U.S. patent application number 16/291788 was filed with the patent office on 2019-07-04 for optical fiber heat dissipation package.
This patent application is currently assigned to Teraxion. The applicant listed for this patent is Teraxion. Invention is credited to Ghislain Bilodeau, Dominic Faucher, Mathieu Faucher, Francois Seguin.
Application Number | 20190204502 16/291788 |
Document ID | / |
Family ID | 64014212 |
Filed Date | 2019-07-04 |
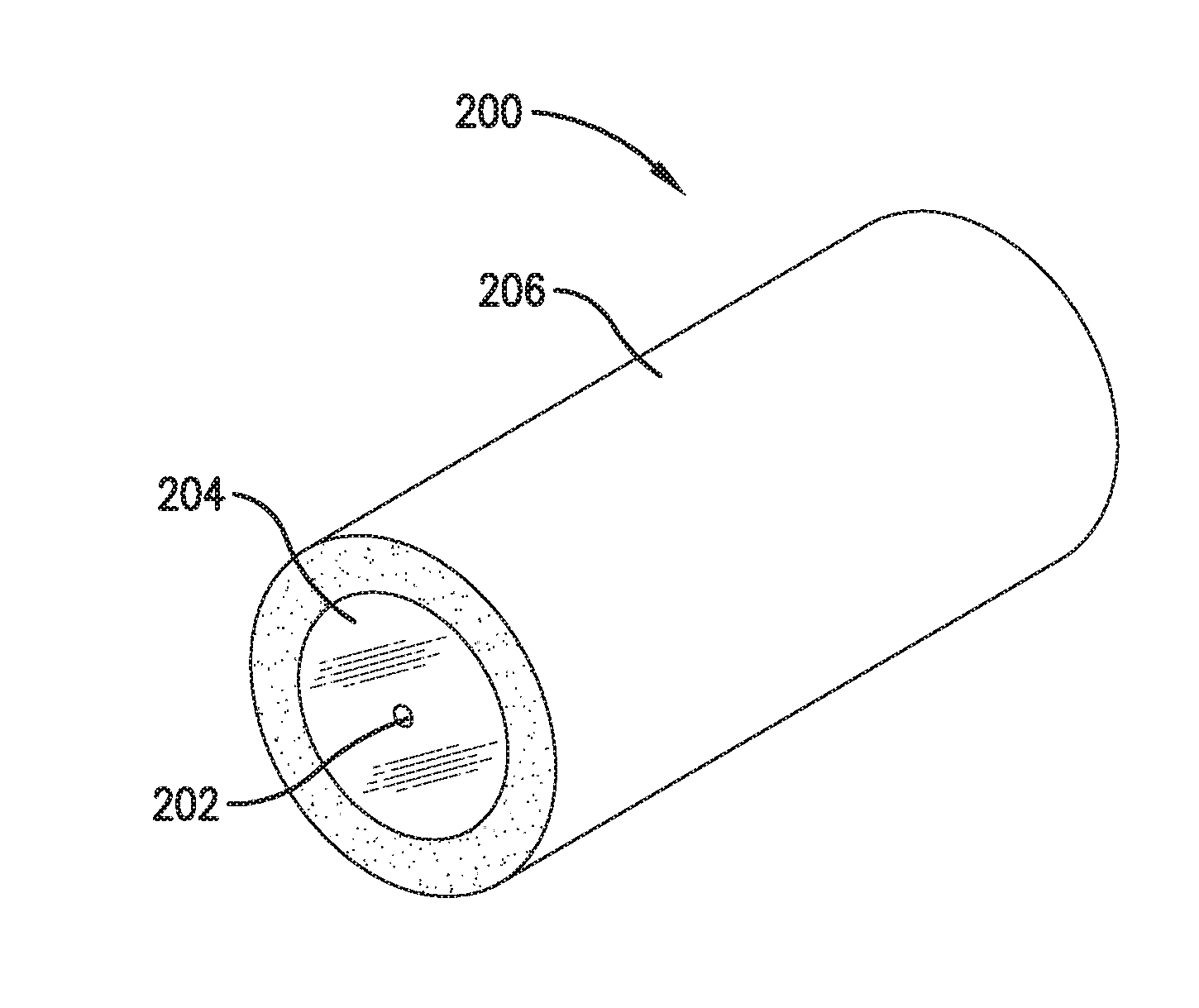
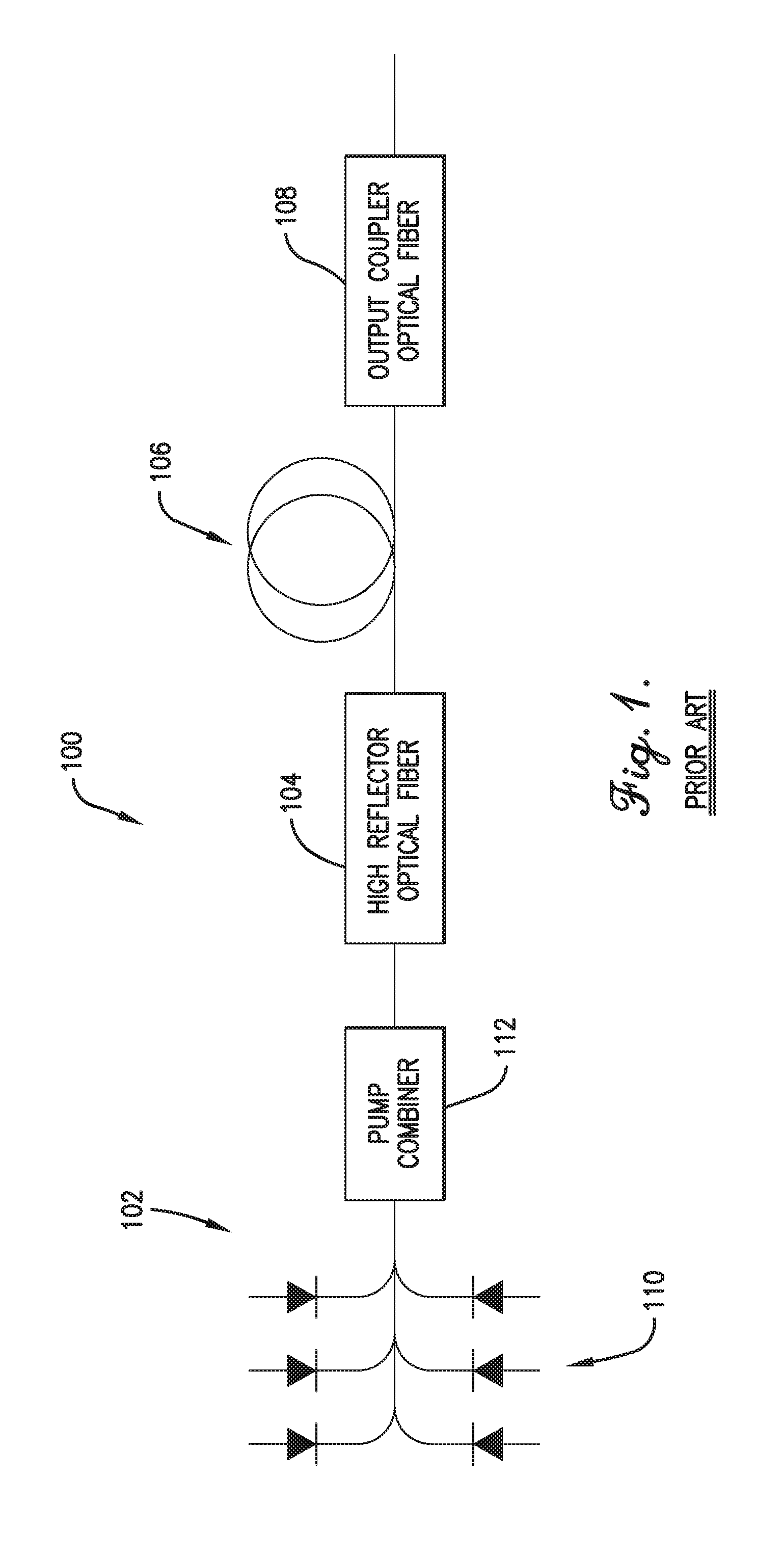
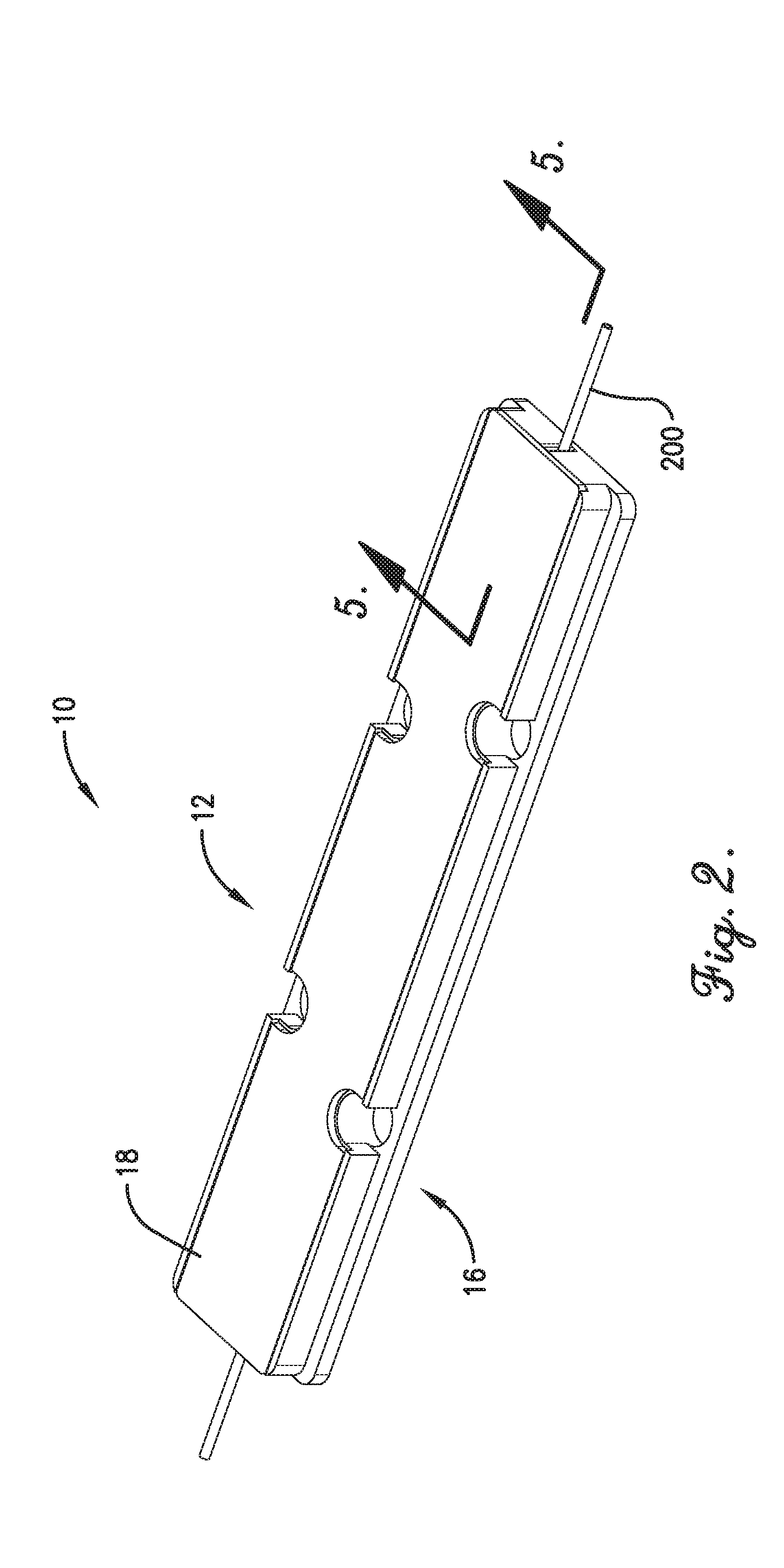
