa cttgtttatt gcagcttata
atggttacaa 1020ataaagcaat agcatcacaa atttcacaaa taaagcattt
ttttcactgc attctagttg 1080tggtttaatt aacaattc
109820931DNAArtificial SequenceSequence of donor plasmid for
integration into Flp/GFP TALEN sites (pD5), fragment following D1.3
antibody expression cassette 20caggcatgct ggggatggcc cgggcatgat
aacttcgtat aatgtatgct atacgaagtt 60atgtatacgg cgcgcccgag caagggcgag
gagctgttca cttctgacct cttctcttcc 120tcccacctga gcctagagag
atctggcagc ggagagggca gaggaagtct tctaacatgc 180ggtgacgtgg
aggagaatcc cggaccgtga gtgagcaagg gagaagaact cttcaccggg
240gtggtgccca tcctggtcga gctggacggc gacgtgaacg gccacaagtt
cagcgtgtcc 300ggcgagggcg agggcgatgc cacctacggc aagctgaccc
tgaagttcat ctgcaccacc 360ggcaagctgc ccgtgccctg gcccaccctc
gtgaccaccc tgacctacgg cgtgcagtgc 420ttcagccgct accccgacca
catgaagcag cacgacttct tcaagtccgc catgcccgaa 480ggctacgtcc
aggagcgcac catcttcttc aaggacgacg gcaactacaa gacccgcgcc
540gaggtgaagt tcgagggcga caccctggtg aaccgcatcg agctgaaggg
catcgacttc 600aaggaggacg gcaacatcct ggggcacaag ctggagtaca
actacaacag ccacaacgtc 660tatatcatgg ccgacaagca gaagaacggc
atcaaggtga acttcaagat ccgccacaac 720atcgaggacg gcagcgtgca
gctcgccgac cactaccagc agaacacccc catcggcgac 780ggccccgtgc
tgctgcccga caaccactac ctgagcaccc agtccgccct gagcaaagac
840cccaacgaga agcgcgatca catggtcctg ctggagttcg tgaccgccgc
cgggatcact 900cacggcatgg acgagcctga cgcgtgtata c
93121131PRTArtificial SequenceSequence of Blasticidin resistance
protein encoded by donor plasmid for integration into FLP/GFP TALEN
sites (pD5) 21Ala Lys Pro Leu Ser Gln Glu Glu Ser Thr Leu Ile Glu
Arg Ala Thr1 5 10 15Ala Thr Ile Asn Ser Ile Pro Ile Ser Glu Asp Tyr
Ser Val Ala Ser 20 25 30Ala Ala Leu Ser Ser Asp Gly Arg Ile Phe Thr
Gly Val Asn Val Tyr 35 40 45His Phe Thr Gly Gly Pro Cys Ala Glu Leu
Val Val Leu Gly Thr Ala 50 55 60Ala Ala Ala Ala Ala Gly Asn Leu Thr
Cys Ile Val Ala Ile Gly Asn65 70 75 80Glu Asn Arg Gly Ile Leu Ser
Pro Cys Gly Arg Cys Arg Gln Val Leu 85 90 95Leu Asp Leu His Pro Gly
Ile Lys Ala Ile Val Lys Asp Ser Asp Gly 100 105 110Gln Pro Thr Ala
Val Gly Ile Arg Glu Leu Leu Pro Ser Gly Tyr Val 115 120 125Trp Glu
Gly 13022813DNAArtificial SequenceSequence of I-Sce-1 meganuclease
construct 22ccatgggcta tccttacgat gtccctgatt acgccaacag tcctggtatc
cctggtatgg 60gtcctaaaaa gaagcgaaaa gtgggtagac tggaacccgg catgaagaac
attaagaaaa 120atcaggtgat gaacctggga cctaattcca agctgctgaa
agagtacaag tctcagctga 180tcgaactgaa cattgagcag tttgaagcag
ggatcggtct gattctgggg gacgcctaca 240tccggagcag ggatgagggc
aagacttatt gcatgcagtt cgaatggaag aataaggcct 300acatggacca
cgtgtgtctg ctgtatgatc agtgggtcct gtctccccct cacaagaaag
360agagagtgaa ccatctgggc aatctggtca ttacttgggg agcacagacc
ttcaagcatc 420aggcctttaa caaactggct aacctgttca tcgtgaacaa
caagaaaacc atccctaaca 480atctggtcga aaactacctg acaccaatga
gtctggccta ttggttcatg gacgatggcg 540gaaaatggga ctacaacaag
aacagcacaa acaaaagcat cgtgctgaat acccagtcct 600tcacatttga
ggaagtggag tatctggtca agggcctgcg gaacaaattc cagctgaact
660gctacgtgaa gatcaacaag aacaagccaa tcatctacat cgattctatg
agttacctga 720tcttttataa cctgattaag ccatacctga tcccccagat
gatgtataaa ctgcccaata 780caatcagctc cgagactttc ctgaaggtct aga
81323269PRTArtificial SequenceSequence encoded by I-Sce1
meganuclease construct HA tag, NLS, and I-Sce1 23Met Gly Tyr Pro
Tyr Asp Val Pro Asp Tyr Ala Asn Ser Pro Gly Ile1 5 10 15Pro Gly Met
Gly Pro Lys Lys Lys Arg Lys Val Gly Arg Leu Glu Pro 20 25 30Gly Met
Lys Asn Ile Lys Lys Asn Gln Val Met Asn Leu Gly Pro Asn 35 40 45Ser
Lys Leu Leu Lys Glu Tyr Lys Ser Gln Leu Ile Glu Leu Asn Ile 50 55
60Glu Gln Phe Glu Ala Gly Ile Gly Leu Ile Leu Gly Asp Ala Tyr Ile65
70 75 80Arg Ser Arg Asp Glu Gly Lys Thr Tyr Cys Met Gln Phe Glu Trp
Lys 85 90 95Asn Lys Ala Tyr Met Asp His Val Cys Leu Leu Tyr Asp Gln
Trp Val 100 105 110Leu Ser Pro Pro His Lys Lys Glu Arg Val Asn His
Leu Gly Asn Leu 115 120 125Val Ile Thr Trp Gly Ala Gln Thr Phe Lys
His Gln Ala Phe Asn Lys 130 135 140Leu Ala Asn Leu Phe Ile Val Asn
Asn Lys Lys Thr Ile Pro Asn Asn145 150 155 160Leu Val Glu Asn Tyr
Leu Thr Pro Met Ser Leu Ala Tyr Trp Phe Met 165 170 175Asp Asp Gly
Gly Lys Trp Asp Tyr Asn Lys Asn Ser Thr Asn Lys Ser 180 185 190Ile
Val Leu Asn Thr Gln Ser Phe Thr Phe Glu Glu Val Glu Tyr Leu 195 200
205Val Lys Gly Leu Arg Asn Lys Phe Gln Leu Asn Cys Tyr Val Lys Ile
210 215 220Asn Lys Asn Lys Pro Ile Ile Tyr Ile Asp Ser Met Ser Tyr
Leu Ile225 230 235 240Phe Tyr Asn Leu Ile Lys Pro Tyr Leu Ile Pro
