U.S. patent application number 16/044937 was filed with the patent office on 2020-01-30 for 3d printed radiator core.
The applicant listed for this patent is GLOBAL HEAT TRANSFER ULC. Invention is credited to Brian Barney, Hamid Reza Zareie Rajani, Haoxuan Yan.
Application Number | 20200033062 16/044937 |
Document ID | / |
Family ID | 67441712 |
Filed Date | 2020-01-30 |


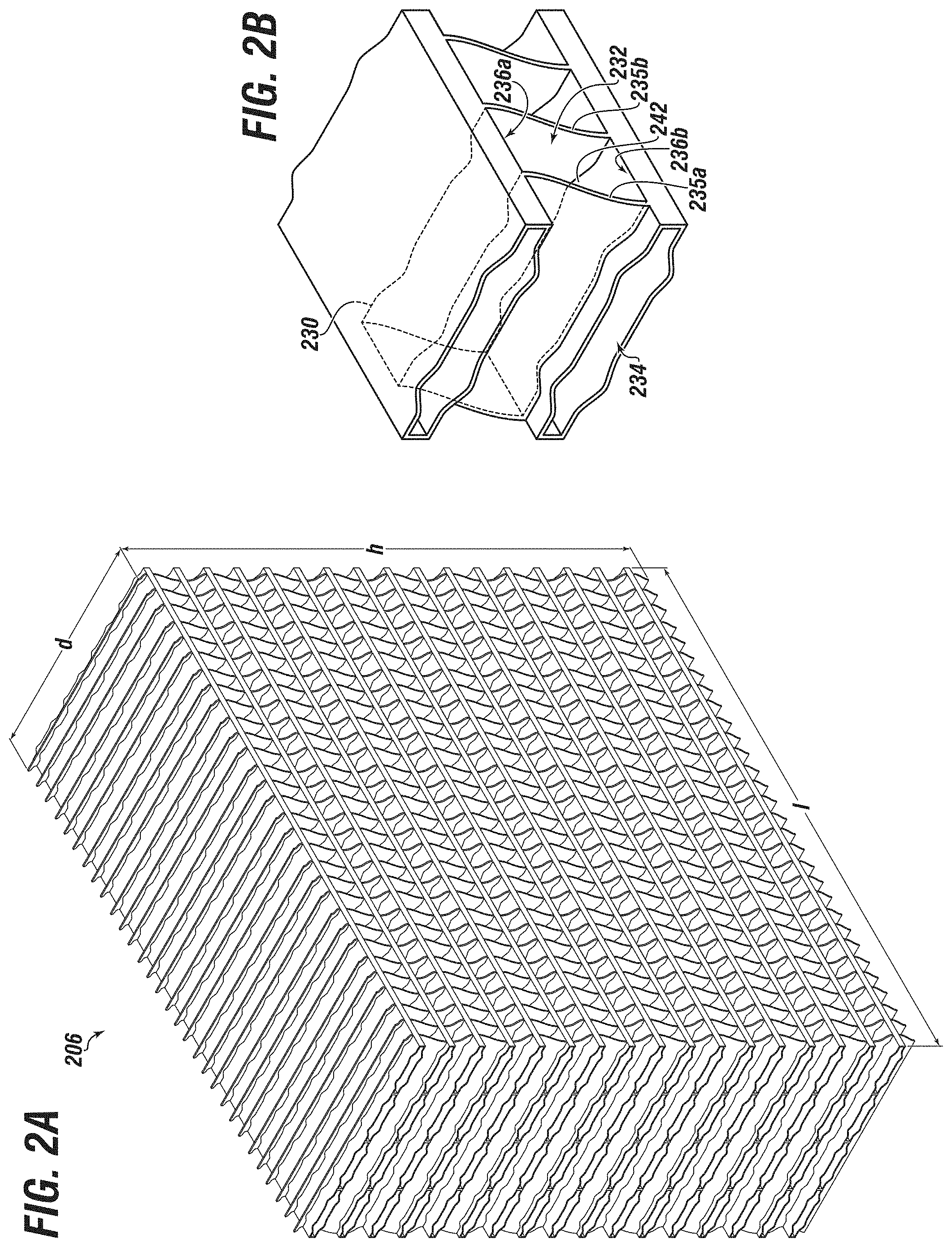


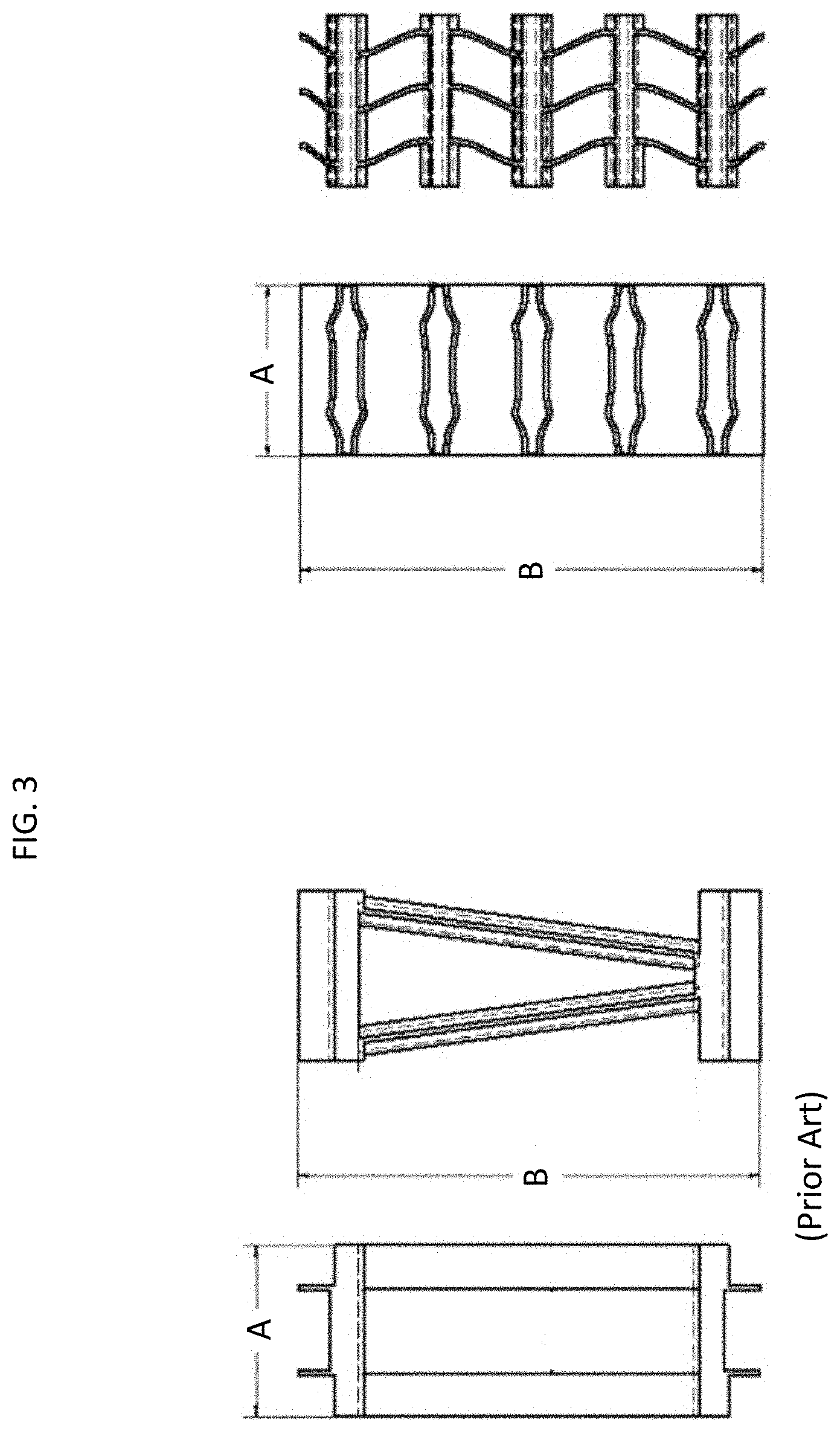
United States Patent
Application |
20200033062 |
Kind Code |
A1 |
Barney; Brian ; et
al. |
January 30, 2020 |
3D PRINTED RADIATOR CORE
Abstract
A 3D printed radiator core that includes a first fluid
passageway further having a first sidewall; a second sidewall; a
top platform; and a bottom platform. There is a second fluid
passageway proximate to, but fluidly isolated from, the first fluid
passageway. An at least part of the first fluid passageway is
defined in lateral cross-section by the top platform having an
upward extending ridge.