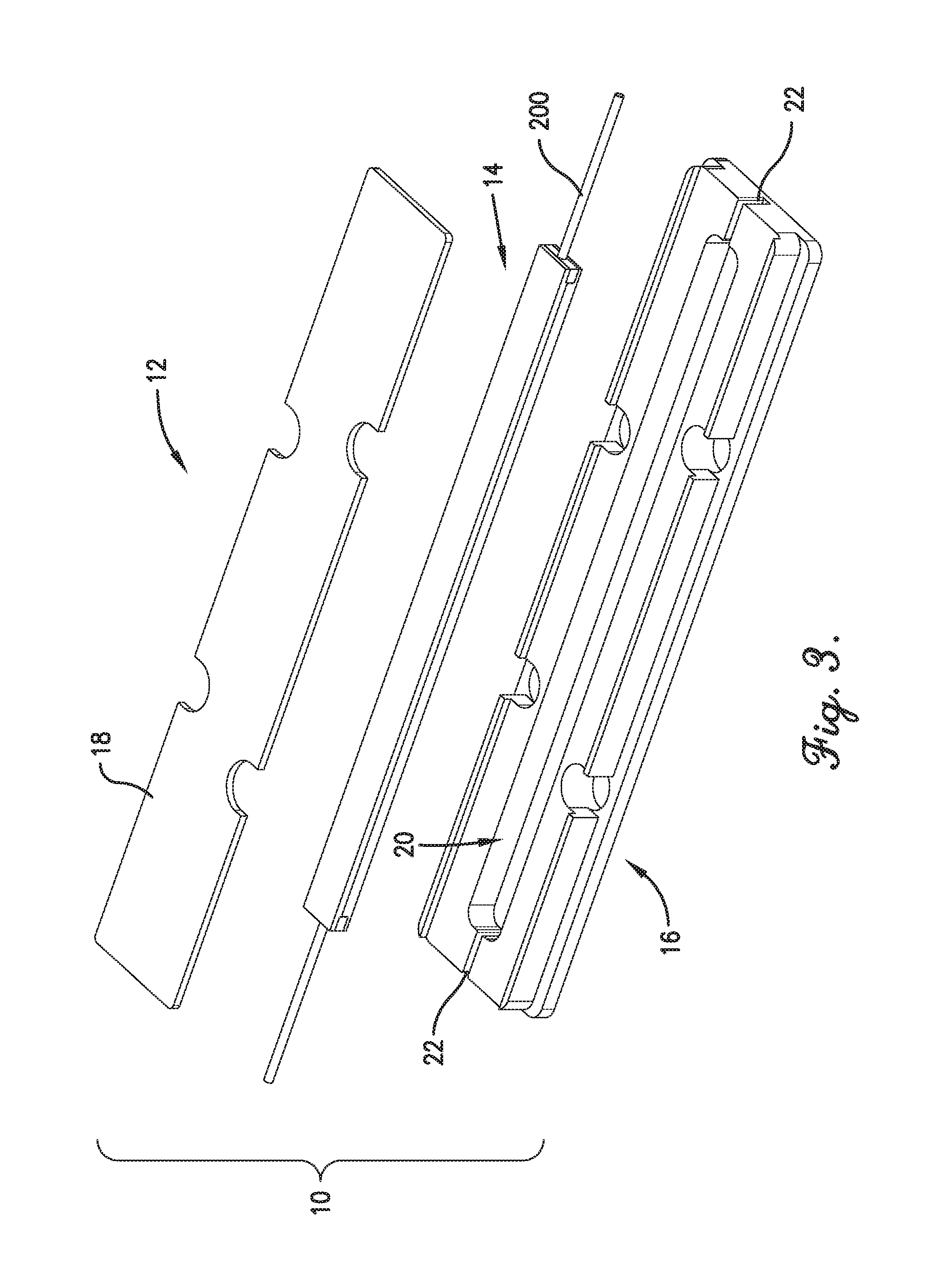
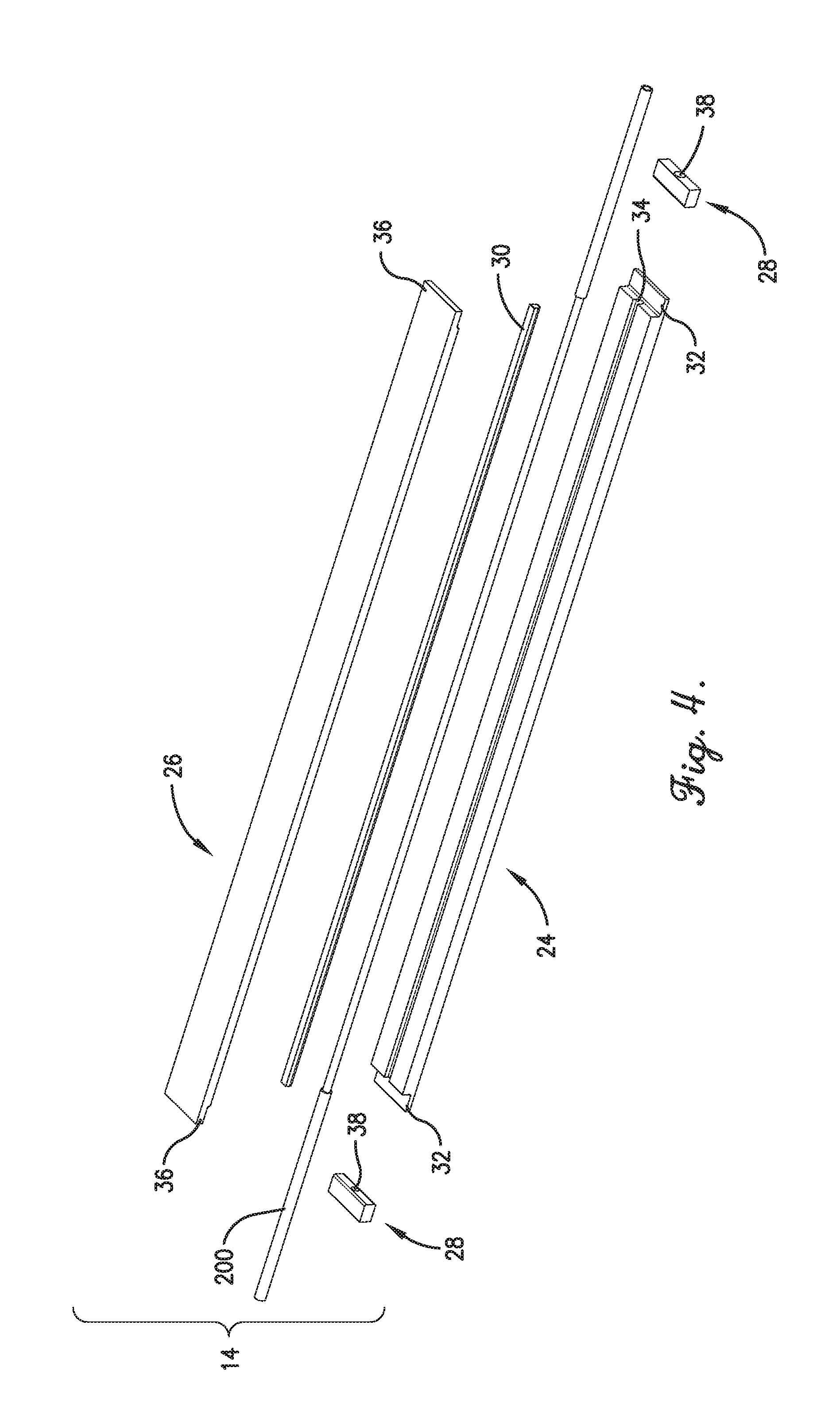
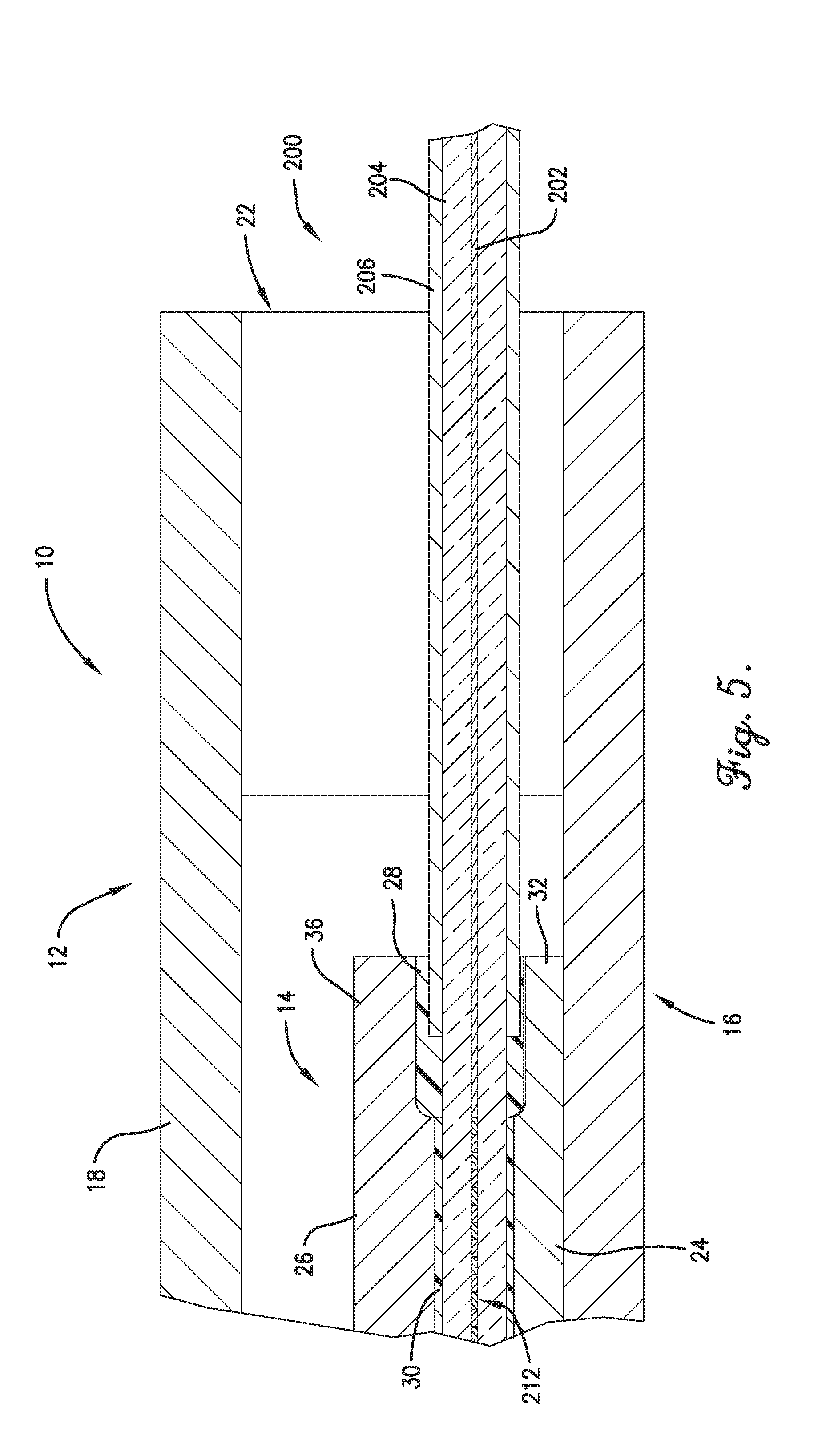
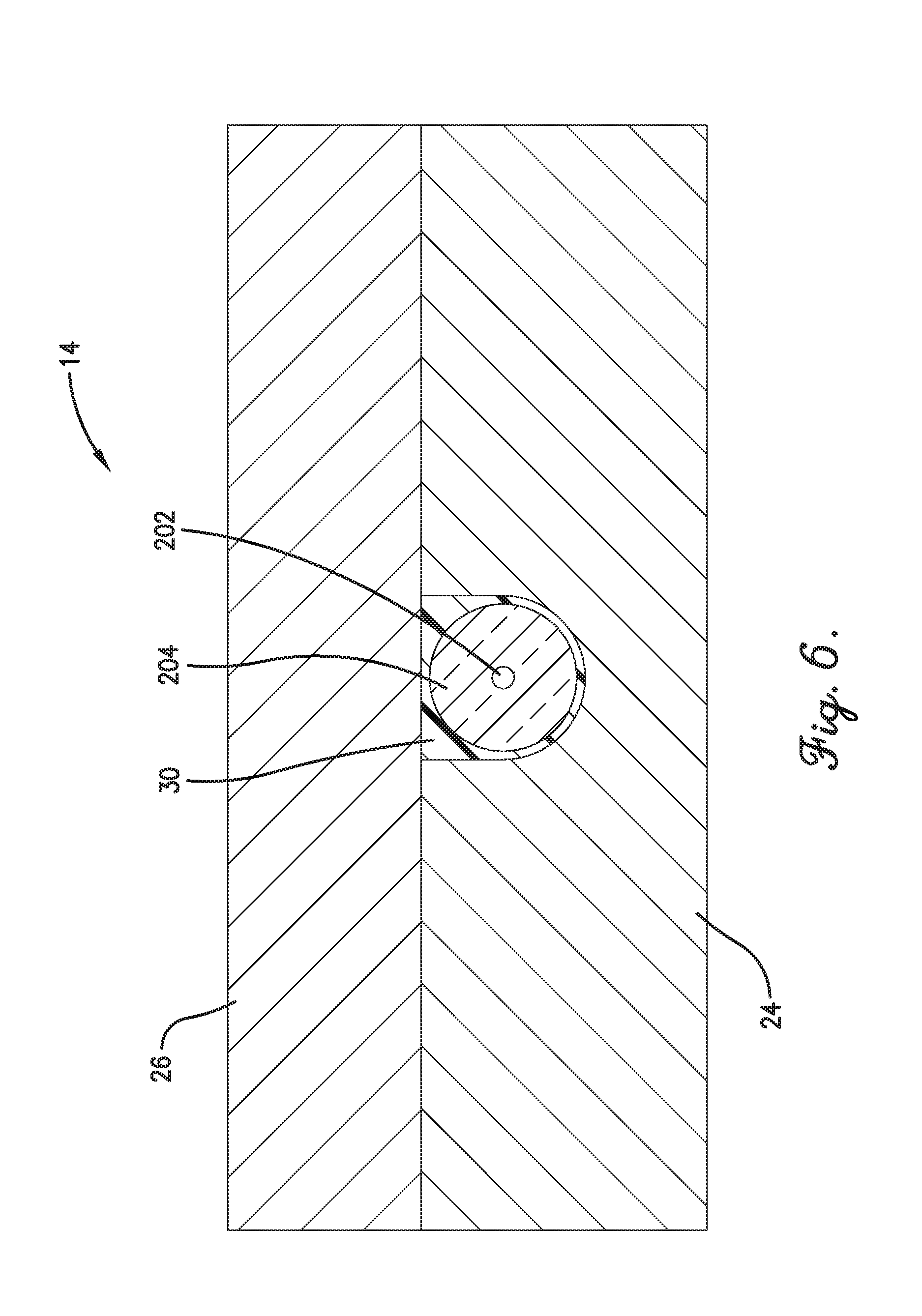


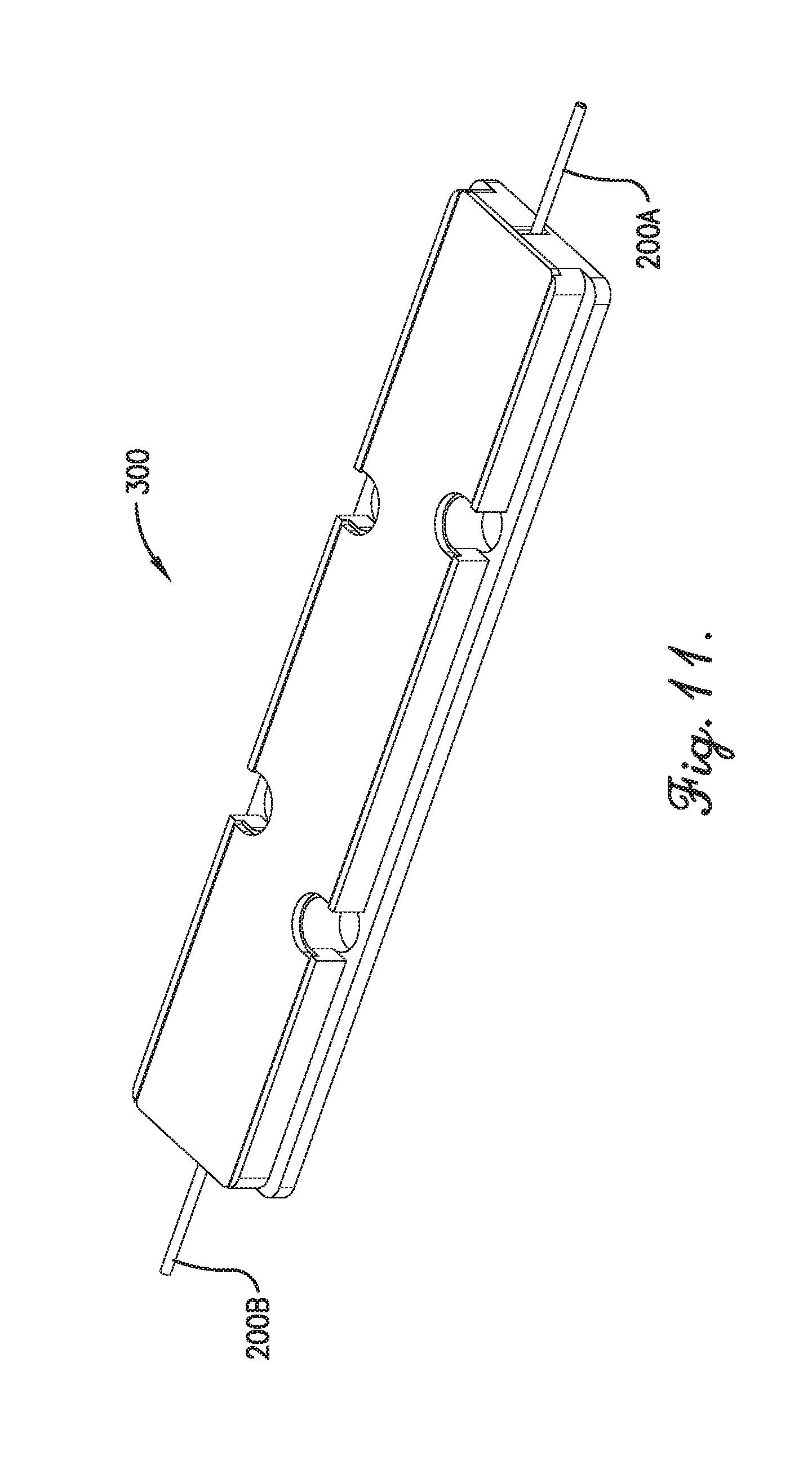

View All Diagrams
United States Patent
Application |
20190204502 |
Kind Code |
A1 |
Bilodeau; Ghislain ; et
al. |
July 4, 2019 |
OPTICAL FIBER HEAT DISSIPATION PACKAGE
Abstract
A heat-dissipation package for use with an optical fiber
includes a base, a cover, and a hollow sleeve. The base includes an
upper surface, a lower surface, and a groove embedded in the upper