Gln Met Met Tyr Lys 245 250 255Leu Pro Asn Thr Ile Ser Ser Glu Thr
Phe Leu Lys Val 260 2652467DNAArtificial SequencepINT20 Vector
fragment showing Nsi1 site, splice acceptor site and start of
Puromycin resistance gene sequence 24atgcatcttc tgacctcttc
tcttcctccc acagggcatg accgagtaca agcccacggt 60gcgcctc
672510PRTArtificial SequenceInitial fragment of puromycin
resistance protein encoded by pINT20 vector 25Met Thr Glu Tyr Lys
Pro Thr Val Arg Leu1 5 10261125DNAArtificial SequenceSequence of T
cell receptor clone c12/c2 alpha chain construct containing Nhe1,
Not1 and Acc651 restriction sites 26gctagcaagc aggaagtgac
tcagatccca gccgctctga gcgtgcctga gggagaaaac 60ctggtcctga attgcagttt
caccgactca gccatctata acctgcagtg gtttcgccag 120gatccaggca
agggactgac ctccctgctg ctgattcaga gctcccagag ggaacagaca
180tctggcagac tgaatgctag tctggacaaa tctagtggac ggtctaccct
gtacatcgca 240gccagccagc ctggagattc cgcaacatat ctgtgcgccg
tgcgcccact tacaggcgga 300agctacattc ccaccttcgg gcgaggtaca
agcctgatcg tgcacccaga catccagaat 360ccggagcccg ccgtatacca
gctgaaggac cccagaagcc aggacagcac cctgtgcctg 420ttcaccgact
tcgacagcca gatcaacgtg cccaagacaa tggaaagcgg caccttcatc
480accgacaaga ccgtgctgga catgaaggct atggacagca agagcaacgg
cgccattgcc 540tggtccaacc agaccagctt cacatgccag gacatcttca
aagagacaaa cgccacctac 600cccagcagcg acgtgccctg tgatgccacc
ctgaccgaga agtccttcga gacagacatg 660aacctgaact tccagaacct
gtccgcggcc gcaggcctgc tggatcccaa gctgtgctac 720ctgctggacg
ggatcctgtt catctacggt gtgatcctga ctgccctgtt cctgcgagtc
780aaattttctc ggagtgccga cgctcctgca taccagcagg ggcagaacca
gctgtataac 840gagctgaatc tgggtcggag ggaggaatat gacgtgctgg
ataagagacg cggcagggat 900ccagaaatgg ggggcaagcc ccagcgacgg
aaaaaccctc aggagggact gtataatgaa 960ctgcagaagg acaaaatggc
cgaggcttac tctgaaattg ggatgaaggg cgagaggaga 1020cgcggcaaag
gacacgatgg cctgtaccag ggactgagca ctgctaccaa ggacacatat
1080gatgctctgc atatgcaggc actgccccct agataataag gtacc
112527369PRTArtificial SequenceSequence of T cell receptor clone
c12/c2 alpha chain 27Lys Gln Glu Val Thr Gln Ile Pro Ala Ala Leu
Ser Val Pro Glu Gly1 5 10 15Glu Asn Leu Val Leu Asn Cys Ser Phe Thr
Asp Ser Ala Ile Tyr Asn 20 25 30Leu Gln Trp Phe Arg Gln Asp Pro
Gly Lys Gly Leu Thr Ser Leu Leu 35 40 45Leu Ile Gln Ser Ser Gln Arg
Glu Gln Thr Ser Gly Arg Leu Asn Ala 50 55 60Ser Leu Asp Lys Ser Ser
Gly Arg Ser Thr Leu Tyr Ile Ala Ala Ser65 70 75 80Gln Pro Gly Asp
Ser Ala Thr Tyr Leu Cys Ala Val Arg Pro Leu Thr 85 90 95Gly Gly Ser
Tyr Ile Pro Thr Phe Gly Arg Gly Thr Ser Leu Ile Val 100 105 110His
Pro Asp Ile Gln Asn Pro Glu Pro Ala Val Tyr Gln Leu Lys Asp 115 120
125Pro Arg Ser Gln Asp Ser Thr Leu Cys Leu Phe Thr Asp Phe Asp Ser
130 135 140Gln Ile Asn Val Pro Lys Thr Met Glu Ser Gly Thr Phe Ile
Thr Asp145 150 155 160Lys Thr Val Leu Asp Met Lys Ala Met Asp Ser
Lys Ser Asn Gly Ala 165 170 175Ile Ala Trp Ser Asn Gln Thr Ser Phe
Thr Cys Gln Asp Ile Phe Lys 180 185 190Glu Thr Asn Ala Thr Tyr Pro
Ser Ser Asp Val Pro Cys Asp Ala Thr 195 200 205Leu Thr Glu Lys Ser
Phe Glu Thr Asp Met Asn Leu Asn Phe Gln Asn 210 215 220Leu Ser Ala
Ala Ala Gly Leu Leu Asp Pro Lys Leu Cys Tyr Leu Leu225 230 235
240Asp Gly Ile Leu Phe Ile Tyr Gly Val Ile Leu Thr Ala Leu Phe Leu
245 250 255Arg Val Lys Phe Ser Arg Ser Ala Asp Ala Pro Ala Tyr Gln
Gln Gly 260 265 270Gln Asn Gln Leu Tyr Asn Glu Leu Asn Leu Gly Arg
Arg Glu Glu Tyr 275 280 285Asp Val Leu Asp Lys Arg Arg Gly Arg Asp
Pro Glu Met Gly Gly Lys 290 295 300Pro Gln Arg Arg Lys Asn Pro Gln
Glu Gly Leu Tyr Asn Glu Leu Gln305 310 315 320Lys Asp Lys Met Ala
Glu Ala Tyr Ser Glu Ile Gly Met Lys Gly Glu 325 330 335Arg Arg Arg
Gly Lys Gly His Asp Gly Leu Tyr Gln Gly Leu Ser Thr 340 345 350Ala
Thr Lys Asp Thr Tyr Asp Ala Leu His Met Gln Ala Leu Pro Pro 355 360
365Arg281226DNAArtificial SequenceSequence of T cell receptor clone
c12/c2 beta chain construct containing Nco1, Xho1 and Hind3
restriction sites 28ccatggccaa cgctggagtg actcagaccc ctaagttcca
ggtcctgaaa actgggcaga 60gtatgaccct gcagtgcgca caggacatga atcacgagta
catgtcatgg tatcggcagg 120atccagggat gggtctgagg ctgatccatt
acagcgtggg cgctggaact accgaccagg 180gcgaggtgcc caacggatat
aatgtctcaa gaagcaccac agaagatttc ccactgcgac 240tgctgagcgc
cgctcctagc cagacatccg tgtacttttg cgccagctcc aatgtcggga
300acaccggcga gctgttcttt ggggaaggtt cccgcctgac agtgctcgag
gacctgagaa 360acgtgacccc ccccaaggtg tccctgttcg agcctagcaa
ggccgagatc gccaacaagc 420agaaagccac cctcgtgtgc ctggccagag