Inventors: |
Barney; Brian; (Peoria,
IL) ; Rajani; Hamid Reza Zareie; (Edmonton, CA)
; Yan; Haoxuan; (Edmonton, CA) |
|
Applicant: |
Name |
City |
State |
Country |
Type |
GLOBAL HEAT TRANSFER ULC |
Edmonton |
|
CA |
|
|
Family ID: |
67441712 |
Appl. No.: |
16/044937 |
Filed: |
July 25, 2018 |
Current U.S.
Class: |
1/1 |
Current CPC
Class: |
F28F 1/26 20130101; F28D
1/05383 20130101; F28D 2001/0266 20130101; F28F 1/08 20130101; F28D
1/05366 20130101; F28D 2001/028 20130101; F28F 1/24 20130101 |
International
Class: |
F28D 1/053 20060101
F28D001/053 |
Claims
1. A 3D printed radiator core comprising: a first fluid passageway
further comprising: a first sidewall; a second sidewall; a top
platform; and a bottom platform; a second fluid passageway
proximate to, but fluidly isolated from, the first fluid
passageway; wherein an at least part of the first fluid passageway
is defined in lateral cross-section by the top platform having an
upward extending curvilinear ridge, and by the bottom platform
having a downward extending curvilinear ridge.
2. The 3D printed radiator core of claim 1, wherein the upward
extending curvilinear ridge lies proximately above the downward
extending curvilinear ridge on a shared vertical axis.
3. The 3D printed radiator core of claim 2, wherein a crest of the
upward extending curvilinear ridge is separated from an another
crest of the downward extending curvilinear ridge by a ridge height
in the range of 0.25 mm to 3 mm.
4. The 3D printed radiator core of claim 3, wherein the top
platform comprises a second upward extending curvilinear ridge, and
the bottom platform comprises a second downward extending ridge,
and wherein the first sidewall and the second sidewall are
separated by a sidewall gap in a range of 5 mm to 9 mm.
5. The 3D printed radiator core of claim 4, wherein in the at least
part of the first fluid passageway in lateral cross-section
comprises a top half of the first fluid passageway substantially
symmetrical to a bottom half of the first fluid passageway.
6. The 3D printed radiator core of claim 4, wherein in the at least
part of the first fluid passageway in lateral cross-section
comprises a first side half of the first fluid passageway
substantially symmetrical to a second side half of the first fluid
passageway.
7. The 3D printed radiator core of claim 1, the second fluid
passageway further comprising: a first second fluid passageway
sidewall; and a second second fluid passageway sidewall, wherein
the second fluid passageway is transverse to the first fluid
passageway, and wherein an at least part of the second fluid
passageway is defined in lateral cross-section by the first second
fluid passageway sidewall having at least one curvature.
8. The 3D printed radiator core of claim 7, wherein the upward
extending curvilinear ridge extends into the second fluid
passageway.
9. The 3D printed radiator core of claim 1, the first fluid
passageway further comprising an entry and an exit, wherein the
entry and the exit are separated by a length in a range of 70
inches to about 90 inches, and wherein the upward extending
curvilinear ridge extends along the length.
10. A 3D printed radiator core comprising: a first fluid passageway
further comprising: a first sidewall; a second sidewall; a top
platform; and a bottom platform; a second fluid passageway
proximate and thermally conductive to, but fluidly isolated from,
the first fluid passageway; wherein an at least part of the first
fluid passageway is defined in lateral cross-section by the top
platform having an upward extending ridge, and by the bottom
platform having a downward extending ridge.
11. The 3D printed radiator core of claim 10, wherein the upward
extending ridge lies proximately above the downward extending ridge
on a shared vertical axis.
12. The 3D printed radiator core of claim 10, wherein an innermost
surface of a crest of the upward extending ridge is separated from
an another innermost surface of a crest of the downward extending
ridge by a ridge height in a range of 0.4 mm to 2.4 mm, and wherein
an amplitude of the crest with reference to the top platform is in
a range of 0.1 mm to 1.5 mm.
13. The 3D printed radiator core of claim 10, wherein the top
platform comprises a second upward extending ridge, and the bottom
platform comprises a second downward extending ridge, and wherein
the first sidewall and the second sidewall are separated by a
sidewall gap in a range of 5 mm to 9 mm, and wherein at least a
portion of an innermost surface of the top platform is separated
from an another portion of an innermost surface of the bottom
platform by a platform height in a range of 0.2 mm to 1 mm.
14. The 3D printed radiator core of claim 13, wherein the at least
part of the first fluid passageway in lateral cross-section
comprises a top half of the first fluid passageway substantially
reflectionally symmetrical to a bottom half of the first fluid
passageway.
15. The 3D printed radiator core of claim 10, wherein the at least
part of the first fluid passageway in lateral cross-section
comprises a first side half of the first fluid passageway
substantially reflectionally symmetrical to a second side half of
the first fluid passageway.
16. The 3D printed radiator core of claim 15, wherein an at least
part of the second fluid passageway in lateral cross-section
comprises a top half of the second fluid passageway substantially
rotationally symmetrical to a bottom half of the second fluid
passageway.
17. The 3D printed radiator core of claim 15, the second fluid
passageway further comprising: a first second fluid passageway
sidewall; and a second second fluid passageway sidewall, wherein
the second fluid passageway is transverse to the first fluid
passageway, and wherein an at least part of the second fluid
passageway is defined in lateral cross-section by the first second
fluid passageway sidewall having at least one curvature.
18. The 3D printed radiator core of claim 10, wherein the upward
extending ridge comprises a midpoint on a first vertical axis, and
a center of the first fluid passageway comprises a second midpoint
to a second vertical axis, wherein a ratio of a lateral distance to
the midpoint to a lateral distance to the second midpoint is in a
range of 0.45 to 0.55.
19. A 3D printed radiator core comprising: a first fluid passageway
further comprising: a first sidewall; a second sidewall; a top
platform; and a bottom platform; a second fluid passageway
proximate to, but fluidly isolated from, the first fluid
passageway; wherein an at least part of the first fluid passageway
in lateral cross-section comprises a first side half reflectionally
symmetrical to a second side half of the first fluid passageway,
wherein an at least part of the second fluid passageway in lateral
cross-section comprises a top half of the second fluid passageway
rotationally symmetrical to a bottom half of the second fluid
passageway, wherein the first sidewall and the second sidewall are
separated by a sidewall gap in a range of 5 mm to 9 mm, and wherein
an at least a portion of an innermost surface of the top platform
is separated from an another portion of an innermost surface of the
bottom platform by a platform height in a range of 0.2 mm to 1
mm.
20. The 3D printed radiator core of claim 19, wherein the at least
part of the first fluid passageway in lateral cross-section further
comprises the top platform having an upward extending ridge, and
the bottom platform having a downward extending ridge.
Description
INCORPORATION BY REFERENCE
[0001] The subject matter of co-pending U.S. non-provisional
application Ser. No. 15/705,024, filed Sep. 14, 2017, Ser. No.
15/629,563, filed Jun. 21, 2017, Ser. No. 15/591,076, filed May 9,
2017, and Ser. Nos. 15/477,097 and 15/477,100, each filed Apr. 2,
2017, is incorporated herein by reference in entirety for all
purposes. One or more of these applications may be referred to
herein as the "Applications".
STATEMENT REGARDING FEDERALLY SPONSORED RESEARCH OR DEVELOPMENT
[0002] Not Applicable.
BACKGROUND
Field of the Disclosure
[0003] This disclosure generally relates to a heat exchanger unit
with characteristics of improved: airflow, noise reduction, cooling
efficiency, and/or structural integrity. Other aspects relate to a
system for monitoring airflow through a heat exchanger unit, or
fouling related thereto. In particular embodiments, any part or
component for a heat exchanger may be 3D printed or made via an
additive manufacturing process.
Background of the Disclosure
[0004] Whether its refrigeration, hot showers, air conditioning,
and even computers and hand-held devices, the function of heating
and cooling is prevalent in today's residential, commercial, and
industrial settings. One area of relevance is the oil and gas
industry, including exploration, upstream, and downstream
operations where the ability to heat and/or cool is critical.