surface, the groove having a generally U-shaped cross-sectional
shape. The cover is positioned on the upper surface of the base.
The sleeve includes a cylindrical inner surface and an outer
surface with a first portion which has a generally U-shaped cross
section and a second portion which has a generally planar cross
section such that edges of the planar cross section contact an open
end of the U-shaped cross section. The first portion of the outer
surface of the sleeve is positioned in the groove and the second
portion of the outer surface of the sleeve is in contact with the
cover. The sleeve is configured to encapsulate a heat-generating
section of the optical fiber.
Inventors: |
Bilodeau; Ghislain; (QUEBEC,
CA) ; Faucher; Dominic; (QUEBEC, CA) ;
Faucher; Mathieu; (QUEBEC, CA) ; Seguin;
Francois; (BAIE-D'URFE, CA) |
|
Applicant: |
Name |
City |
State |
Country |
Type |
Teraxion |
QUEBEC |
|
CA |
|
|
Assignee: |
Teraxion
QUEBEC
QC
|
Family ID: |
64014212 |
Appl. No.: |
16/291788 |
Filed: |
March 4, 2019 |
Related U.S. Patent Documents
|
|
|
|
|
|
Application
Number |
Filing Date |
Patent Number |
|
|
15584612 |
May 2, 2017 |
10288802 |
|
|
16291788 |
|
|
|
|
Current U.S.