gcttcttccc cgaccacgtg gaactgtctt 480ggtgggtcaa cggcaaagag
gtgcacagcg gcgtgtccac cgatccccag gcctacaaag 540agagcaacta
cagctactgc ctgagcagca gactgcgggt gtccgccacc ttctggcaca
600acccccggaa ccacttcaga tgccaggtgc agtttcacgg cctgagcgaa
gaggacaagt 660ggcccgaggg cagccctaag cccgtgaccc agaatatctc
tgccgaagcc tggggcagag 720ccgactgtgg cattaccagc gccagctacc
agcagggcgt gctgtctgcc accatcctgt 780acgaggtcgc gagcggactg
ctggacccaa agctgtgcta cctgctggat gggatcctgt 840tcatctacgg
tgtgattctg acagccctgt tcctgcgagt caagttcagc cggagcgccg
900acgcaccagc ataccagcag gggcagaatc agctgtataa cgagctgaat
ctgggtcgga 960gggaggaata cgacgtgctg gataagagac gcggcaggga
tcccgaaatg ggcggaaagc 1020ctcagcgacg gaaaaaccca caggagggac
tgtacaatga actgcagaag gacaaaatgg 1080ctgaggcata ttctgaaatc
ggcatgaagg gagagaggag acgcggcaaa ggacacgatg 1140ggctgtacca
gggtctgagt acagccacta aggacaccta tgatgccctg catatgcagg
1200ctctgccacc cagataataa aagctt 122629404PRTArtificial
SequenceSequence of T cell receptor clone c12/c2 beta chain
construct 29Met Ala Asn Ala Gly Val Thr Gln Thr Pro Lys Phe Gln Val
Leu Lys1 5 10 15Thr Gly Gln Ser Met Thr Leu Gln Cys Ala Gln Asp Met
Asn His Glu 20 25 30Tyr Met Ser Trp Tyr Arg Gln Asp Pro Gly Met Gly
Leu Arg Leu Ile 35 40 45His Tyr Ser Val Gly Ala Gly Thr Thr Asp Gln
Gly Glu Val Pro Asn 50 55 60Gly Tyr Asn Val Ser Arg Ser Thr Thr Glu
Asp Phe Pro Leu Arg Leu65 70 75 80Leu Ser Ala Ala Pro Ser Gln Thr
Ser Val Tyr Phe Cys Ala Ser Ser 85 90 95Asn Val Gly Asn Thr Gly Glu
Leu Phe Phe Gly Glu Gly Ser Arg Leu 100 105 110Thr Val Leu Glu Asp
Leu Arg Asn Val Thr Pro Pro Lys Val Ser Leu 115 120 125Phe Glu Pro
Ser Lys Ala Glu Ile Ala Asn Lys Gln Lys Ala Thr Leu 130 135 140Val
Cys Leu Ala Arg Gly Phe Phe Pro Asp His Val Glu Leu Ser Trp145 150
155 160Trp Val Asn Gly Lys Glu Val His Ser Gly Val Ser Thr Asp Pro
Gln 165 170 175Ala Tyr Lys Glu Ser Asn Tyr Ser Tyr Cys Leu Ser Ser
Arg Leu Arg 180 185 190Val Ser Ala Thr Phe Trp His Asn Pro Arg Asn
His Phe Arg Cys Gln 195 200 205Val Gln Phe His Gly Leu Ser Glu Glu
Asp Lys Trp Pro Glu Gly Ser 210 215 220Pro Lys Pro Val Thr Gln Asn
Ile Ser Ala Glu Ala Trp Gly Arg Ala225 230 235 240Asp Cys Gly Ile
Thr Ser Ala Ser Tyr Gln Gln Gly Val Leu Ser Ala 245 250 255Thr Ile
Leu Tyr Glu Val Ala Ser Gly Leu Leu Asp Pro Lys Leu Cys 260 265
270Tyr Leu Leu Asp Gly Ile Leu Phe Ile Tyr Gly Val Ile Leu Thr Ala
275 280 285Leu Phe Leu Arg Val Lys Phe Ser Arg Ser Ala Asp Ala Pro
Ala Tyr 290 295 300Gln Gln Gly Gln Asn Gln Leu Tyr Asn Glu Leu Asn
Leu Gly Arg Arg305 310 315 320Glu Glu Tyr Asp Val Leu Asp Lys Arg
Arg Gly Arg Asp Pro Glu Met 325 330 335Gly Gly Lys Pro Gln Arg Arg
Lys Asn Pro Gln Glu Gly Leu Tyr Asn 340 345 350Glu Leu Gln Lys Asp
Lys Met Ala Glu Ala Tyr Ser Glu Ile Gly Met 355 360 365Lys Gly Glu
Arg Arg Arg Gly Lys Gly His Asp Gly Leu Tyr Gln Gly 370 375 380Leu
Ser Thr Ala Thr Lys Asp Thr Tyr Asp Ala Leu His Met Gln Ala385 390
395 400Leu Pro Pro Arg30677DNAArtificial SequenceSequence of T cell
receptor clone 4JFH alpha chain construct with initial Nhe1 and
terminal Not1 restriction sites 30gctagccaga aagaggtgga acagaacagc
ggccctctga gcgtgccaga aggcgctatc 60gccagcctga actgcaccta cagctttctg
ggcagccaga gcttcttctg gtacagacag 120tacagcggca agagccccga
gctgatcatg ttcacctaca gagagggcga caaagaggac 180ggcagattca
ccgcccagct gaacaaggcc agccagcacg tgtccctgct gatcagagac
240agccagccta gcgacagcgc cacctacctg tgcgccgtga atgatggcgg
cagactgacc 300tttggcgacg gcaccaccct gaccgtgaag cctgacatcc
agaatccgga gcccgccgta 360taccagctga aggaccccag aagccaggac
agcaccctgt gcctgttcac cgacttcgac 420agccagatca acgtgcccaa
gacaatggaa agcggcacct tcatcaccga caagaccgtg 480ctggacatga
aggctatgga cagcaagagc aacggcgcca ttgcctggtc caaccagacc
540agcttcacat gccaggacat cttcaaagag acaaacgcca cctaccccag
cagcgacgtg 600ccctgtgatg ccaccctgac cgagaagtcc ttcgagacag
acatgaacct gaacttccag 660aacctgtccg cggccgc 67731221PRTArtificial
SequenceSequence of T cell receptor clone 4JFH alpha chain
construct 31Gln Lys Glu Val Glu Gln Asn Ser Gly Pro Leu Ser Val Pro
Glu Gly1 5 10 15Ala Ile Ala Ser Leu Asn Cys Thr Tyr Ser Phe Leu Gly
Ser Gln Ser 20 25 30Phe Phe Trp Tyr Arg Gln Tyr Ser Gly Lys Ser Pro
Glu Leu Ile Met 35 40 45Phe Thr Tyr Arg Glu Gly Asp Lys Glu Asp Gly
Arg Phe Thr Ala Gln 50 55 60Leu Asn Lys Ala Ser Gln His Val Ser Leu
Leu Ile Arg Asp Ser Gln65 70 75 80Pro Ser Asp Ser Ala Thr Tyr Leu
Cys Ala Val Asn Asp Gly Gly Arg 85 90 95Leu Thr Phe Gly Asp Gly Thr
Thr Leu Thr Val Lys Pro Asp Ile Gln 100 105 110Asn Pro Glu Pro Ala