Upstream operations can include drilling, completion, and
production, whereas downstream operations can include refining and
other related hydrocarbon processing, all of which utilize a vast
amount of process equipment including that which provide heat
transfer. To be sure, the background of the disclosure and
embodiments described herein are relevant elsewhere, such as a
small heat exchanger for a computer.
[0005] For brevity, discussion herein is focused on the O&G
industry. Common settings in this context are nothing short of
challenging in the sense that in many instances operations and
processes (and related equipment) are exposed to environmental
conditions, such as extreme heat, cold, wind, and dust (including
natural amounts of particulate, as well as that caused by the
operation of equipment and vehicles).
[0006] It is routine to have (indeed, need) some type of heat
exchange ability in such settings. As set forth in U.S. Ser. No.
15/477,097, an example operation in an industrial setting may
include one or more frac pump units. Each unit is typically
operable with a pump and engine mounted or otherwise disposed
thereon, as well as a radiator (or analogously referred to as
cooler, heat exchanger, etc.). As mentioned before, equipment like
this must be rugged and durable in order to have long-term
operational capacity and effectiveness.
[0007] The radiator is configured for cooling one or more hot
service fluids associated with the equipment of the frac pump unit,
such as lube oil or jacket water. The radiator typically includes a
`core` of stacked fins, with one part of the core providing a flow
are for the service fluid(s), while another part of the core
provides a proximate, albeit separate, flow area for ambient air. A
fan is used to blow or pull air through the stacked fins, the air
being a low or moderate enough temperature to cool the service
fluid, which is then recirculated in a loop.
[0008] The stacked fins often have a configuration that is
tantamount to an extensive amount of small air passageways
proximate to (albeit separate from) service fluid passageways,
whereby the air and the service fluid can `exchange heat` via the
surface material of the stacked fins between the passageways (e.g.,
aluminum).
[0009] Over time airborne dirt in and other particulate in the air
will begin to deposit on the air intake side (and elsewhere),
resulting in a fouled radiator. Fouling can seriously deteriorate
the capacity of the surface of the fins to transfer heat under the
conditions for which they were designed. Among other problems, the
fouling layer has a low thermal conductivity which increases the
resistance to heat transfer and reduces the effectiveness of heat
exchangers. In addition, fouling reduces the cross-sectional area
in the passageways, which causes an increase in pressure drop
across a heat exchanger.
[0010] Conventional manufacturing processes routinely utilize
subtractive manufacturing, machining, and stamping. For example, a
first fin section is stamped out of a machined piece of metal, with
a plate then added on top, followed by the addition of a transverse
fin section, with another plate, and then a repetitive process of
stamping, adding, stamping, adding, etc., followed by a brazing
process.
[0011] As a result of cost for materials and fabrication, heat
exchanger related components (e.g., a radiator core) are often made
outside the U.S., such as in China. The aforementioned
manufacturing process is cumbersome and while automated to some
extent, requires extensive manual labor. However, the cost-savings
of reduced material and labor costs for making and manufacturing
outside the U.S. is not without downsides.
[0012] It has been well-documented that theft of intellectual
property in China costs hundreds of millions of dollars annually.
Fear of theft is an inherent, sometimes unrecognized barrier for
companies operating in countries like China to undertake
innovation. For large pieces of equipment, such as industrial heat
exchangers, cost-savings of overseas manufacture may be reduced by
higher logistical costs for shipping, especially when fuel prices
soar.
[0013] There is a need in the art to overcome deficiencies and
defects identified herein. There is a need in the part to make and
manufacture heat exchanger technology without having to disclose
valuable IP to countries where theft is rampant. There is a need in
the art to alleviate logistical costs associated with overseas
manufacture and transportation back to the U.S. There is a need in
the art for producing a cost-effective radiator core with
characteristics of improved thermal conductivity, heat transfer,
and/or pressure drop.
SUMMARY
[0014] Embodiments herein pertain to a monitored heat exchanger
system that may include a heat exchanger unit in operable
engagement with a heat generating device, with an at least one
service fluid being transferable therebetween, the heat exchanger
unit further having a frame. There may be at least one cooler
coupled with the frame, the at least one cooler having an airflow
side and a service fluid side.
[0015] Embodiments herein pertain to a 3D printed radiator core.
The printed core may include a first fluid passageway proximate to
a second fluid passageway. The first fluid passageway may be
fluidly isolated from the second fluid passageway. The first fluid
passageway may be thermally conductive to the second fluid
passageway.
[0016] The first fluid passageway may include: a first sidewall; a
second sidewall; a top platform; and a bottom platform.
[0017] An at least part of the first fluid passageway (as may be
defined in lateral cross-section) may include the top platform
having an upward extending ridge. An at least part of the first
fluid passageway may include the bottom platform having a downward
extending ridge. Any of the ridges may be linear, curved,
curvilinear, and so forth.
[0018] The upward extending ridge may lie or be positioned
proximately above the downward extending curvilinear on a shared
vertical axis.
[0019] A crest of the upward extending ridge may be separated from
another crest of the downward extending ridge by a ridge height in
the range of about 0.25 mm to about 3 mm. In embodiments, the range
may be about 1 mm to about 1.5 mm.
[0020] The top platform may include a second upward extending
ridge. The bottom platform may include a second downward extending
ridge. The first sidewall and the second sidewall may be separated
by a sidewall gap in a range of about 5 mm to about 9 mm. The range
may be about 6 mm to about 8 mm.
[0021] The at least part of the first fluid passageway may (in
lateral cross-section) include a top half of the first fluid
passageway symmetrical or substantially symmetrical to a bottom
half of the first fluid passageway.
[0022] The at least part of the first fluid passageway may (in
lateral cross-section) include a first side half of the first fluid
passageway symmetrical or substantially symmetrical to a second
side half of the first fluid passageway.
[0023] In aspects, the second fluid passageway may further include
a first second fluid passageway sidewall; and a second second fluid
passageway sidewall. An at least part of the second fluid
passageway may be defined in lateral cross-section by the first
second fluid passageway sidewall having at least one curvature.
[0024] The second fluid passageway may be transverse to the first
fluid passageway. The upward extending ridge may extend into the
second fluid passageway.
[0025] The first fluid passageway may include an entry and an exit.
The entry and the exit may be separated by a length in a range of
70 inches to about 90 inches. The upward extending ridge may extend
along the entire length.
[0026] Other embodiments of the disclosure pertain to a 3D printed
radiator core that may include a first fluid passageway and a
second fluid passageway. The passageways may be proximate and
thermally conductive to each other. The passageways may be fluidly
isolated from each other.
[0027] Either of the first fluid passageway or the second fluid
passageway may include its own respective: a first sidewall; a
second sidewall; a top platform; and a bottom platform. One or more
of the sidewalls or platforms may be shared by the passageways.
[0028] An at least part of the first fluid passageway may be
defined (in lateral cross-section) by the top platform having an
upward extending ridge, and by the bottom platform having a
downward extending ridge. In aspects, the upward extending ridge
may lie or be positioned proximately above the downward extending
ridge on a shared vertical axis.
[0029] An innermost surface of a crest of the upward extending
ridge may be separated from another innermost surface of a crest of
the downward extending ridge by a ridge height in a range of about
0.4 mm to about 2.4 mm. In embodiments, the range may be about 1 mm
to about 1.5 mm.
[0030] An amplitude of the crest with reference to the top platform
may be in a range of about 0.1 mm to about 1.5 mm. In embodiments
the range may be about 0.3 mm to about 0.5 mm.
[0031] The top platform may include a second upward extending
ridge, and the bottom platform comprises a second downward
extending ridge. The first sidewall and the second sidewall are
separated by a sidewall gap in a range of about 5 mm to about 9 mm.
An at least a portion of an innermost surface of the top platform
is separated from another portion of an innermost surface of the
bottom platform by a platform height in a range of about 0.2 mm to
about 0.6 mm.
[0032] In aspects, the at least part of the first fluid passageway
in lateral cross-section may include a top half of the first fluid
passageway substantially reflectionally symmetrical to a bottom
half of the first fluid passageway. In other aspects, the at least
part of the first fluid passageway in lateral cross-section may
include a first side half of the first fluid passageway
substantially reflectionally symmetrical to a second side half of
the first fluid passageway. In yet other aspects, an at least part
of the second fluid passageway in lateral cross-section may include
a top half of the second fluid passageway substantially
rotationally symmetrical to a bottom half of the second fluid
passageway.