Class: |
1/1 |
Current CPC
Class: |
G02B 6/02 20130101; G02B
6/44 20130101; G02B 6/4267 20130101; G02B 6/0218 20130101; G02B
6/036 20130101; G02B 6/02209 20130101; G02B 7/008 20130101 |
International
Class: |
G02B 6/02 20060101
G02B006/02; G02B 7/00 20060101 G02B007/00; G02B 6/44 20060101
G02B006/44; G02B 6/036 20060101 G02B006/036; G02B 6/42 20060101
G02B006/42 |
Claims
1. A heat-dissipation package for use with an optical fiber with a
heat-generating section, the optical fiber including a core which
is surrounded by at least one cladding which is surrounded by at
least one coating layer, the heat-generating section including the
core and the at least one cladding and occupying a portion of an
axial length of the optical fiber, the heat-dissipation package
comprising: a base including an upper surface, a lower surface, two
opposing end surfaces, and a groove embedded in the upper surface,
the groove having a generally U-shaped cross-sectional shape; a
cover including an upper surface, a lower surface, and two opposing
end surfaces, the cover positioned on the upper surface of the
base; and a hollow sleeve including a cylindrical inner surface and
an outer surface with a first portion which has a generally
U-shaped cross section and a second portion which has a generally
planar cross section such that edges of the planar cross section
contact an open end of the U-shaped cross section, the first
portion of the outer surface of the sleeve positioned in the groove
and the second portion of the outer surface of the sleeve in
contact with the cover, the sleeve configured to encapsulate the
heat-generating section of the optical fiber.
2. The heat-dissipation package of claim 1, further comprising two
fiber support blocks, each fiber support block of generally
rectangular box shape and each including a through hole with a
first section having a first diameter and a second section having a
second diameter, the first diameter being less than the second
diameter, each fiber support block configured to receive a portion
of the optical fiber in the through hole such that the first
section receives the core and the at least one cladding and the
second section receives the core, the at least one cladding, and
the at least one coating layer.
3. The heat-dissipation package of claim 2, wherein the base
further includes two pedestals, each pedestal extending outward
longitudinally from one end surface adjacent to the lower surface,
the cover further includes two overhangs, each overhang extending
outward longitudinally from one end surface adjacent to the upper
surface, and each fiber support block is positioned in the space
between one pedestal and one corresponding overhang.
4. The heat-dissipation package of claim 2, wherein the fiber
support blocks are formed of a material with an index of refraction
smaller than the index of refraction of the at least one
cladding.
5. The heat-dissipation package of claim 2, wherein the fiber
support blocks are formed from a fluoroacrylate polymer.
6. The heat-dissipation package of claim 1, wherein the sleeve is
formed from a material that has a thermal resistance less than
1.5.times.10.sup.-4 degree Kelvin meter.sup.2 per Watt between the
inner surface and outer surface.
7. The heat-dissipating package of claim 1, wherein the sleeve is
formed of a material with an index of refraction smaller than the
index of refraction of the at least one cladding.
8. The heat-dissipation package of claim 1, wherein the sleeve is
formed from a fluoroacrylate polymer and has a thickness ranging
from approximately 5 microns to approximately 30 microns.
9. The heat-dissipation package of claim 1, wherein the sleeve has
an axial length that is approximately the same as an axial length
of the heat-generating section of the optical fiber.
10. The heat-dissipation package of claim 1, wherein the cover and
the base are each formed from silicon or tungsten.
11. The heat-dissipation package of claim 1, wherein the cover is
formed from a material that is transmissive to ultraviolet
wavelength radiation.
12. The heat-dissipation package of claim 1, wherein the cover is
formed from multispectral zinc sulphide or synthetic sapphire.
13. The heat-dissipation package of claim 1, wherein the base is
formed from a material that has a thermal conductivity greater than
50 Watts per meter degree Kelvin and a coefficient of thermal
expansion less than 5.times.10.sup.-6 per degree Kelvin.
14. The heat-dissipation package of claim 1, wherein the cover is
formed from a material that has a thermal conductivity greater than
20 Watts per meter degree Kelvin and a coefficient of thermal
expansion less than 7.times.10.sup.-6 per degree Kelvin.
15. The heat-dissipation package of claim 1, further comprising a
housing including a body and a lid, the body including a central
cavity retaining the base and the cover and opposing ends, each end
having a channel configured to receive a portion of the optical
fiber.
16. The heat-dissipation package of claim 15, wherein the body is
formed from a material that has a thermal conductivity greater than
50 Watts per meter degree Kelvin and a coefficient of thermal
expansion less than 5.times.10.sup.-6 per degree Kelvin.
17. The heat-dissipation package of claim 15, wherein the body is
formed from tungsten.
Description
RELATED APPLICATION
[0001] The current patent application is a continuation patent
application which claims priority benefit with regard to all common
subject matter to U.S. patent application Ser. No. 15/584,612,
titled "OPTICAL FIBER HEAT DISSIPATION PACKAGE", and filed May 2,
2017. The earlier-filed utility application is hereby incorporated
by reference in its entirety into the current patent
application.
BACKGROUND OF THE INVENTION
Field of the Invention
[0002] Embodiments of the current invention relate to packaging for
use with optical fibers.
Description of the Related Art
[0003] Optical fiber laser systems 100, such as the exemplary
system of prior art FIG. 1, may include a lasing pump source 102, a
high reflector optical fiber 104, a gain optical fiber 106, and an
output coupler optical fiber 108. The lasing pump source 102 may be
provided by a plurality of laser diodes 110 and a pump combiner
112. Each laser diode 110 may generate laser light. The pump
combiner 112 may optically combine the laser light from the laser
diodes 110. The high reflector optical fiber 104 may include a
fiber Bragg grating (FBG) which acts as a mirror. The gain optical
fiber 106 may be spliced to the high reflector optical fiber 104
and may be doped with elements such as ytterbium or erbium to act
as a gain medium. The output coupler optical fiber 108 may be
spliced to the gain optical fiber 106 and may include an FBG which
acts as a partial mirror. The light output from the pump combiner
112 may be coupled into the high reflector optical fiber 104, which
along with the gain optical fiber 106 and the output coupler
optical fiber 108 form an optical resonator. The laser beam may be
provided by the output coupler optical fiber 108 or a delivery
optical fiber spliced thereto.
[0004] The FBG of the high reflector optical fiber 104 and the
output coupler optical fiber 108 typically absorbs a small fraction
of the incoming light. Contaminants that remain at the glass
surface of the optical fiber after the fabrication of an FBG can
also absorb some of the light propagating inside the fiber. This
absorption by the FBG and surface contaminants may produce heat and
an ensuing temperature increase that can be sizable when the
incoming optical power reaches kilowatt levels. The FBG and
surrounding materials may not be able to withstand this temperature
increase without sacrificing performance metrics such as spectral
response.
SUMMARY OF THE INVENTION
[0005] Embodiments of the current invention solve the
above-mentioned problems and provide a distinct advance in the art
of optical fiber packaging. Specifically, embodiments of the
current invention provide a heat-dissipation package that
effectively removes heat from the cladding of an optical fiber
implemented with a fiber Bragg grating. The heat-dissipation
package may broadly comprise a base, a cover, and a hollow sleeve.
The base includes an upper surface, a lower surface, and a groove
embedded in the upper surface, the groove having a generally
U-shaped cross-sectional shape. The cover is positioned on the
upper surface of the base. The sleeve includes a cylindrical inner
surface and an outer surface with a first portion which has a
generally U-shaped cross section and a second portion which has a
generally planar cross section such that edges of the planar cross
section contact an open end of the U-shaped cross section. The
first portion of the outer surface of the sleeve is positioned in
the groove and the second portion of the outer surface of the
sleeve is in contact with the cover. The sleeve is configured to
encapsulate a heat-generating section of the optical fiber.
[0006] Another embodiment of the current invention may provide a
heat-dissipation package for use with an optical fiber that broadly
comprises a housing and a submount. The housing includes a central
cavity and opposing ends, each end having a channel configured to
receive a portion of the optical fiber. The submount is positioned
in the central cavity and includes a base, a cover, two fiber
support blocks, and a hollow sleeve. The base includes an upper
surface, a lower surface, two opposing end surfaces, and a groove
embedded in the upper surface. The groove has a generally U-shaped
cross-sectional shape, with each end surface including a pedestal
extending longitudinally therefrom. The cover includes an upper
surface, a lower surface and two opposing end surfaces, with each
end surface including an overhang extending longitudinally
therefrom. One fiber support block is positioned in each of the
spaces created between one overhang and one corresponding pedestal.
Each fiber support block includes a through hole and is configured
to receive a portion of the optical fiber in the through hole. The
sleeve includes a cylindrical inner surface and an outer surface
with a first portion which has a generally U-shaped cross section
and a second portion which has a generally planar cross section
such that edges of the planar cross section contact an open end of
the U-shaped cross section. The first portion of the outer surface
of the sleeve is positioned in the groove and the second portion of
the outer surface of the sleeve is in contact with the cover. The
sleeve is configured to encapsulate the heat-generating section of
the optical fiber.