Val Tyr Gln Leu Lys Asp Pro Arg Ser Gln Asp 115 120 125Ser Thr Leu
Cys Leu Phe Thr Asp Phe Asp Ser Gln Ile Asn Val Pro 130 135 140Lys
Thr Met Glu Ser Gly Thr Phe Ile Thr Asp Lys Thr Val Leu Asp145 150
155 160Met Lys Ala Met Asp Ser Lys Ser Asn Gly Ala Ile Ala Trp Ser
Asn 165 170 175Gln Thr Ser Phe Thr Cys Gln Asp Ile Phe Lys Glu Thr
Asn Ala Thr 180 185 190Tyr Pro Ser Ser Asp Val Pro Cys Asp Ala Thr
Leu Thr Glu Lys Ser 195 200 205Phe Glu Thr Asp Met Asn Leu Asn Phe
Gln Asn Leu Ser 210 215 22032353DNAArtificial SequenceSequence of T
cell receptor clone4JFH beta chain construct with initial Nco1
restriction site and terminal Xho1 restriction site 32ccatggccag
ccagaccatc catcagtggc ctgccaccct ggtgcagcct gtgggatctc 60ctctgagcct
ggaatgcacc gtggaaggca ccagcaaccc caacctgtac tggtacagac
120aggccgctgg cagaggcccc cagctgctgt tttactgggg cccctttggc
cagatcagca 180gcgaggtgcc ccagaacctg agcgccagca gaccccagga
ccggcagttt atcctgagca 240gcaagaagct gctgctgagc gacagcggct
tctacctgtg cgcttggagc gagacaggcc 300tgggcatggg cggatggcag
tttggcgagg gcagcagact gacagtgctc gag 35333117PRTArtificial
SequenceSequence of T cell receptor clone 4JFH beta chain construct
33Met Ala Ser Gln Thr Ile His Gln Trp Pro Ala Thr Leu Val Gln Pro1
5 10 15Val Gly Ser Pro Leu Ser Leu Glu Cys Thr Val Glu Gly Thr Ser
Asn 20 25 30Pro Asn Leu Tyr Trp Tyr Arg Gln Ala Ala Gly Arg Gly Pro
Gln Leu 35 40 45Leu Phe Tyr Trp Gly Pro Phe Gly Gln Ile Ser Ser Glu
Val Pro Gln 50 55 60Asn Leu Ser Ala Ser Arg Pro Gln Asp Arg Gln Phe
Ile Leu Ser Ser65 70 75 80Lys Lys Leu Leu Leu Ser Asp Ser Gly Phe
Tyr Leu Cys Ala Trp Ser 85 90 95Glu Thr Gly Leu Gly Met Gly Gly Trp
Gln Phe Gly Glu Gly Ser Arg 100 105 110Leu Thr Val Leu Glu
1153453DNAArtificial SequencePrimer for mutation of CDR3 of c12/c2
TCR alpha chainmisc_feature(20)..(21)n is a, c, g, or
tmisc_feature(23)..(24)n is a, c, g, or tmisc_feature(31)..(31)n is
a, c, g, or t 34cacgcggcac gcgggtgaan nsnnscctwc natgtaaggg
tggaagcccg ctc 533552DNAArtificial SequencePrimer used to mutate
CDR3 of c12/c2 TCR alpha chainmisc_feature(23)..(23)n is a, c, g,
or tmisc_feature(30)..(31)n is a, c, g, or tmisc_feature(33)..(34)n
is a, c, g, or t 35ctcgcccgaa ggtgggaatg tangwtccsn nsnnaagtgg
gcgcacggcg ca 523617PRTArtificial SequenceStrategy used to mutate
CDR3 of c12/c2 TCR alpha chainmisc_feature(7)..(8)Xaa can be any
naturally occurring amino acid 36Cys Ala Val Arg Pro Leu Xaa Xaa
Gly Ser Tyr Ile Pro Thr Phe Gly1 5 10 15Arg3717PRTArtificial
SequenceStrategy used to mutate CDR3 of c12/c2 TCR alpha
chainmisc_feature(7)..(8)Xaa can be any naturally occurring amino
acid 37Cys Ala Val Arg Pro Leu Xaa Xaa Gly Thr Tyr Ile Pro Thr Phe
Gly1 5 10 15Arg3850DNAArtificial SequencePrimer used to mutate CDR3
of c12/c2 TCR beta chainmisc_feature(20)..(21)n is a, c, g, or
tmisc_feature(29)..(30)n is a, c, g, or t 38gtacttttgc gccagctccn
nsstcgggnn saccggcgag ctgttctttg 503949DNAArtificial SequencePrimer
used to mutate CDR3 of c12/c2 TCR beta chainmisc_feature(21)..(22)n
is a, c, g, or tmisc_feature(30)..(31)n is a, c, g, or t
39caaagaacag ctcgccggts nncccgassn nggagctggc gcaaaagta
494016PRTArtificial SequenceStrategy used to mutate CDR3 of c12/c2
TCR beta chain 40Tyr Phe Cys Ala Ser Ser Asn Val Gly Asn Thr Gly
Glu Leu Phe Phe1 5 10 154116PRTArtificial SequenceStrategy used to
mutate CDR3 of c12/c2 TCR beta chain 41Tyr Phe Cys Ala Ser Ser Asn
Leu Gly Asn Thr Gly Glu Leu Phe Phe1 5 10 1542462DNAArtificial
SequenceSequence of CD3 zeta in pINT21 CAR1 42ggcgccatgg cccaggtcgc
ggccgcaagc ggactgctgg acccaaagct gtgctacctg 60ctggatggga tcctgttcat
ctacggtgtg attctgacag ccctgttcct gcgagtcaag 120ttcagccgga
gcgccgacgc accagcatac cagcaggggc agaatcagct gtataacgag
180ctgaatctgg gtcggaggga ggaatacgac gtgctggata agagacgcgg
cagggatccc 240gaaatgggcg gaaagcctca gcgacggaaa aacccacagg
agggactgta caatgaactg 300cagaaggaca aaatggctga ggcatattct
gaaatcggca tgaagggaga gaggagacgc 360ggcaaaggac acgatgggct
gtaccagggt ctgagtacag ccactaagga cacctatgat 420gccctgcata
tgcaggctct gccacccaga taataaaagc tt 462434PRTArtificial
SequenceFragment encoded by pINT21 CAR1 vector 43Gly Ala Met
Ala144141PRTArtificial SequenceCDR3 zeta sequence encoded by pINT21
CAR1 44Ser Gly Leu Leu Asp Pro Lys Leu Cys Tyr Leu Leu Asp Gly Ile
Leu1 5 10 15Phe Ile Tyr Gly Val Ile Leu Thr Ala Leu Phe Leu Arg Val
Lys Phe 20 25 30Ser Arg Ser Ala Asp Ala Pro Ala Tyr Gln Gln Gly Gln