[0033] The second fluid passageway may include a first second fluid
passageway sidewall; and a second second fluid passageway sidewall.
The second fluid passageway may be generally transverse to the
first fluid passageway. An at least part of the second fluid
passageway may be defined in lateral cross-section by the first
second fluid passageway sidewall having at least one curvature.
[0034] The upward extending ridge may include a midpoint on a first
vertical axis, and a center of the first fluid passageway comprises
a second midpoint to a second vertical axis. A ratio of a lateral
distance to the midpoint to a lateral distance to the second
midpoint may be in a range of about 0.45 to about 0.55.
[0035] Still other embodiments of the disclosure pertain to a 3D
printed radiator core that may include a first fluid passageway
having a first sidewall; a second sidewall; a top platform; and a
bottom platform. There may be a second fluid passageway proximate
to the first fluid passageway.
[0036] An at least part of the first fluid passageway may include a
first side half reflectionally symmetrical or substantially
symmetrical to a second side half of the first fluid
passageway.
[0037] An at least part of the second fluid passageway may include
a top half of the second fluid passageway rotationally symmetrical
or substantially symmetrical to a bottom half of the second fluid
passageway.
[0038] The first sidewall and the second sidewall may be separated
by a sidewall gap in a range of about 5 mm to 9 about mm. In
aspects, the range may be about 6 mm to about 8 mm.
[0039] An at least a portion of an innermost surface of the top
platform may be separated from another portion of an innermost
surface of the bottom platform by a platform height in a range of
about 0.2 mm to about 1 mm.
[0040] The at least part of the first fluid passageway may include
the top platform having an upward extending ridge, and the bottom
platform having a downward extending ridge.
[0041] Other embodiments herein pertain to a 3D printing or
additive manufacturing system operable to produce a repeatable
lattice structure comprising a plurality of fluid passageways. The
structure may include a first set of fluid passages oriented in a
first direction, and a second set of fluid passages oriented in a
second direction. Either the first set or second set may include a
fluid passageway in accordance with embodiments herein.
[0042] Still other embodiments pertain a method of using 3D
printing or additive manufacturing to produce a repeatable lattice
structure comprising a plurality of fluid passageways. The
structure may include a first set of fluid passages oriented in a
first direction, and a second set of fluid passages oriented in a
second direction. Either the first set or second set may include a
fluid passageway in accordance with embodiments herein.
[0043] The lattice structure may be a radiator core. The radiator
core may be useful with a heat exchanger configured to cool a
liquid with air.
[0044] These and other embodiments, features and advantages will be
apparent in the following detailed description and drawings.
BRIEF DESCRIPTION OF THE DRAWINGS
[0045] A full understanding of embodiments disclosed herein is
obtained from the detailed description of the disclosure presented
herein below, and the accompanying drawings, which are given by way
of illustration only and are not intended to be limitative of the
present embodiments, and wherein:
[0046] FIG. 1 shows an overview diagram of a 3D printing system
according to embodiments of the disclosure;
[0047] FIG. 2A shows an isometric view of a 3D printed radiator
core according to embodiments of the disclosure;
[0048] FIG. 2B shows a zoom-in sectional view of a partial cell
structure of the core of FIG. 2A according to embodiments of the
disclosure;
[0049] FIG. 2C shows a sideview of a passageway of a single cell
module of the core of FIG. 2A according to embodiments of the
disclosure;
[0050] FIG. 2D shows a rotated side view of the passageway of FIG.
2C according to embodiments of the disclosure;
[0051] FIG. 2E shows, a side cross-sectional view of the passageway
of FIG. 2D according to embodiments of the disclosure;
[0052] FIG. 2F shows, a side cross-sectional view of the passageway
of FIG. 2D with fluid motion therethrough according to embodiments
of the disclosure;
[0053] FIG. 2G shows a sideview of an adjacent passageway to the
passageway of FIG. 2C according to embodiments of the disclosure;
and
[0054] FIG. 3 shows a side view of a partial conventional cooler
structure compared to a partial 3D printed cooler of the same size
according to embodiments of the disclosure.
DETAILED DESCRIPTION
[0055] Herein disclosed are novel apparatuses, systems, and methods
that pertain to an improved heat exchanger and aspects (including
components) related thereto, details of which are described
herein.
[0056] Embodiments of the present disclosure are described in
detail with reference to the accompanying Figures. In the following
discussion and in the claims, the terms "including" and
"comprising" are used in an open-ended fashion, such as to mean,
for example, "including, but not limited to . . . ". While the
disclosure may be described with reference to relevant apparatuses,
systems, and methods, it should be understood that the disclosure
is not limited to the specific embodiments shown or described.
Rather, one skilled in the art will appreciate that a variety of
configurations may be implemented in accordance with embodiments
herein.
[0057] Although not necessary, like elements in the various figures
may be denoted by like reference numerals for consistency and ease
of understanding. Numerous specific details are set forth in order
to provide a more thorough understanding of the disclosure;
however, it will be apparent to one of ordinary skill in the art
that the embodiments disclosed herein may be practiced without
these specific details. In other instances, well-known features
have not been described in detail to avoid unnecessarily
complicating the description. Directional terms, such as "above,"
"below," "upper," "lower," "front," "back," "right", "left",
"down", etc., are used for convenience and to refer to general
direction and/or orientation, and are only intended for
illustrative purposes only, and not to limit the disclosure.
[0058] Connection(s), couplings, or other forms of contact between
parts, components, and so forth may include conventional items,
such as lubricant, additional sealing materials, such as a gasket
between flanges, PTFE between threads, and the like. The make and
manufacture of any particular component, subcomponent, etc., may be
as would be apparent to one of skill in the art, such as molding,
forming, press extrusion, machining, or additive manufacturing.
Embodiments of the disclosure provide for one or more components to
be new, used, and/or retrofitted to existing machines and
systems.
[0059] Various equipment may be in fluid communication directly or
indirectly with other equipment. Fluid communication may occur via
one or more transfer lines and respective connectors, couplings,
valving, and so forth. Fluid movers, such as pumps, may be utilized
as would be apparent to one of skill in the art.
[0060] Numerical ranges in this disclosure may be approximate, and
thus may include values outside of the range unless otherwise
indicated. Numerical ranges include all values from and including
the expressed lower and the upper values, in increments of smaller
units. As an example, if a compositional, physical or other
property, such as, for example, molecular weight, viscosity, melt
index, etc., is from 100 to 1,000, it is intended that all
individual values, such as 100, 101, 102, etc., and sub ranges,
such as 100 to 144, 155 to 170, 197 to 200, etc., are expressly
enumerated. It is intended that decimals or fractions thereof be
included. For ranges containing values which are less than one or
containing fractional numbers greater than one (e.g., 1.1, 1.5,
etc.), smaller units may be considered to be 0.0001, 0.001, 0.01,
0.1, etc. as appropriate. These are only examples of what is
specifically intended, and all possible combinations of numerical
values between the lowest value and the highest value enumerated,
are to be considered to be expressly stated in this disclosure.
[0061] Embodiments herein may be described at the macro level,
especially from an ornamental or visual appearance. Thus, a
dimension, such as length, may be described as having a certain
numerical unit, albeit with or without attribution of a particular
significant figure. One of skill in the art would appreciate that
the dimension of "2 centimeters" may not be exactly 2 centimeters,
and that at the micro-level may deviate. Similarly, reference to a
"uniform" dimension, such as thickness, need not refer to
completely, exactly uniform. Thus, a uniform or equal thickness of
"1 millimeter" may have discernable variation at the micro-level
within a certain tolerance (e.g., 0.001 millimeter) related to
imprecision in measuring and fabrication.
Terms
[0062] The term "connected" as used herein may refer to a
connection between a respective component (or subcomponent) and
another component (or another subcomponent), which can be fixed,
movable, direct, indirect, and analogous to engaged, coupled,
disposed, etc., and can be by screw, nut/bolt, weld, and so forth.
Any use of any form of the terms "connect", "engage", "couple",
"attach", "mount", etc. or any other term describing an interaction
between elements is not meant to limit the interaction to direct
interaction between the elements and may also include indirect
interaction between the elements described.