[0007] Yet another embodiment of the current invention may provide
an optical fiber laser system component broadly comprising an
optical fiber, a base, a cover, and a hollow sleeve. The optical
fiber has a fiber Bragg grating section and includes a core which
is surrounded by at least one cladding which is surrounded by at
least one coating layer. The fiber Bragg grating section includes
the core surrounded by the cladding. The base includes an upper
surface, a lower surface, and a groove embedded in the upper
surface, the groove having a generally U-shaped cross-sectional
shape. The cover is positioned on the upper surface of the base.
The sleeve includes a cylindrical inner surface and an outer
surface with a first portion which has a generally U-shaped cross
section and a second portion which has a generally planar cross
section such that edges of the planar cross section contact an open
end of the U-shaped cross section. The first portion of the outer
surface of the sleeve is positioned in the groove and the second
portion of the outer surface of the sleeve is in contact with the
cover. The sleeve is configured to encapsulate the fiber Bragg
grating section of the optical fiber.
[0008] Still another embodiment of the current invention may
provide an optical fiber assembly broadly comprising first and
second optical fibers, a base, a cover, and a hollow sleeve. The
optical fibers are spliced together with a fiber splice. Each
optical fiber includes a core which is surrounded by at least one
cladding which is surrounded by at least one coating layer and a
stripped section which abuts the fiber splice. The stripped section
includes the core surrounded by the at least one cladding. The base
includes an upper surface, a lower surface, and a groove embedded
in the upper surface, the groove having a generally U-shaped
cross-sectional shape. The cover is positioned on the upper surface
of the base. The sleeve includes a cylindrical inner surface and an
outer surface with a first portion which has a generally U-shaped
cross section and a second portion which has a generally planar
cross section such that edges of the planar cross section contact
an open end of the U-shaped cross section. The first portion of the
outer surface of the sleeve is positioned in the groove and the
second portion of the outer surface of the sleeve is in contact
with the cover. The sleeve is configured to encapsulate the
stripped sections of the first and second optical fibers.
[0009] This summary is provided to introduce a selection of
concepts in a simplified form that are further described below in
the detailed description. This summary is not intended to identify
key features or essential features of the claimed subject matter,
nor is it intended to be used to limit the scope of the claimed
subject matter. Other aspects and advantages of the current
invention will be apparent from the following detailed description
of the embodiments and the accompanying drawing figures.
BRIEF DESCRIPTION OF THE DRAWING FIGURES
[0010] Embodiments of the current invention are described in detail
below with reference to the attached drawing figures, wherein:
[0011] FIG. 1 is a schematic block diagram of a prior art optical
fiber laser system;
[0012] FIG. 2 is a perspective view of a heat-dissipation package,
constructed in accordance with various embodiments of the current
invention, for use with an optical fiber;
[0013] FIG. 3 is an exploded view of the heat-dissipation package
including a housing and a submount;
[0014] FIG. 4 is an exploded view of the submount including a base,
a cover, two support blocks, and a sleeve;
[0015] FIG. 5 is a cross-sectional view of one end of the
heat-dissipation package, cut along line 5-5 of FIG. 2, depicting
the interface of the optical fiber and the package;
[0016] FIG. 6 is a cross-sectional view of the submount cut along a
vertical plane transverse to the longitudinal axis depicting the
base, the cover, the sleeve, and the optical fiber encapsulated by
the sleeve;
[0017] FIG. 7 is a perspective view of an end of a first embodiment
of the optical fiber including a mechanical protection coating, a
cladding, and a core;
[0018] FIG. 8 is a perspective view of an end of a second
embodiment of the optical fiber including a secondary coating, a
primary low-index coating, a cladding, and a core;
[0019] FIG. 9 is cross-sectional view of the optical fiber, cut
along an axial plane, depicting the core with a first embodiment of
a fiber Bragg grating;
[0020] FIG. 10 is cross-sectional view of the optical fiber, cut
along an axial plane, depicting the core with a second embodiment
of a fiber Bragg grating;
[0021] FIG. 11 is a perspective view of an optical fiber assembly,
constructed in accordance with additional embodiments of the
current invention, the assembly including a fiber splice;
[0022] FIG. 12 is an exploded view of the optical fiber assembly of
FIG. 11 including a housing and a submount; and
[0023] FIG. 13 is an exploded view of the submount of the assembly
depicting the fiber splice.
[0024] The drawing figures do not limit the current invention to
the specific embodiments disclosed and described herein. The
drawings are not necessarily to scale, emphasis instead being
placed upon clearly illustrating the principles of the
invention.
DETAILED DESCRIPTION OF THE EMBODIMENTS
[0025] The following detailed description of the invention
references the accompanying drawings that illustrate specific
embodiments in which the invention can be practiced. The
embodiments are intended to describe aspects of the invention in
sufficient detail to enable those skilled in the art to practice
the invention. Other embodiments can be utilized and changes can be
made without departing from the scope of the present invention. The
following detailed description is, therefore, not to be taken in a
limiting sense. The scope of the present invention is defined only
by the appended claims, along with the full scope of equivalents to
which such claims are entitled.
[0026] In this description, references to "one embodiment", "an
embodiment", or "embodiments" mean that the feature or features
being referred to are included in at least one embodiment of the
technology. Separate references to "one embodiment", "an
embodiment", or "embodiments" in this description do not
necessarily refer to the same embodiment and are also not mutually
exclusive unless so stated and/or except as will be readily
apparent to those skilled in the art from the description. For
example, a feature, structure, act, etc. described in one
embodiment may also be included in other embodiments, but is not
necessarily included. Thus, the current technology can include a
variety of combinations and/or integrations of the embodiments
described herein.
[0027] A heat-dissipation package 10, constructed in accordance
with various embodiments of the current invention, for use with
optical fibers 200 in an optical fiber laser system 100 is shown in
FIGS. 2-6. A first heat-dissipation package 10 may retain the high
reflector optical fiber 104 and a second heat dissipation package
10 may retain the output coupler optical fiber 108 in the optical
fiber laser system 100 of FIG. 1.
[0028] The optical fiber 200 may generally have an exemplary
structure that includes a central core 202, a coaxial cladding 204,
and a coaxial coating 206, as seen in FIG. 7. The core 202 and the
cladding 204 may each be formed from a glass material with the
index of refraction of the cladding 204 being less than the index
of refraction of the core 202. The coating 206 may be formed from a
polymer material and generally provides mechanical protection and
minimizes stress for the cladding 204.
[0029] In another embodiment, the optical fiber 200 may include a
central core 202, a coaxial cladding 204, a coaxial first coating
208, and a coaxial second coating 210, as seen in FIG. 8. As above,
the core 202 and the cladding 204 may each be formed from a glass
material with the index of refraction of the cladding 204 being
less than the index of refraction of the core 202. The first
coating 208 may be from a polymer material, such as a soft,
low-index fluoroacrylate polymer, that has an index of refraction
less than the index of refraction of the cladding 204. Typically,
the difference in index of refraction between the first coating 208
and the cladding 204 is much greater than the difference in index
of refraction between the cladding 204 and the core 202 in order to
minimize penetration of light from the cladding 204 to the first
coating 208 and to be able to inject light into the cladding 204
instead of just the core 202. The second coating 210 may be formed
from a polymer material and generally provides mechanical
protection. Such a fiber is typically used in a high-power laser
system 100 where the cladding 204 is used to guide the light output
from the pump combiner 112. As known in the art, a fiber of the
type illustrated in FIG. 8 may have more than one cladding made of
glass (not illustrated).
[0030] When the optical fiber 200 is utilized as the high reflector
optical fiber 104 or the output coupler optical fiber 108, the core
202 of the optical fiber 200 may include a fiber Bragg grating
(FBG) 212. An exemplary FBG 212 may include a periodical modulation
of the index of refraction 214 extending along the longitudinal
axis of the optical fiber. In a first embodiment, seen in FIG. 9,
the modulation of the index of refraction has a uniform period. In
a second embodiment, seen in FIG. 10, the period of the modulation
of the index of refraction varies axially, which forms a chirped
FBG 212. The FBG 212 within the optical fiber 200 creates an
optical reflector for laser light. The wavelength of the light
reflected by the FBG 212 may vary according to, at least, the
period of the modulation of the index of refraction 214 and the
average value of the index of refraction. The strength of the
reflection depends on a length of the grating and an amplitude of
the index modulation.