Asn Gln Leu 35 40 45Tyr Asn Glu Leu Asn Leu Gly Arg Arg Glu Glu Tyr
Asp Val Leu Asp 50 55 60Lys Arg Arg Gly Arg Asp Pro Glu Met Gly Gly
Lys Pro Gln Arg Arg65 70 75 80Lys Asn Pro Gln Glu Gly Leu Tyr Asn
Glu Leu Gln Lys Asp Lys Met 85 90 95Ala Glu Ala Tyr Ser Glu Ile Gly
Met Lys Gly Glu Arg Arg Arg Gly 100 105 110Lys Gly His Asp Gly Leu
Tyr Gln Gly Leu Ser Thr Ala Thr Lys Asp 115 120 125Thr Tyr Asp Ala
Leu His Met Gln Ala Leu Pro Pro Arg 130 135 14045705DNAArtificial
SequencepINT21 CAR2 Sequence encoding CD3 zeta 45gccatggccc
aggtcgcggc cgcaacaaca accccagccc ccagacctcc tacccctgcc 60cctacaattg
ccagccagcc tctgagcctg aggcccgagg cttgtagacc agctgctggc
120ggagccgtgc acaccagagg actggatttc gcctgcgaca tctacatctg
ggcccctctg 180gccggcacat gtggcgtgct gctgctgagc ctcgtgatca
ccctgtactg caagcggggc 240agaaagaaac tgctgtacat ctttaagcag
cccttcatgc ggcccgtgca gaccacccag 300gaagaggacg gctgctcctg
cagattcccc gaggaagaag aaggcggctg cgagctgaga 360gtgaagttca
gcagatccgc cgacgcccct gcctacaagc agggccagaa ccagctgtac
420aacgagctga acctgggcag acgggaagag tacgacgtgc tggacaagcg
gagaggccgg 480gacccagaga tgggcggaaa gcccagaaga aagaaccccc
aggaaggcct gtataacgaa 540ctgcagaaag acaaaatggc cgaggcctac
agcgagatcg gaatgaaggg cgagcggaga 600agaggcaagg ggcacgatgg
cctgtaccag ggcctgagca ccgccaccaa ggacacctat 660gacgccctgc
acatgcaggc cctgccccct agataataaa agctt 70546223PRTArtificial
SequenceSequence encoded by pINT21 CAR2 CD8 hinge / transmembrane
region, 4-1BB and CD3 zeta 46Thr Thr Thr Pro Ala Pro Arg Pro Pro
Thr Pro Ala Pro Thr Ile Ala1 5 10 15Ser Gln Pro Leu Ser Leu Arg Pro
Glu Ala Cys Arg Pro Ala Ala Gly 20 25 30Gly Ala Val His Thr Arg Gly
Leu Asp Phe Ala Cys Asp Ile Tyr Ile 35 40 45Trp Ala Pro Leu Ala Gly
Thr Cys Gly Val Leu Leu Leu Ser Leu Val 50 55 60Ile Thr Leu Tyr Cys
Lys Arg Gly Arg Lys Lys Leu Leu Tyr Ile Phe65 70 75 80Lys Gln Pro
Phe Met Arg Pro Val Gln Thr Thr Gln Glu Glu Asp Gly 85 90 95Cys Ser
Cys Arg Phe Pro Glu Glu Glu Glu Gly Gly Cys Glu Leu Arg 100 105
110Val Lys Phe Ser Arg Ser Ala Asp Ala Pro Ala Tyr Lys Gln Gly Gln
115 120 125Asn Gln Leu Tyr Asn Glu Leu Asn Leu Gly Arg Arg Glu Glu
Tyr Asp 130 135 140Val Leu Asp Lys Arg Arg Gly Arg Asp Pro Glu Met
Gly Gly Lys Pro145 150 155 160Arg Arg Lys Asn Pro Gln Glu Gly Leu
Tyr Asn Glu Leu Gln Lys Asp 165 170 175Lys Met Ala Glu Ala Tyr Ser
Glu Ile Gly Met Lys Gly Glu Arg Arg 180 185 190Arg Gly Lys Gly His
Asp Gly Leu Tyr Gln Gly Leu Ser Thr Ala Thr 195 200 205Lys Asp Thr
Tyr Asp Ala Leu His Met Gln Ala Leu Pro Pro Arg 210 215
22047750DNAArtificial SequenceSequence of FMC63
H-L (anti-CD19 antibody) 47gccatggccg aagtgaaact gcaggagtct
ggacccggcc tggtggcccc atctcagtct 60ctgagcgtga cctgtaccgt gtccggcgtg
tccctgcctg actatggcgt gtcctggatc 120agacagcccc ccagaaaggg
cctggaatgg ctgggagtga tctggggcag cgaaaccacc 180tactacaaca
gcgccctgaa gtcccggctg accatcatca aggacaactc caagagccag
240gtgttcctga agatgaacag cctgcagacc gacgacaccg ccatctacta
ctgcgccaag 300cactactact acggcggcag ctacgctatg gactactggg
gccagggcac ctcggtcacc 360gtctcgagtg gtggaggcgg ttcaggcgga
ggtggctctg gcggtggcgc tagcgacatc 420cagatgaccc agaccaccag
cagcctgagc gccagcctgg gcgatagagt gaccatcagc 480tgcagagcca
gccaggacat cagcaagtac ctgaactggt atcagcagaa acccgacggc
540accgtgaagc tgctgatcta ccacaccagc agactgcaca gcggcgtgcc
cagcagattt 600tccggctctg gcagcggcac cgactacagc ctgaccatct
ccaacctgga acaggaagat 660atcgctacct acttctgtca gcaaggcaac
accctgccct acaccttcgg cggagggacc 720aagctggaga tcaaacgtac
cgcggccgca 75048250PRTArtificial SequenceSequence of FMC63 H-L
(anti-CD19 antibody) 48Ala Met Ala Glu Val Lys Leu Gln Glu Ser Gly
Pro Gly Leu Val Ala1 5 10 15Pro Ser Gln Ser Leu Ser Val Thr Cys Thr
Val Ser Gly Val Ser Leu 20 25 30Pro Asp Tyr Gly Val Ser Trp Ile Arg
Gln Pro Pro Arg Lys Gly Leu 35 40 45Glu Trp Leu Gly Val Ile Trp Gly
Ser Glu Thr Thr Tyr Tyr Asn Ser 50 55 60Ala Leu Lys Ser Arg Leu Thr
Ile Ile Lys Asp Asn Ser Lys Ser Gln65 70 75 80Val Phe Leu Lys Met
Asn Ser Leu Gln Thr Asp Asp Thr Ala Ile Tyr 85 90 95Tyr Cys Ala Lys
His Tyr Tyr Tyr Gly Gly Ser Tyr Ala Met Asp Tyr 100 105 110Trp Gly
Gln Gly Thr Ser Val Thr Val Ser Ser Gly Gly Gly Gly Ser 115 120
125Gly Gly Gly Gly Ser Gly Gly Gly Ala Ser Asp Ile Gln Met Thr Gln
130 135 140Thr Thr Ser Ser Leu Ser Ala Ser Leu Gly Asp Arg Val Thr
Ile Ser145 150 155 160Cys Arg Ala Ser Gln Asp Ile Ser Lys Tyr Leu
Asn Trp Tyr Gln Gln 165 170 175Lys Pro Asp Gly Thr Val Lys Leu Leu
Ile Tyr His Thr Ser Arg Leu 180 185 190His Ser Gly Val Pro Ser Arg