[0063] The term "fluid" as used herein may refer to a liquid, gas,
slurry, multi-phase, etc. and is not limited to any particular type
of fluid such as hydrocarbons.
[0064] The term "radiator" as used herein can refer to or
interchangeable with the term `heat exchanger` or `heat exchanger
panel`. The radiator can be a heat exchanger used to transfer
thermal energy from one medium to another for the purpose of
cooling and/or heating.
[0065] The term "cooler" as used herein can refer to a radiator
made up of tubes or other structure surrounded by fins (or `core`)
that can be configured to extract heat from a fluid moved through
the cooler. The term can be interchangeable with `heat exchanger
panel` or comparable. Heat can also be exchanged to another fluid,
such as air.
[0066] The term "cooling circuit" as used herein can refer to a
cooler and respective components.
[0067] The term "core" as used herein can refer to part of a
cooler, and can include multiple layers of fins, fin elements, a
lattice structure, or a plurality of interconnected cell
modules.
[0068] The term "heat exchanger unit" as used herein can refer to a
device or configuration that uses one or more coolers along with
other components, such as a fan, mounts, tubing, frame, and so on.
The heat exchanger unit can be independent and standalone or can be
directly mounted to a heat generating device. The heat exchanger
unit can be operable to pull (draw) ambient air in through the
coolers in order to cool one or more service fluids. The heated air
is moved or blown out as a waste exhaust stream. Or vice versa.
[0069] The term "heat generating device" (or sometimes `HGD`) as
used herein can refer to an operable device, machine, etc. that
emits or otherwise generates heat during its operation, such as an
engine, motor, a genset, or a frac pump (including the pump and/or
respective engine). The HGD can be for an industrial or a
residential setting.
[0070] The term "genset" (or generator set) as used herein can
refer to a `diesel generator` or the combination of a diesel engine
(or comparable) and an electric generator. The genset can convert
mechanical energy to electrical energy.
[0071] The term "utility fluid" as used herein can refer to a fluid
used in connection with the operation of a heat generating device,
such as a lubricant or water. The utility fluid can be for heating,
cooling, lubricating, or other type of utility. `Utility fluid` can
also be referred to and interchangeable with `service fluid` or
comparable.
[0072] The term "mounted" as used herein can refer to a connection
between a respective component (or subcomponent) and another
component (or another subcomponent), which can be fixed, movable,
direct, indirect, and analogous to engaged, coupled, disposed,
etc., and can be by screw, nut/bolt, weld, and so forth.
[0073] Referring now to FIG. 1, an overview diagram of a 3D
printing system in accordance with embodiments disclosed herein, is
shown.
[0074] 3D printing (or additive manufacturing) system 100 may
include a computer aided design (CAD) module 102 in operable
communication with a 3D printing module 104. Operable communication
may be via respective cabling, wireless, and so forth. Thus, a
transceiver or the like may be used transmit data and instructions
between the CAD module 102 and the 3D printing module 104.
Alternatively, a wired or integrated setup may be used. Although
not shown here, one of skill would appreciate system 100 may
include various utility connections, such as power, cooling,
ventilation, and so forth.
[0075] The CAD module 102 may be used for designing and generating
a computer model of a part 106. Although not meant to be limited,
embodiments herein provide for the part 106 to be a core (i.e.,
radiator core) for a heat exchanger. In this respect, the part 106
may have a repeatable cell structure of predetermined dimensioning,
the size and repetitive nature being unachievable by regular CNC
machining process and the like. The printed part 106 may be
designed and sized for use as may be desired. For example, a
radiator core may be designed and sized for fitting within a
computer or mobile device. Alternatively, the radiator core may be
designed and size for fitting in a heat exchanger unit for a frac
skid (such as for a cooling a motor for a frac pump). The heat
exchanger unit may be like that as provided for in the
Applications, each incorporated herein in in its entirety for all
purposes.
[0076] The CAD module 102 may include various subcomponents known
to one of skill, such as computer 122 with a processor, a memory, a
transceiver, a plurality of inputs and outputs, a display etc. The
CAD module 102 may be integral with or separate from the 3D
printing module 104.
[0077] The processor may be suitable (including respective computer
instructions) for creating a computer model of the part 106
according to inputs and data received from a user. The processor
may include a circuit board, memory, display, inputs, and/or other
electronic components such as a transceiver or external connection
for communicating with external computers and the like.
[0078] The processor may implement embodiments herein with one or
more computer programs stored in or on computer-readable medium
residing on or accessible by the processor. A computer program can
be embodied in any suitable computer-readable medium for use by or
in connection with an instruction execution system, apparatus, or
device, such as a computer-based system, processor-containing
system, or other system that can fetch the instructions from the
instruction execution system, apparatus, or device, and execute the
instructions, as would be known to one of skill.
[0079] The memory may be any computer-readable non-transitory
medium that can store the program for use by or in connection with
the instruction execution system, apparatus, or device. The
computer-readable medium can be, for example, but not limited to,
an electronic, magnetic, optical, electro-magnetic, infrared, or
semi-conductor system, apparatus, or device. More specific,
although not inclusive, examples of the computer-readable medium
would include the following: an electrical connection having one or
more wires, a portable computer diskette, a random access memory
(RAM), a read-only memory (ROM), an erasable, programmable,
read-only memory (EPROM or Flash memory), an optical fiber, and a
portable compact disk read-only memory (CDROM).
[0080] The inputs may allow a user to design and modify a model of
the part 106 and may comprise a keyboard 118, a mouse or touchpad
124, trackball, a touchscreen 116, buttons, dials, virtual inputs,
and/or a virtual reality simulator. The inputs may also be used to
control or instruct the 3D printing module 104. The computer 122
may be a desktop, laptop, or other suitable programmable device.
The computer 122 may be configured with a synchronization unit 120
for interfacing with another computer or mobile device 114.
[0081] The printing module 104 may be any such module suitable to
print the part 106. The module 104 may include a frame 108, a
support surface 110, a material reserve, a feeder, a material
applicator, a set of motors, a processor, and so forth. The 3D
printing module 106 may include a powder coating system.
[0082] The frame 108 may provide the structure for the support
surface 110, and other respective components. The frame 108 may
include a base, horizontal member, vertical members, cross members,
and mounting points for mounting the above components thereto.
Alternatively, the frame 108 may be a walled housing or similar
structure.
[0083] The support surface 110 may be configured to support the
part 106 as it is being constructed and may be a stationary or
movable flat tray or bed, a substrate, a mandrel, a wheel,
scaffolding, or similar support. The support surface 110 may be
integral with the 3D printing module 104 or may be removable and
transferable with the part 106 as it is being constructed.
[0084] The material reserve may be suitable to hold 3D printing
material, and may be in a known form such as a hopper, tank,
cartridge, container, spool, or other similar material holder. The
material reserve may be integral with the 3D printing module 104 or
may be disposable and/or reusable.
[0085] Material suitable for embodiments herein may be for 3D
printing techniques, such as Selective Laser Sintering (SLS),
Direct Metal Laser Sintering (DMLS), Selective Laser Melting (SLM),
and Fused Deposition Modeling (FDM). Thus, the part 106 may be made
from metal or from other materials, such as plastics (including
thermoplastics), composites, metal-infused plastics, or any other
material suitable for 3D printing.
[0086] For example, the additive manufacturing material may be
acrylonitrile butadiene styrene (ABS), Polyphenylene Sulphide
(PPS), Polysulfone (PSU), Polytetrafluoroethylene (PTFE),
Fluorinated Ethylene Propylene (FEP), Ethylene tetrafluoroethylene
(ETFE), Polyetherimide (PEI), Polyethetersulfone (PES),
PolyEtherEther-Ketone (PEEK), non-PEEK (including PAEK, PEK,
PEKEEK, PEKK, and PFA), polyamidic (e.g., Polyamide 6--TA6'),
Polycarbonate (PC), Poly(methyl methacrylate) (PMMA), straw-based
plastic, or other similar material. The manufacturing material may
originally be in pellet or powder form, filament or spooled form,
or any other suitable form. A feeder may direct the additive
manufacturing material to the applicator 112. The feeder may be in
the form of a spool feeder, gravity feed, a pump, an auger, or any
other suitable feeder.
[0087] Filler materials include additives such as milled carbon
fiber, copper powder, copper fiber, and graphene. Such an additive
may be used with a thermoplastic material to give the resultant 3D
printed part better thermal conductivity properties.