[0031] The heat-dissipation package 10 may broadly comprise a
housing 12 and a submount 14, as best seen in FIG. 3. The housing
12 may include a body 16 and a lid 18. The body 16 may be of
generally solid elongated rectangular box shape with an upper
surface, a lower surface, two side surfaces, and two end surfaces.
The upper surface may be recessed from the tops of the side
surfaces. The body 16 may include an elongated cavity 20 with two
end channels 22. The cavity 20 may be positioned in the center of
the body 16 and aligned with the longitudinal axis thereof. The end
channels 22 are longitudinal axis aligned as well and extend from
the cavity 20 to each end surface. The body 16 may also include a
plurality of through holes from the top surface to the bottom
surface configured to receive screws, or the like, to mount the
package 10 to an external surface. In some embodiments, the body 16
may further include two flanges, each extending from one side
surface adjacent to the bottom surface.
[0032] The lid 18 may be of generally elongated rectangular shape
and relatively thin compared to its length and width. The lid 18
may include a top surface, a bottom surface, two side edges, and
two end edges. The lid 18 may also include through holes from the
top surface to the bottom surface which align with the through
holes of the body 16. The body 16 may be formed from material with
high thermal conductivity and mechanical strength.
[0033] The submount 14 may comprise a base 24, a cover 26, first
and second fiber support blocks 28, and a sleeve 30, as best seen
in FIG. 4. The base 24 may also be of generally solid elongated
rectangular box shape with an upper surface, a lower surface, two
side surfaces, and two end surfaces. The base 24 may further
include first and second pedestals 32, each of which extends
outward longitudinally from one of the end surfaces adjacent to the
lower surface, as seen in FIGS. 4 and 5. In some embodiments, a
corner of the joint between each pedestal 32 and its corresponding
end surface may be rounded. In addition, the base 24 may include a
groove 34 in the upper surface extending along a central axis line.
The groove 34 may have a U-shaped cross section. In alternative
embodiments, the groove 34 may have other cross-sectional shapes,
such as semi-circular, semi-oval, semi-elliptical, triangular,
square, rectangular, etc.
[0034] The cover 26 may be of generally solid elongated rectangular
box shape with an upper surface, a lower surface, two side
surfaces, and two end surfaces. In certain embodiments, the cover
26 may include a groove typically with a complementary
cross-sectional shape to that of the groove 34 in the base 24. The
cover 26 may further include first and second overhangs 36, each of
which extends outward longitudinally from one of the end surfaces
adjacent to the upper surface, as seen in FIGS. 4 and 5. In some
embodiments, a corner of the joint between each overhang 36 and its
corresponding end surface may be rounded. The base 24 and the cover
26 may each be formed from material with a high thermal
conductivity.
[0035] Each fiber support block 28 may be of generally solid
elongated rectangular box shape with an upper surface, a lower
surface, two side surfaces, and two end surfaces, as seen in FIGS.
4 and 5. In some embodiments, the corners between one end surface
and the upper and lower surfaces may be rounded. Each fiber support
block 28 may further include a through hole 38 extending,
transverse from the longitudinal axis of the block 28, from one end
surface to the opposing end surface. In addition, the through hole
38 may include a first section with a first diameter and a second
section with a second diameter, wherein the first diameter is less
than the second diameter. Each fiber support block 28 may be formed
from a polymer material with an index of refraction that is less
than the index of refraction of the cladding 204 of the optical
fiber 200.
[0036] The sleeve 30 may be hollow with a cylindrical inner surface
that has a circular cross-sectional shape. The sleeve 30 may have
an outer surface with a U-shaped cross section, such that a first
portion of the outer surface forms a U shape and a second portion
of the outer surface is planar and couples with the open end of the
first portion, as best seen in FIG. 6. The sleeve 30 may be
utilized to encapsulate the cladding 204 of the optical fiber 200
in region where the optical fiber 200 includes the FBG 212.
Therefore, the sleeve 30 may have a longitudinal axial length that
is approximately equal to the length of the FBG 212. In some
embodiments, the ends of the sleeve 30 may extend beyond the ends
of the FBG 212 by a predetermined length. The sleeve 30 may be
formed from a polymer material with an index of refraction that is
less than the index of refraction of the cladding 204 of the
optical fiber 200.
[0037] The submount 14 may have a construction as follows. The
sleeve 30 may be configured to receive, retain, and/or encapsulate
a portion of the longitudinal axial length of the cladding 204 and
the core 202 of an optical fiber 200 such that an outer surface of
the cladding 204 is in physical contact with the inner surface of
the sleeve 30. (Coatings on the optical fiber 200 are removed
before the optical fiber 200 is implemented with the
heat-dissipation package 10.) The sleeve 30 may be positioned
within the groove 34 of the base 24 such that the U-shaped portion
of the outer surface of the sleeve 30 is in physical contact with
the groove 34. The cover 26 may be positioned on the base 24 such
that the lower surface of the cover 26 is in physical contact with
the upper surface of the base 24 and the planar portion of the
outer surface of the sleeve 30. The cover 26 may be attached to the
base 24 with adhesives, fasteners, or the like. Once the cover 26
is on the base 24, the ends of the sleeve 30 may align with the end
surfaces of the cover 26 and the base 24.
[0038] The assembly of the base 24 and the cover 26 may form two
spaces, one space at each end of the submount 14 between one
overhang 36 and one corresponding pedestal 32. As seen in FIGS. 3
and 5, one fiber support block 28 may be positioned within each
space, such that the upper surface of the fiber support block 28
physically contacts the overhang 36, the lower surface of the fiber
support block 28 physically contacts the pedestal 32, and one end
surface of the fiber support block 28 physically contacts at least
a portion of the end surfaces of the base 24 and the cover 26.
Furthermore, each end of the sleeve 30 may physically contact a
portion of one end surface of one fiber support block 28. In
addition, the through hole 38 of each fiber support block 28 may be
configured to receive and/or retain a portion of the optical fiber
200 such that the cladding 204 and the core 202 of the optical
fiber 200 are positioned in the first section of the through hole
38, and the coating 206, the cladding 204, and the core 202 of the
optical fiber 200 are positioned in the second section of the
through hole 38, as illustrated in FIG. 5. When the optical fiber
has two coatings as illustrated in FIG. 8, the second section of
the through hole 38 may be configured to receive the second coating
210, the first coating 208, the cladding 204 and the core 202 (not
illustrated).
[0039] The heat-dissipation package 10 may have a construction as
follows. The submount 14 may be positioned in the cavity 20 such
that the lower surface of the base 24 contacts the body 16 of the
housing 12. In addition, the optical fiber 200 is retained by the
submount 14 and extends through the end channels 22. The optical
fiber 200 may be attached to the housing 12 with an adhesive in
each end channel 22, or within the cavity 20 adjacent to each end
channel 22. In various embodiments, the end channels 22 may be
filled with a soft material (not shown in the figures) such as
silicone to minimize the mechanical forces acting on the optical
fiber 200. The lid 18 may be positioned in the recess on the top
surface of the body 16 and may be attached thereto with adhesives
and/or fasteners.
[0040] At least one of the functions of the heat-dissipation
package 10 is transfer or remove heat from the FBG 212 of the
optical fiber 200 when the optical fiber 200 is used as the high
reflector optical fiber 104 or the output coupler optical fiber 108
in an optical fiber laser system 100. In various implementations of
the optical fiber laser system 100, light may be coupled into the
core 202 and the cladding 204 of the optical fiber 200 from the
pump combiner 112. In such a case, the package must also maintain
the transmission of light in the cladding 204. Hence, the index of
refraction of the hollow sleeve 30 must be smaller than the index
of refraction of the fiber cladding 204. An exemplary low-index
material may include a fluoroacrylate polymer. Furthermore, the
thickness of the sleeve 30 should be relatively large in order to
ensure that light guided in the cladding 204 does not reach the
outer boundary of the sleeve 30. The hollow sleeve must also allow
a good flow of heat in order to limit temperature increases at the
fiber 200. To this end, the thermal resistance of the hollow sleeve
30 between the fiber cladding 204 and the base 24 may be less than
approximately 1.5.times.10.sup.-4 degree Kelvin meter.sup.2 per
Watt (.degree. Km.sup.2/W). Typically, the sleeve 30 is formed from
material that has a low value of thermal conductivity. For example,
the thermal conductivity of fluoroacrylate can be as small as
approximately 0.2 Watts per meter degree Kelvin (W/m.degree. K).