Phe Ser Gly Ser Gly Ser Gly Thr Asp 195 200 205Tyr Ser Leu Thr Ile
Ser Asn Leu Glu Gln Glu Asp Ile Ala Thr Tyr 210 215 220Phe Cys Gln
Gln Gly Asn Thr Leu Pro Tyr Thr Phe Gly Gly Gly Thr225 230 235
240Lys Leu Glu Ile Lys Arg Thr Ala Ala Ala 245
25049327DNAArtificial SequenceSequence of lox1 binding Adhiron
lox1A 49gccatggctg ctacaggcgt gcgggctgtg cccggcaatg agaacagcct
ggaaatcgag 60gaactggcca gattcgccgt ggacgagcac aacaagaaag agaacgccct
gctggaattc 120gtgcgggtcg tgaaggccaa agagcagtgg agcgaggccg
acaacgactg gcacaccatg 180tactacctga ccctggaagc caaggacggc
ggcaagaaga agctgtacga ggccaaagtg 240tgggtcaagc tggacctgga
aacctggcag cacttcaact tcaaagagct ccaggaattc 300aagcccgtgg
gcgacgctgc ggccgcg 32750109PRTArtificial SequenceSequence of Lox1
binding Adhiron lox1A 50Ala Met Ala Ala Thr Gly Val Arg Ala Val Pro
Gly Asn Glu Asn Ser1 5 10 15Leu Glu Ile Glu Glu Leu Ala Arg Phe Ala
Val Asp Glu His Asn Lys 20 25 30Lys Glu Asn Ala Leu Leu Glu Phe Val
Arg Val Val Lys Ala Lys Glu 35 40 45Gln Trp Ser Glu Ala Asp Asn Asp
Trp His Thr Met Tyr Tyr Leu Thr 50 55 60Leu Glu Ala Lys Asp Gly Gly
Lys Lys Lys Leu Tyr Glu Ala Lys Val65 70 75 80Trp Val Lys Leu Asp
Leu Glu Thr Trp Gln His Phe Asn Phe Lys Glu 85 90 95Leu Gln Glu Phe
Lys Pro Val Gly Asp Ala Ala Ala Ala 100 10551333DNAArtificial
SequenceSequence of lox1 binding Adhiron lox1B 51gccatggctg
ctacaggcgt gcgggctgtg cccggcaatg agaacagcct ggaaatcgag 60gaactggcca
gattcgccgt ggacgagcac aacaagaaag agaacgccct gctggaattc
120gtgcgggtcg tgaaggccaa agagcaggaa cagcccatcg gcgagcaccc
cgtgaacgac 180accatgtact acctgaccct ggaagccaag gacggcggca
agaagaagct gtacgaggcc 240aaagtgtggg tcaagcggtg gctgcggttc
accgagatct acaacttcaa agagctccag 300gaattcaagc ccgtgggcga
cgctgcggcc gcg 33352111PRTArtificial SequenceSequence of lox1
binding Adhiron lox1B 52Ala Met Ala Ala Thr Gly Val Arg Ala Val Pro
Gly Asn Glu Asn Ser1 5 10 15Leu Glu Ile Glu Glu Leu Ala Arg Phe Ala
Val Asp Glu His Asn Lys 20 25 30Lys Glu Asn Ala Leu Leu Glu Phe Val
Arg Val Val Lys Ala Lys Glu 35 40 45Gln Glu Gln Pro Ile Gly Glu His
Pro Val Asn Asp Thr Met Tyr Tyr 50 55 60Leu Thr Leu Glu Ala Lys Asp
Gly Gly Lys Lys Lys Leu Tyr Glu Ala65 70 75 80Lys Val Trp Val Lys
Arg Trp Leu Arg Phe Thr Glu Ile Tyr Asn Phe 85 90 95Lys Glu Leu Gln
Glu Phe Lys Pro Val Gly Asp Ala Ala Ala Ala 100 105
1105354DNAArtificial SequenceAdhiron_mut1
primermisc_feature(23)..(24)n is a, c, g, or
tmisc_feature(26)..(27)n is a, c, g, or tmisc_feature(29)..(30)n is
a, c, g, or tmisc_feature(32)..(33)n is a, c, g, or t 53cagggtcagg
tagtacatgg tsnnsnnsnn snnctgctct ttggccttca cgac
545448DNAArtificial SequenceAdhiron_mut2
primermisc_feature(20)..(21)n is a, c, g, or
tmisc_feature(23)..(24)n is a, c, g, or tmisc_feature(26)..(27)n is
a, c, g, or tmisc_feature(29)..(30)n is a, c, g, or t 54ctggagctct
ttgaagttsn nsnnsnnsnn cttgacccac actttggc 485554DNAArtificial
SequenceMutagenic primer for construction of library of binders
within loop 1 (adhiron mut 1)misc_feature(22)..(23)n is a, c, g, or
tmisc_feature(25)..(26)n is a, c, g, or tmisc_feature(28)..(29)n is
a, c, g, or tmisc_feature(31)..(32)n is a, c, g, or t 55gtcgtgaagg
ccaaagagca gnnsnnsnns nnsaccatgt actacctgac cctg
545618PRTArtificial SequenceAdhiron mut1 loop library variant
sequencemisc_feature(8)..(11)Xaa can be any naturally occurring
amino acid 56Val Val Lys Ala Lys Glu Gln Xaa Xaa Xaa Xaa Thr Met
Tyr Tyr Leu1 5 10 15Thr Leu5748DNAArtificial SequenceMutagenic
primer for construction of library of binders within Adhiron mut2
loopmisc_feature(19)..(20)n is a, c, g, or tmisc_feature(22)..(23)n
is a, c, g, or tmisc_feature(25)..(26)n is a, c, g, or
tmisc_feature(28)..(29)n is a, c, g, or t 57gccaaagtgt gggtcaagnn
snnsnnsnns aacttcaaag agctccag 485816PRTArtificial SequenceAdhiron
mut 2 loop library variant sequencemisc_feature(7)..(10)Xaa can be
any naturally occurring amino acid 58Ala Lys Val Trp Val Lys Xaa
Xaa Xaa Xaa Asn Phe Lys Glu Leu Gln1 5 10 1559105DNAArtificial
sequenceTrypsin binding Knottin MCoTI-IIsequence 59gccatggccg
gtgtgtgccc caagatcttg aaaaagtgcc gccgtgacag cgattgtccc 60ggcgcctgca
tctgccgcgg caatggctat tgcggagcgg ccgca 1056032PRTArtificial
SequenceTrypsin binding Knottin MCo-TI-II sequence 60Ala Met Ala
Gly Val Cys Pro Lys Ile Leu Lys Lys Cys Arg Arg Asp1 5 10 15Ser Asp
Cys Pro Gly Ala Cys Ile Cys Arg Gly Asn Gly Tyr Cys Gly 20 25
306199DNAArtificial SequencePrimer for creation of library of