[0088] In order to achieve beneficial weight reduction embodiments
herein provide for new materials, including polymer-based
composites, in manufacturing of heat exchanger components. In terms
of cost and performance, these new materials are able to compete
with metal-based materials such as aluminum and copper alloys.
Specifically, these new and light-weight materials may be able to
withstand the maximum fluid pressure requirements at operating
temperatures as high as 250.degree. F. Also, these composites may
be thermally conductive enough to preserve the thermal efficiency.
In addition, the new materials may yet further be compatible with
3D printing technology.
[0089] Research has shown that the more metallic additive used with
plastic, the better the thermal conductivity; however, glass
transition temperature may be detrimentally affected. Comprehensive
research was thus carried out to identify and test potential
thermoplastics that may be used in developing the new composite
materials. Glass transition temperature as an indicator of
mechanical strength, water absorption, thermal conductivity, and
printability constitute key criteria considered and tested. Results
showed that only a few categories of thermoplastics are strong
enough to operate at high temperatures while showing a good
hydrolysis resistant. ABS, PEEK, and PPSU are example
thermoplastics that successfully passed mechanical and hydrolysis
tests. These three thermoplastics were also used in a 3D printer
and the results were satisfactory. However, the thermal
conductivity of these plastics was viewed as too low and therefore
using them in heat exchange applications may result in
significantly reduced the thermal efficiency.
[0090] Thus, metal additives or fillers were considered for
addition to polymer-based composites in order to achieve higher
thermal conductivities. Acrylonitrile Butadiene Styrene (ABS) was
chosen as an example matrix for the new composite. Two different
types of metallic additives, namely copper powder and chopped
copper fiber were tested as a reinforcing phase. The size of the
copper powder varied from about 10 micrometers to about 300
micrometers in average bulk diameter. The copper fiber had an
average bulk diameter of about 20 micrometers to about 60
micrometers, with an average length that varied from about 50
micrometers to 400 micrometers. ABS was mixed alternatively with
these additives in various ratios through a single screw
extruder.
[0091] Extrusion occurred at a temperature range of 180.degree. C.
to 210.degree. C., extruding a 3 mm filament suitable for use in 3D
printer in accordance with embodiments herein. In addition to
testing the thermal conductivity of the composite filaments,
testing was also conducted to ensure that the mechanical strength
and the integrity of the new composites remain in the acceptable
range.
[0092] The results indicate that adding metallic additives, such as
copper, improves the thermal conductivity of ABS. The change in
thermal conductivity was found to be more significant in copper
fiber infused filaments rather than copper powder infused
filaments. Microstructural analysis shows that copper fibers may
create many continuous paths through the matrix (percolation
phenomenon) that may carry heat, whereas while copper powders may
create discontinuous paths that do not have a significant impact on
the capability of matrix to conduct heat.
[0093] Although adding copper additives improves the thermal
conductivity of ABS, the glass transition temperature and therefore
the mechanical strength of the composites drop as the amount of
copper additives increases. Testing filaments with various ratios
of copper fiber and ABS indicates that the maximum acceptable ratio
is about 40% copper fiber. Infusing higher amounts of copper fiber
may reduce the mechanical strength of the composites below the
acceptable ranges of anticipated operating conditions. Embodiments
herein provide for a base matrix material infused with a metallic
additive, the resultant filament material having about 10% to about
50% by weight of the metallic additive.
[0094] A difficulty in the manufacturing of copper infused
filaments was also addressed. Copper infused filaments that come
out of the extruder may be too brittle to be directly fed into the
3D printer. These filaments may easily break under the pressure of
the driving system in a 3D printer. It was discovered that this
issue is mainly induced by nonuniform distribution of copper
additives within the matrix as areas with higher densities of
copper fiber are prone to cracking.
[0095] It was discovered that chopping the infused filament into
pellets may be beneficial. That is, the copper infused filament may
be chopped into pellets after a first extrusion. The copper infused
pellets were then fed back into the extruder. The results indicated
that subsequent, or multiple, extrusion(s) leads to more uniform
distribution of the second phase in the filaments and therefore
improves the flexibility of the copper infused filaments. About 1
to 4 rounds of extrusion may yield an optimum ductility.
[0096] Thus, embodiments herein provide for forming a first infused
filament. Chopping or cutting the first infused filament into
pellets. Extruding the first infused filament pellets, and
repeating as may be desired, to form a 3D printing material for use
in system 100.
[0097] In operation, the material applicator 112 may deposit a
selected 3D printing material onto the support surface 110 (and/or
on previously constructed layers of the part 106). The material
applicator 112 may include a nozzle, guide, sprayer, or other
similar component for channeling the additive manufacturing
material and a laser, heater, or similar component for melting the
additive manufacturing material and bonding (e.g., sintering) the
additive manufacturing material onto a previously constructed
layer. The material applicator 112 may be sized according to the
size of the pellets, powder, or filament being deposited.
[0098] Motors may be used for positioning the material applicator
112 over the support surface 110, and thereby moving during the
deposit application. Motors may be configured in such a manner
whereby the material applicator 112 may be moved in a lateral "x"
direction, a longitudinal "y" direction, and an altitudinal "z"
direction. In aspects, motors may alternatively move the support
surface 110 (and hence the part 106) while the material applicator
112 may be stationary.
[0099] One of skill would appreciate the 3D printing system 100 may
be any type of additive manufacturing or "3D printing" system such
as a sintering, laser melting, laser sintering, extruding, fusing,
stereolithography, extrusion, light polymerizing, powder bed, wire
additive, or laminated object manufacturing system. The 3D system
100 may also be a hybrid system that combines 3D printing with
machining, molding, scaffolding, and/or other subtractive
manufacturing or assembly techniques.
[0100] Referring now to FIGS. 2A, 2B, 2C, 2D, 2E, and 2F together,
an isometric view of a 3D printed radiator core, and a zoom-in
sectional view of a partial cell structure of the core of FIG. 2A,
a sideview of a passageway of a single cell module of the core of
FIG. 2A, a rotated side view of the passageway of FIG. 2C, a side
cross-sectional view of the passageway of FIG. 2D, a
cross-sectional view of the passageway of FIG. 2D with fluid motion
therethrough, and a lateral cross-sectional view of an adjacent
passageway, respectively, in accordance with embodiments disclosed
herein, are shown.
[0101] Although 3D printing has been around for decades, related
printing processes have not been of great consideration for
industrialized heat exchange technology. FIG. 2A shows a radiator
core 206 that may be 3D printed (such as via system 100), including
with any material described herein. Although not limited to any
particular overall shape, FIG. 2A illustrates the core 206 may be
generally rectangular prism in nature. Thus, the core 206 may have
a size (or volume) characterized by a length l, a height h, and a
depth d. In a non-limiting example, length l may be in the range of
about 70'' to about 90''; height h may be in the range of about
55'' to about 75''; depth d may be in the range of about 4'' to
about 8''.
[0102] The core 206 may be 3D printed with varied structure. As
shown, the core 206 may have a lattice structure with multiple,
repeatable cell module structure(s) 230. Although a myriad of ways
exist to characterize the structure of the core 206, one such
manner is to refer to the cell structure 230. Much like an organic
`cell`, the cell structure 230 may be contemplated as the basic
structure and building block of the core 206. In this instance, the
cell structure 230 may be referred to as the interaction or
interface between adjacent, yet isolated fluid passageways 232, 234
(i.e., fluid within passageway 232 may be kept from being in fluid
communication with fluid in passageway 234). In aspects, the first
fluid passageway 232 may accommodate a gaseous fluid, such as air
(or airflow), while the second fluid passageway 234 may accommodate
a liquidious fluid, such as water or a water-based (aqueous)
mixture.
[0103] The single cell module (or `module`) 230 may include the
first fluid passageway 232 that may be defined by the arrangement
of a first sidewall 235a, and a second sidewall 235b. The first
sidewall 235a may be coupled (in this instance, indirectly) with
the second sidewall 235b via a top deck 236a and a bottom deck
236b. The top deck 236a may correspond to a bottom of fluid
passageway 234a. In a similar manner, the bottom deck 236b may
correspond to a top of fluid passageway 234b. The outermost
surfaces of the top and bottom decks 236 a, b through the
passageway by be linear in lateral cross-section, whereas the
outermost surfaces of the sidewalls 235 a, b may be curvilinear in
lateral cross-section.