Thus, the thickness (from the inner surface to the outer surface)
of the sleeve 30 should be small to provide sufficient heat
transfer away from the optical fiber 200. For optimum performance,
the sleeve 30 made of a fluoroacrylate may have a thickness ranging
from approximately 5 microns to approximately 30 microns, along its
smallest dimension such as the rounded part of the U-shaped outer
surface, perhaps best seen in FIG. 6.
[0041] The fiber support blocks 28 are also in direct contact with
the optical fiber 200, as seen in FIG. 5, and may be formed from a
material with the same properties as the sleeve 30. An exemplary
material from which the fiber support blocks 28 are formed may
include a fluoroacrylate polymer.
[0042] The base 24 and the cover 26 of the submount 14 may provide,
among other features, transfer or removal of heat from the sleeve
30 and may be formed from materials with high thermal conductivity.
However, the materials should also have a CTE similar to that of
the fiber 200, be easy to manufacture, and have a reasonable cost,
among other properties. Other considerations for the materials may
include transmission of, or transparency to, ultraviolet (UV)
wavelength radiation. In some instances, the sleeve 30 may be
formed, or coated, on the cladding 204 of the optical fiber 200 by
using a separate, external mold or by using other methods. In other
instances, the sleeve 30 may be formed, or coated, on the cladding
204 while the cladding 204 is positioned in the base 24 with the
cover 26 attached. In such circumstances, UV light transmitted
through the cover 26 could be used to cure the material of the
sleeve 30.
[0043] Exemplary materials that may be used to form the base 24 and
the cover 26 include synthetic diamond, copper, aluminum, silicon,
tungsten, synthetic sapphire, multispectral zinc sulphide, or the
like. Synthetic diamond has an extremely high thermal conductivity
and a CTE that is close to that of the optical fiber 200. However,
its low availability and high cost make it impractical for a
commercial product. Copper and aluminum have high thermal
conductivities and are easily machined, but they also have a high
CTE--making them incompatible with the optical fiber 200. Silicon
and tungsten have relatively high thermal conductivities and CTEs
which are closer to that of the optical fiber 200 than are copper
and aluminum. Synthetic sapphire and multispectral zinc sulphide
have CTEs which are closer to that of the optical fiber 200 than
are copper and aluminum, but their thermal conductivities are
relatively low. However, synthetic sapphire and multispectral zinc
sulphide have the advantage of being transmissive to UV light.
Thus, if the sleeve 30 is separately formed, then the base 24 and
the cover 26 may each be formed from either silicon or tungsten.
Alternatively, one may be formed from silicon, while the other is
formed from tungsten. If the sleeve 30 is to be UV cured in the
base 24 with the cover 26 attached, then typically the cover 26 may
be formed from either synthetic sapphire or multispectral zinc
sulphide. When the cover 26 is formed from either synthetic
sapphire or multispectral zinc sulphide, the base 24 may be formed
from silicon or tungsten. An exemplary material from which the base
24 is formed may have a thermal conductivity greater than 50
W/m.degree. K and a CTE less than 5.times.10.sup.-6/.degree. K. An
exemplary material from which the cover 26 is formed may have a
thermal conductivity greater than 20 W/m.degree. K and a CTE less
than 7.times.10.sup.-6/.degree. K.
[0044] The housing 12 may provide, among other features, transfer
or removal of heat from the submount 14. Generally, heat may
transfer from the submount 14 to the housing 12 through the
interface of the lower surface of the base 24 and the body 16 when
the submount 14 is positioned within the cavity 20 of the housing
12. The body 16 may be formed from materials with high thermal
conductivity. The body 16 may have similar constraints to those of
the base 24. For reasons similar to those discussed above, an
exemplary material for forming the body 16 may include tungsten. An
exemplary material from which the body 16 is formed may have a
thermal conductivity greater than 50 W/m.degree. K and a CTE less
than 5.times.10.sup.-6/.degree. K.
[0045] The heat-dissipation package 10, that is constructed with
the sleeve 30 having the dimensions discussed and with all of the
components being formed from the materials discussed, may have a
thermal slope of 0.005 degrees Celsius per Watt (.degree. C./W),
wherein the thermal slope of an object is the amount of temperature
increase of the object for the amount of power applied to it. The
thermal slope applies to the temperature increase per watt of pump
power in the cladding 204 as well as to the temperature increase
per watt of signal power in the fiber core 202. The thermal slope
of the heat-dissipation package 10 of the current invention allows
for multiple kilowatts of power to be applied while maintaining a
temperature of the components of the package 10 and the optical
fiber 200 that is within acceptable parameters.
[0046] It is generally desired to limit the temperature of the
coatings of the optical fiber 200 in order to maintain structural
integrity of the coating. For example, the temperature of a
fluoroacrylate coating should be kept at less than or equal to
approximately 70.degree. C. This temperature constraint also
applies to the sleeve 30 of the heat-dissipation package 10, since
the sleeve 30 encapsulates the cladding 204 in the same fashion as
the coatings. In an exemplary optical fiber laser system 100, a
pump power of 5 kilowatts (kW) and a signal power of 5 kW may be
applied, resulting in a temperature increase of 50.degree. C.
(0.005.degree. C./W.times.10 kW). This leads to the sleeve 30
having a temperature of 70.degree. C., assuming that the
temperature of the base 24 is 20.degree. C. In contrast, a high
reflector optical fiber 104 with two coating layers as illustrated
in FIG. 8, that would normally be utilized in an optical fiber
laser system 100 may have a thermal slope of 0.05.degree. C./W.
Therefore, the high reflector optical fiber 104, without the
heat-dissipation package 10 of the current invention, would be able
to handle only 10% of the applied power before the temperature of
the coatings exceeds the safe operating temperature of 70.degree.
C. Furthermore, the heat-dissipation package 10 of the current
invention may minimize hot spots created by contaminants at the
surface of the optical fiber 200 which generate temperature
gradients along the FBG 212 that will spoil its spectral
response.
[0047] In addition, when the components of the submount 14 and the
housing 12 are formed from materials with the ranges of values of
CTEs that are discussed above, the optical fiber 200 that is
retained in the heat-dissipation package 10 is better able to
withstand the thermal stresses which occur when a large pump power
and a large signal power are applied. Also, since the optical fiber
200 is attached to the housing 12 at two axially spaced apart
points, there is a slight tension on the fiber 200 that is less
likely to vary when the materials constituting the package 10 have
CTEs that are more closely matched to that of the optical fiber
200. Therefore, the heat-dissipation package 10 of the current
invention may offer greater structural reliability and improved
spectral response.
[0048] The heat-dissipation package 10 may also be utilized with
the packaging of two optical fibers 200A, 200B of the type
illustrated in FIG. 8 that are to be spliced to one another, which
creates an optical fiber assembly 300, as shown in FIGS. 11-13. As
an example, one end of each optical fiber 200A, 200B may be
stripped of its coating layers leaving a stripped section with just
the cladding 204 and the core 202. The ends of the optical fibers
200A and 200B may be fused together, creating a single optical
fiber 200 which includes a fiber splice 302 and has a length that
is just cladding 204 and core 202. Without using the
heat-dissipation package 10, the cladding 204 and core 202 could be
recoated with one or more layers, and the optical fiber 200 could
be utilized in a normal fashion. However, the stripping and
recoating procedure may leave contaminants at the surface of the
optical fibers 200A and 200B, which absorb optical power
propagating in the cladding 204 and generate heat. As a result,
performance of the spliced optical fiber 200 would suffer. To avoid
this situation, the spliced cladding 204 and core 202 may be
encapsulated by the sleeve 30, and the rest of the optical fiber
200 may be positioned in the heat-dissipation package 10 as
described above. The heat-dissipation package 10 may remove the
heat generated at the splice while preserving the transmission of
optical power through the optical fiber 200.
[0049] In another embodiment of the current invention, the
heat-dissipation package 10 may be combined with the optical fiber
200 including the FBG 212 to create the high reflector optical
fiber 104 or the output coupler optical fiber 108. The high
reflector optical fiber 104 or the output coupler optical fiber 108
may be constructed as shown in FIG. 2, wherein at least one end of
the optical fiber 200 may be spliced to the gain optical fiber 106
to form the optical resonator portion of the optical fiber laser
system 100.
[0050] Although the invention has been described with reference to
the embodiments illustrated in the attached drawing figures, it is
noted that equivalents may be employed and substitutions made
herein without departing from the scope of the invention as recited
in the claims.
* * * * *