Knottin mutantsmisc_feature(11)..(11)n is a, c, g, or
tmisc_feature(14)..(14)n is a, c, g, or tmisc_feature(17)..(17)n is
a, c, g, or tmisc_feature(20)..(20)n is a, c, g, or
tmisc_feature(23)..(23)n is a, c, g, or tmisc_feature(26)..(26)n is
a, c, g, or tmisc_feature(29)..(29)n is a, c, g, or
tmisc_feature(32)..(32)n is a, c, g, or tmisc_feature(35)..(35)n is
a, c, g, or tmisc_feature(38)..(38)n is a, c, g, or t 61ggtgtgtgcv
nsvnsvnsvn svnsvnsvns vnsvnsvnst gccgccgtga cagcgattgt 60cccggcgcct
gcatctgccg cggcaatggc tattgcgga 996299DNAArtificial
SequenceSequence varied in library of knottin
mutantsmisc_feature(62)..(62)n is a, c, g, or
tmisc_feature(65)..(65)n is a, c, g, or tmisc_feature(68)..(68)n is
a, c, g, or tmisc_feature(71)..(71)n is a, c, g, or
tmisc_feature(74)..(74)n is a, c, g, or tmisc_feature(77)..(77)n is
a, c, g, or tmisc_feature(80)..(80)n is a, c, g, or
tmisc_feature(83)..(83)n is a, c, g, or tmisc_feature(86)..(86)n is
a, c, g, or tmisc_feature(89)..(89)n is a, c, g, or t 62tccgcaatag
ccattgccgc ggcagatgca ggcgccggga caatcgctgt cacggcggca 60snbsnbsnbs
nbsnbsnbsn bsnbsnbsnb gcacacacc 996333PRTArtificial
SequenceSequence of Knottin varied in
librarymisc_feature(4)..(13)Xaa can be any naturally occurring
amino acid 63Gly Val Cys Xaa Xaa Xaa Xaa Xaa Xaa Xaa Xaa Xaa Xaa
Cys Arg Arg1 5 10 15Asp Ser Asp Cys Pro Gly Ala Cys Ile Cys Arg Gly
Asn Gly Tyr Cys 20 25 30Gly64121DNAArtificial SequencepD7-Sce1
sequence nucleotides 1 - 120 64ggtaccgaat tctagggata acagggtaat
atgcatcttc tgacctcttc tcttcctccc 60acagggcatg gcaaaacctc tgagccagga
agaaagcaca ctgattgaaa gagcaaccgc 120t 1216518PRTArtificial
SequenceFragment of blasticidin resistance gene encoded by pD7-Sce1
65Met Ala Lys Pro Leu Ser Gln Glu Glu Ser Thr Leu Ile Glu Arg Ala1
5 10 15Thr Ala66120DNAArtificial SequencepD7-ObLiGaRe sequence
fragment 66ggtaccgaat tcttttctgt caccaatcct ggggccacta gggacactgt
ggggtggagg 60aaatgcatct tctgacctct tctcttcctc ccacagggca tggcaaaacc
tctgagccag 120677PRTArtificial SequenceFragment of Blasticidin
resistance gene encoded by pD7-ObLiGaRe 67Met Ala Lys Pro Leu Ser
Gln1 5681061DNAArtificial SequenceROSA 26 left homology arm
sequence 68gcgatcgcgc tgattggctt cttttcctcc cgccgtgtgt gaaaacacaa
atggcgtgtt 60ttggttggcg taaggcgcct gtcagttaac ggcagccgga gtgcgcagcc
gccggcagcc 120tcgctctgcc cactgggtgg ggcgggaggt aggtggggtg
aggcgagctg gacgtgcggg 180cgcggtcggc ctctggcggg gcgggggagg
ggagggaggg tcagcgaaag tagctcgcgc 240gcgagcggcc gcccaccctc
cccttcctct gggggagtcg ttttacccgc cgccggccgg 300gcctcgtcgt
ctgattggct ctcggggccc agaaaactgg cccttgccat tggctcgtgt
360tcgtgcaagt tgagtccatc cgccggccag cgggggcggc gaggaggcgc
tcccaggttc 420cggccctccc ctcggccccg cgccgcagag tctggccgcg
cgcccctgcg caacgtggca 480ggaagcgcgc gctgggggcg gggacgggca
gtagggctga gcggctgcgg ggcgggtgca 540agcacgtttc cgacttgagt
tgcctcaaga ggggcgtgct gagccagacc tccatcgcgc 600actccgggga
gtggagggaa ggagcgaggg ctcagttggg ctgttttgga ggcaggaagc
660acttgctctc ccaaagtcgc tctgagttgt tatcagtaag ggagctgcag
tggagtaggc 720ggggagaagg ccgcaccctt ctccggaggg gggaggggag
tgttgcaata cctttctggg 780agttctctgc tgcctcctgg cttctgagga
ccgccctggg cctgggagaa tcccttcccc 840ctcttccctc gtgatctgca
actccagtct ttctagaatg cattaaggga tctgtagggc 900gcagtagtcc
agggtttcct tgatgatgtc atacttatcc tgtccctttt ttttccacag
960ctcgcggttg aggacaaact cttcgcggtc tttccagtgg ggatcgacgg
tatcgtagag 1020tcgaggccgc tctaggaatt cacgccgcca ccatgaccga g
106169703DNAArtificial SequenceROSA 26 right homology arm sequence
69gtatacggga attgaacagg tgtaaaattg gagggacaag acttcccaca gattttcggt
60tttgtcggga agttttttaa taggggcaaa taaggaaaat gggaggatag gtagtcatct
120ggggttttat gcagcaaaac tacaggttat tattgcttgt gatccgcctc
ggagtatttt 180ccatcgaggt agattaaaga catgctcacc cgagttttat
actctcctgc ttgagatcct 240tactacagta tgaaattaca gtgtcgcgag
ttagactatg taagcagaat tttaatcatt 300tttaaagagc ccagtacttc
atatccattt ctcccgctcc ttctgcagcc ttatcaaaag 360gtattttaga
acactcattt tagccccatt ttcatttatt atactggctt atccaacccc
420tagacagagc attggcattt tccctttcct gatcttagaa gtctgatgac
tcatgaaacc 480agacagatta gttacataca ccacaaatcg aggctgtagc
tggggcctca acactgcagt 540tcttttataa ctccttagta cactttttgt
tgatcctttg ccttgatcct taattttcag 600tgtctatcac ctctcccgtc
aggtggtgtt ccacatttgg gcctattctc agtccaggga 660gttttacaac
aatagatgta ttgagaatcc aacctcctgc agg 7037018DNAArtificial
SequenceLeft TALEN recognition sequence 70tcccctccac cccacagt
187115DNAArtificial SequenceTALEN spacer 71ggggccacta gggac