[0104] The first sidewall 235a and the second sidewall 235b may
have a respective thickness T2. Although the thickness T2 of any
particular sidewall (of core 206) may be equal or uniform at the
visual level, there may be variation within a certain tolerance.
Just the same, the thickness T2 need not be equal, and thus may
indeed be varied. Thickness T2 may be in the range of about 0.1 mm
to about 0.5 mm.
[0105] The top deck 236a and the bottom deck 236b may have a
respective thickness T1. Although the thickness T1 of any
particular top or bottom deck (of core 206) may be equal or uniform
at the visual level, there may be variation within a certain
tolerance. Just the same, the thickness T1 need not be equal, and
thus indeed may be varied. Thickness T1 may be in the range of
about 0.1 mm to about 0.5 mm.
[0106] Thickness T1 and/or T2 may have a direct impact on overall
size of the core 206, as well as heat transfer characteristics. Too
thin of material thickness may result in fragility or be
non-printable; too thick of material may decrease heat
transferability or increase costs.
[0107] The outermost surfaces of the top deck 236a and the bottom
deck 236b may be separated by spacing of a height h1. The height
between any two points may vary along a length of the passageway
232. Although the first sidewall 235a and the second sidewall 235b
may have a relative height equal to that of the height h1, FIG. 2D
illustrates a curvilinear nature of the each of the sidewalls 235
a, b whereby if the sidewalls were flattened (straightened out),
the respective height would be larger than that of the height h1.
FIG. 2D further illustrates midpoint reference 238 may divide a
respective sidewall to the point that the reflection of an upper
half need not mirror the lower half. On the other hand, were one of
the sidewalls 235 a, b rotated 180.degree. around a pivot point P1,
it may have the same appearance, and thus symmetry. Still,
sidewalls of any cell module of the core 206 need not be
curvilinear nor uniform, and may have variation in height.
[0108] The sidewalls 235 a, b may have a dual curvature
characteristic represented by respective radius of curvatures r1,
r2. In aspects, r1 may be equal to r2 within a given tolerance. The
equal and opposite appearance of r1 and r2 provides a `wavy` or
sinusoidal orientation of sidewalls 235 a, b. Although the
sidewalls of any module of the core 206 may have varied shapes (in
cross-section), including linear, the repetitive nature of a
sinusoidal shape may provide desired synergy of improved pressure
drop and improved heat transfer characteristics for fluid passages
232 of the core 206. The curvature of r1 and/or r2 may be in the
range of about 2.degree..
[0109] In aspects, the curvilinear nature of the sidewalls 235 a, b
may provide additional surface area for heat transfer for module
230, as compared to lesser surface area with linear sidewalls of a
comparable height.
[0110] The sidewalls 235 a, b may be separated by a spacing length
l1. Although it need not be, the lateral spacing between any given
pair of lateral cross-sectional points between respective sidewalls
may be equal. Thus, as shown here l1 may be equal to l1a. l1 may be
in the range of about 1 mm to about 5 mm.
[0111] Either or both of the top deck 236a and the bottom deck 236b
may have one or more ridge or fin-shape surfaces. In aspects,
module 230 may have at least one ridge surface 242 associated
therewith. Although not limited, passageway 232 may have between
about 0 to about 6 ridges associated therewith. FIG. 2C illustrates
a plurality of four ridges 242 with 242 a, b, c. In contrast to
conventional radiator cores, 3D printed core (206) has the unique
characteristic of having shared surface interaction between fluid
passageways 232 and 234. That is, passageways for conventionally
manufactured cores are not only fluidly isolated, but also
structurally isolated, typically by the presence of one or more
plates between adjacent passageways (i.e., fin layers).
[0112] FIGS. 2E-2F exemplify a given flow passage through a
respective cell module may have one or more flow regimes. The cell
module 230 may have a generally uniform flow regime, which may be
achievable by having continuous, including linear, and/or planar
horizontal/vertical, uniform surfaces. This type of flow regime may
be desirous in certain applications, and may be characterized as
having a low pressure drop. However, in other applications it may
be desirous to have discontinuous surfaces.
[0113] It has been discovered that the parameters related to the
geometry of the cell module 230 directly relates to thermal
conductivity and pressure drop performance. One of skill in the art
would greatly appreciate that modifying structure of the passageway
232 may have a significant impact on the heat transferability
and/or pressure drop of the other passageway 234, and vice versa.
This is particularly the case when the mediums are different, such
as gaseous and liquidious.
[0114] Thus, in contrast to conventional cores, where fin isolation
provides for independent fin design, with 3D printing, it is of
significance that there is dependence between adjacent passageways
232, 234.
[0115] FIG. 2E illustrates a non-limiting example of various
discontinuities on the top deck 236a and the bottom deck 236b that
effect not only fluid flowing through passageway 232, but also
adjacent passageways 234 a and b.
[0116] In this example, fluid may enter passageway 232 at position
F1 (tantamount to an inlet of the cell module 230) with the least
amount of resistance or obstruction (see height h1). The fluid may
come from the inlet of the heat exchanger (such as from a tank) or
from a preceding cell module. As the fluid moves with appreciable
velocity to position F2, the fluid may eddy as a result of the
narrowing space (see height h2) formed by an at least one ride
portion 242. As shown in the Figures together, there may be an
upper ridge portion 242b on the top deck 236a and a lower ridge
portion 242a on the bottom deck 236b (see FIG. 2C). Narrowing at
point F2 may be reduced spacing between at least one ridge and
opposite deck surface. In aspects, narrowing at point F2 may be
spacing between two respective ridges.
[0117] Accordingly a midpoint of a crest of one ridge (e.g., 242a)
may be on the same vertical axis (e.g., an axis parallel to
reference line 244) as a midpoint of a crest of another ridge
(e.g., 242b). The distance between the underside of each ridge
(e.g., h6) may be in a range of about 0.25 mm to about 3 mm.
[0118] Similarly, the top most point of a crest of one ridge (e.g.,
242a) may additionally or alternatively be on the same horizontal
axis (e.g., an axis parallel to reference line 238) as the top most
point of another crest of another ridge (e.g., 242). A distance
from top most midpoint to another top most midpoint on a horizontal
may be in the range of about 0.5 mm to about 7 mm.
[0119] Eddies may desirably provide a suitable amount of
instability and stirring within the flow regime of the cell module
230 whereby heat transfer is improved. However, eddies may
undesirably attribute to increases in pressure drop. The ridge
portion or structure of the cell module is not meant to be limited.
The cell module 230 may have varied ridge portions on a respective
top deck and/or bottom deck. Moreover, the ridge portion need not
be uniform across the lateral length l1 of the passageway 232. Even
furthermore, cell modules of an entire core may have varied ridge
portions.
[0120] As shown here, ridges 242 with 242 a, b, c may be uniform
and symmetrical, resulting in a mirrored appearance with respect to
midline 250.
[0121] Although shown in cross-section with a form tantamount to a
`wave` with a curved or curvilinear ridge with a crest/trough (see
radii Ra, Rb, Rc), any ridge may be pyramidal, frustoconical, or
other printable shape, or have associated linear surfaces. The
presence of one or more ridges may provide narrowing between the
top deck 236a and the bottom deck 236b. In embodiments, h2 may be
less than h1.
[0122] As the fluid transitions from F2 to F3, the swirling effect
of the eddies promotes better heat transfer from the decks 236 a, b
to the fluid as it moves thereby. However, it may be useful to
curtail the turbidity by trailing the crest back to flat, planar
surfaces of the decks 236 a, b. The `calming` effect on the fluid
at point F3 may be useful for curtailing pressure drop through the
passageway 232. In embodiments, h3 may be about equal to h1. In
other embodiments, h3 may be less than h1, but greater than h2.
[0123] The fluid may then transition from F3 to F4 in the presence
of one or more ridges 242 a, b. As with ridges 242 and 242c, as the
fluid moves with appreciable velocity to position F4, the fluid may
eddy as a result of the narrowing space (see height h4) formed by
an at least one ridge portion. As shown here, there may be another
(a second, etc.) upper ridge portion 242b associated with the top
deck 236a and another (second, third, etc.) lower ridge portion
242a associated with the bottom deck 236b.
[0124] A subsequent calming of the fluid may occur as the fluid
transitions from F4 to F5, where again the top deck and the bottom
deck 236 a, b become flat or planar. As shown, the decks 236 a, b
at point F5 may be separated by clearance h1a. Although not meant
to be limited, h1a may be about equal to h1. In aspects, one or
both of h1 and h1a may be in the range of about 2 mm to about 4 mm.
The fluid may then exit what is tantamount to an `outlet` of the
cell module 230 and move to the next cell, or exit the core, and
out of the heat exchanger.
[0125] The flow regimes within passageway 232 may be epitomized by
surface geometry. One parameter of particular interest is the ratio
of d1:d2. Distance or depth d1 may be the length between an
entrance of the passageway 232 to ridge bisect or midpoint 244
(i.e., a reference line bisecting the approximate middle of ridge
242 and/or 242c). Distance or depth d2 may be the length between an
entrance of the passageway 232 to passageway bisect or midpoint 250
(i.e., a reference line bisecting the approximate middle of
passageway 232). In aspects, the ratio of d1:d2 nay be in the range
of about 0.4 to about 0.6. In aspects, the ratio of d1:d2 nay be in
the range of about 0.5. As result of symmetry, the ridges 242a
and/or 242b may be contemplated in a similar manner from the
opposite entrance (or exit, depending).
[0126] FIG. 2G is a look-through side view of passageway 234b. In
aspects, passageways 232 and 234 may be similar, or even exact to
each other. This may be the case when the fluids are comparable or
identical, such as two airstreams. However, passageways 232 and 234
may also differ, which may be particularly useful when the fluids
are of different mediums, namely, gaseous and liquidious. In
embodiments, air may flow through passageway 232, while a liquid
medium may flow through passageway 234.
[0127] The passageway 234b may be defined by side partitions 240 a,
b and the top deck 236b and the bottom deck 236c (noting that the
top deck 236b in this view is referenced as the bottom deck 236b
for passageway 234 in FIG. 2D). The partitions and decks may be
curvilinear in nature, including some partial linear and partial
curvature. One of or both of decks 236 b, c may have at least one
ridge 242. Although viewed here as a `wave` with a
crest/trough-type structure, the at least one ridge 242 could have
other shapes, such as pyramidal, frustoconical, etc.
[0128] The lateral distance d3 from an innermost surface of
partition 240a to an opposite innermost surface of partition 240b
may be in the range of about 5 mm to about 9 mm.
[0129] A crest 246 of the ridge 242 may have an amplitude 248 (with
reference to topmost surface 249 of deck 236b of topmost surface of
the crest 246) of about 0 mm (thus flat) to about 1.5 mm. In this
sense, the passageway 234 may have varied space or clearance
ranging from its narrowest height of h5 to its broadest or most
open height of h6. h5 may be in the range of about 0.1 to about 1.5
mm. h6 may be in the range of about 0.25 mm to about 3 mm. In some
embodiments, h5 may be equal to h6.
[0130] Embodiments herein provide for considerably smaller details
to be printed in a more dense nature than traditional cooler
manufacturing methods. Conventional manufacturing methods utilize a
stamping process to produce fins of various geometric shapes and
sizes. However typical stamping processes have limitations in the
resultant sizes and shapes that can be achieved. For example, too
thin of material will tear during the stamping process and complex
shapes and patterns may not be possible to stamp and then remove
from the die. Finally stamping has limitations on the fidelity of
the feature detail that is possible to transfer from the die to the
material plate being formed, very small and precise details may not
transfer properly to the plate during the process.
[0131] Casting material is not practical due to the complex shape
and need for cores and molds to form those shapes. Most casting
processes use casting sand or some non-metallic foam as the mold or
form. Keeping those patterns in the proper location during the
pouring and solidification process for such small compact details
is not possible. Machining evening using advanced CNC machines can
only achieve certain level of detail due to limitations of cutter
head size and axis of rotation of the cutting head during
machining.
[0132] Embodiments herein provide for the capability to manufacture
finer details in the fin geometry which can impact cooler
performance. Because smaller parts can be printed with great
fidelity, it is now possible to print these details into a far
greater density than can be achieved via traditional stamping,
casting or machining manufacturing processes. FIG. 3 shows a
conventional stamped cooler design with two fluid passages one at
the top and one at the bottom and a single cross fluid passage in
between (labeled as `prior art`). By way of an example comparison,
with in the same spacing (A and B), embodiments herein provide for
five fluid passages with five cross fluid passages is now possible.
The exact number of passages and spacing between them can vary
based on design needs and fluids being transferred through the
passages. More passageways, without detrimental pressure drop, is a
radical change (and substantial improvement in heat transfer) over
conventional coolers.
ADVANTAGES
[0133] Embodiments of the disclosure advantageously provide for an
improved heat exchanger unit useable with a wide array of heat
generating devices.
[0134] Advantages herein provide for a radiator core 3D printed out
of a base material such as aluminum with a specific base
metallurgical content. During manufacturing a tank may be welded to
the cooler core forming a sealed pressure vessel for the fluid
passage (such as water, glycol or oil) to flow through. During
conventional core manufacturing the cooler core is made by brazing
plates and fins together and then the tank is welded to the core.
The welding process generates a heat which is higher than the
melting point of the brazing material, thus causing what is
referred to as braze leaching. This leaching can weaken or even
fail the previously brazed joints of the core. With 3D printing the
melting point of the core material is now the same as the tank
material thus eliminating the potential for brazing failures at the
weld seam.
[0135] 3D printing allows for higher fidelity details in the fin
design which facilitates improved heat transfer between the two
fluid mediums. By creating special features such as bumps, risers,
curves, divots, windows and other features the fluid can be
controlled to create turbulence, eddy currents and laminar flow at
precise points along the fluid flow path thus facilitating increase
thermal efficiency and heat transfer. Traditional manufacturing
methods such as stamping simply do not allow for great enough
fidelity to generate these details to the level that 3D printing
can achieve.
[0136] 3D printing allows for the elimination of separation plates
which contain the cladding or brazing material used in conventional
cooler manufacturing. Using conventional manufacturing processes, a
separation plate between the two fluid passageways is required to
braze the fins from the two cross passages together. This plate is
cladded with a brazing material that melts at a certain temperature
thus brazing the fins and separator bars to together forming the
passageways for the fluid mediums. 3D printing eliminates the need
for this separation plate since the fins or top and bottom of one
passage way, actually can form the floor or roof of the passageway
that runs perpendicular to the other passageway. This eliminates
weight, space and cost of the parts used to create these
passages.
[0137] The process of alternative manufacturing for a cooler would
eliminate the potential for operator mistakes during the
manufacturing process of the cooler cores. Errors in stacking fins,
bars and plates with one another would be eliminated. More
importantly, the brazing process utilized today is not as
scientific as one might believe and the process leads to
inconsistency in the brazing results which effects cooler core
durability and operational pressures. 3D printing of cooler cores
would yield a greater consistency in material quality and molecular
structure of the core thus improving durability.
[0138] While embodiments of the disclosure have been shown and
described, modifications thereof can be made by one skilled in the
art without departing from the spirit and teachings of the
disclosure. The embodiments described herein are exemplary only,
and are not intended to be limiting. Many variations and
modifications of the disclosure presented herein are possible and
are within the scope of the disclosure. Where numerical ranges or
limitations are expressly stated, such express ranges or
limitations should be understood to include iterative ranges or
limitations of like magnitude falling within the expressly stated
ranges or limitations. The use of the term "optionally" with
respect to any element of a claim is intended to mean that the
subject element is required, or alternatively, is not required.
Both alternatives are intended to be within the scope of any claim.
Use of broader terms such as comprises, includes, having, etc.
should be understood to provide support for narrower terms such as
consisting of, consisting essentially of, comprised substantially
of, and the like.
[0139] Accordingly, the scope of protection is not limited by the
description set out above but is only limited by the claims which
follow, that scope including all equivalents of the subject matter
of the claims. Each and every claim is incorporated into the
specification as an embodiment of the present disclosure. Thus, the
claims are a further description and are an addition to the
preferred embodiments of the disclosure. The inclusion or
discussion of a reference is not an admission that it is prior art
to the present disclosure, especially any reference that may have a
publication date after the priority date of this application. The
disclosures of all patents, patent applications, and publications
cited herein are hereby incorporated by reference, to the extent
they provide background knowledge; or exemplary, procedural or
other details supplementary to those set forth herein.
* * * * *