157218DNAArtificial SequenceRight TALEN target sequence
72aggattggtg acagaaaa 187318DNAArtificial SequenceRight TALEN
sequence 73ttttctgtca ccaatcct 187420DNAArtificial
SequenceAAVS-Left-arm-junction-PCR-Forw (9625) primer 74ccggaactct
gccctctaac 207523DNAArtificial SequenceBSD_Junction PCR-rev (9626)
primer 75tagccacaga atagtcttcg gag 237621DNAArtificial
SequenceDonor_plasmid_seq_PDGFRTM-2 Forw primer 76acacgcagga
ggccatcgtg g 217720DNAArtificial SequenceAAVS1_right
arm_junction_PCR_rev primer 77tcctgggata ccccgaagag
207854DNAArtificial SequencePrimer 2598 78ttttttttaa ttaagattat
tgactagtta ttaatagtaa tcaattacgg ggtc 547952DNAArtificial
SequencePrimer 2619 79ttttttgttt aaacttacct tggatccctt gccggggctc
aggctcaggg ac 528033DNAArtificial SequenceM13Leadseq primer
80aaattattat tcgcaattcc tttggttgtt cct 338130DNAArtificial
SequenceNotmycseq primer 81ggccccattc agatcctctt ctgagatgag
308222DNAArtificial SequencePrimer 2623 82taaagtaggc ggtcttgaga cg
228322DNAArtificial SequencePrimer 2624 83gaaggtgctg ttgaactgtt cc
228419DNAArtificial SequencePrimer 2595 84gagggctctg gcagctagc
198520DNAArtificial SequencePrimer 2597 85tcgagactgt gacgaggctg
208619DNAArtificial SequencePrimer 2625 86ccttggtgct ggcactcga
198740DNAArtificial SequencePrimer 1999 87aaaaagcagg ctaccatgag
ggcctggatc ttctttctcc 408844DNAArtificial SequenceTALEN recognition
sequence 88tccaccggtc gccaccatgg tgagcaaggg cgaggagctg ttca
448933DNAArtificial SequenceAAVS1_HA-L_Nested_Forw1 primer
89gtgcccttgc tgtgccgccg gaactctgcc ctc 339023DNAArtificial
SequenceEGFP_Synthetic_gene_Rev_Assembly primer 90ttcacgtcgc
cgtccagctc gac 239121DNAArtificial SequencePurotk_seq_fow2 primer
91tccataccga cgatctgcga c 219227DNAArtificial SequenceJ44 primer
92aaaagcgcct cccctacccg gtagaat 279327DNAArtificial SequenceJ46
primer 93ggcgacacgg aaatgttgaa tactcat 279427DNAArtificial
SequenceJ48 primer 94cactacaccc agaagtccct gagcctg
279520DNAArtificial SequencepSANG10pelB primer 95cgctgcccag
ccggccatgg 209626DNAArtificial SequencePrimer 2097 96gatggtgatg
atgatgtgcg gatgcg 269738DNAArtificial SequencePrimer 2423
97ttttttccat gggccggccc tccttcagtt tagttgag 389839DNAArtificial
SequencePrimer 2437 98ttttttgcgg ccgcggaagc cgtgatctcc ttctctctc
399920DNAArtificial SequencePrimer 2622 99gaacaggaac acggaaggtc
2010022DNAArtificial SequencePrimer 41679 100atgagttgga gctgtatcat
cc 2210118DNAArtificial SequencePrimer 2621 101gcattccacg gcggccgc
181029PRTArtificial SequencePeptide SLLMWITQV (NY-ESO-1 157-165)
recognised by c12c2 102Ser Leu Leu Met Trp Ile Thr Gln Val1
510310PRTArtificial SequencePeptide MART-1 26-35 recognised by MEL5
TCR 103Glu Leu Ala Gly Ile Gly Ile Leu Thr Val1 5
1010430DNAArtificial SequencePrimer 4JFH-Valpha-F 104acacacgcta
gccagaaaga ggtggaacag 3010550DNAArtificial SequencePrimer
2770misc_feature(21)..(22)n is a, c, g, or tmisc_feature(30)..(31)n
is a, c, g, or t 105caaagaacag ctcgccggts nncccgassn nggagctggc
gcaaaagtac 5010653DNAArtificial SequencePrimer
2771misc_feature(23)..(23)n is a, c, g, or tmisc_feature(30)..(31)n
is a, c, g, or tmisc_feature(33)..(34)n is a, c, g, or t
106ctcgcccgaa ggtgggaatg tangwtccsn nsnnaagtgg gcgcacggcg cac
5310722DNAArtificial SequencePrimer 2780 107ctggcagcta gcaagcagga
ag 2210822DNAArtificial SequencePrimer 2781 108tacattccca
ccttcgggcg ag
2210926DNAArtificial SequencePrimer 2782 109ttttttgcgg ccgcggacag
gttctg 2611027DNAArtificial SequencePrimer 2783 110cgtaagctgg
taccttatta tctaggg 2711127DNAArtificial SequencePrimer 2785
111ccctagataa taaggtacca gcttacg 2711219DNAArtificial
SequencePrimer 2787 112accggcgagc tgttctttg 1911326DNAArtificial
SequencePrimer 2788 113agtgacaagc ttttattatc tgggtg
2611419DNAArtificial SequencePrimer 2789 114caggtcctcg agcactgtc
1911525DNAArtificial SequenceCRISPR1 double-stranded DNA insert
strand 115ggggccacta gggacaggat gtttt 2511626DNAArtificial
SequenceCRISPR1 double-stranded DNA insert strand 116catcctgtcc
ctagtggccc ccggtg 2611725DNAArtificial SequenceCRISPR2
double-stranded DNA insert strand 117gtcaccaatc ctgtccctag gtttt
2511825DNAArtificial SequenceCRISPR2 double-stranded DNA insert
strand 118ctagggacag gattggtgac cggtg 25119102RNAArtificial
SequenceCRISPR 1 RNA 119ggggggccac uagggacagg auguuuuaga gcuagaaaua
gcaaguuaaa auaaggcuag 60uccguuauca acuugaaaaa guggcaccga gucggugcuu
uu 102120102RNAArtificial SequenceCRISPR 2 RNA 120gggucaccaa
uccugucccu agguuu
References