U.S. patent application number 16/085361 was filed with the patent office on 2019-03-07 for method for temperature-treating a manganese steel intermediate product, and steel intermediate product which has been temperature-treated in a corresponding manner.
This patent application is currently assigned to VOESTALPINE STAHL GMBH. The applicant listed for this patent is VOESTALPINE STAHL GMBH. Invention is credited to Friedrich FUREDER-KITZMULLER, Daniel KRIZAN, Reinhold SCHNEIDER.
Application Number | 20190071748 16/085361 |
Document ID | / |
Family ID | 55640576 |
Filed Date | 2019-03-07 |


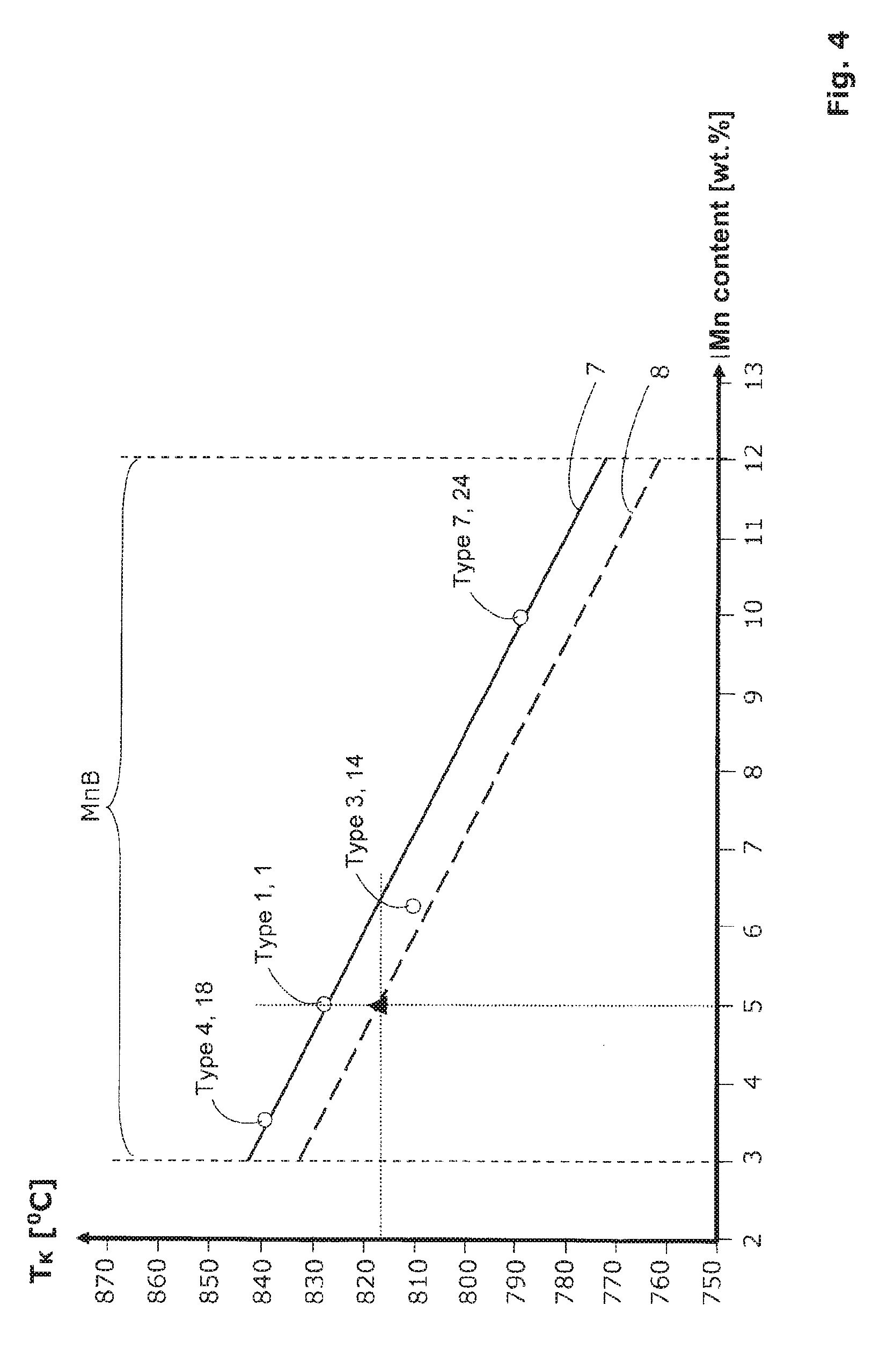


United States Patent
Application |
20190071748 |
Kind Code |
A1 |
FUREDER-KITZMULLER; Friedrich ;
et al. |
March 7, 2019 |
METHOD FOR TEMPERATURE-TREATING A MANGANESE STEEL INTERMEDIATE
PRODUCT, AND STEEL INTERMEDIATE PRODUCT WHICH HAS BEEN
TEMPERATURE-TREATED IN A CORRESPONDING MANNER
Abstract
A method for temperature-treating a manganese steel intermediate
product whose alloy includes: a manganese content, which lies in
the following manganese range: 3 wt. %.ltoreq.Mn.ltoreq.12 wt. %, a
fraction of one or more alloying elements of the group: silicon
(Si), aluminum (Al), nickel (Ni), chromium (Cr), molybdenum (Mo),
phosphorus (P), sulfur (S), nitrogen (N), copper (Cu), boron (B),
cobalt (Co), tungsten (W), an optional carbon content (C) of less
than 1 wt. %, an optional content of one or more microalloying
elements, wherein the total content of the micro-alloying elements
is less than 0.45 wt. %; and as a remainder an iron content (Fe)
and unavoidable impurities.
Inventors: |
FUREDER-KITZMULLER; Friedrich;
(Puchenau, AT) ; SCHNEIDER; Reinhold; (Wels,
AT) ; KRIZAN; Daniel; (Linz, AT) |
|
Applicant: |
Name |
City |
State |
Country |
Type |
VOESTALPINE STAHL GMBH |
Linz |
|
AT |
|
|
Assignee: |
VOESTALPINE STAHL GMBH
Linz
AT
|
Family ID: |
55640576 |
Appl. No.: |
16/085361 |
Filed: |
March 10, 2017 |
PCT Filed: |
March 10, 2017 |
PCT NO: |
PCT/EP2017/055714 |
371 Date: |
September 14, 2018 |
Current U.S.
Class: |
1/1 |
Current CPC
Class: |
C22C 38/04 20130101;
C21D 8/0205 20130101; C21D 1/26 20130101; C22C 38/06 20130101; C21D
6/005 20130101; C21D 9/52 20130101; C21D 8/0247 20130101; C22C
38/12 20130101 |
International
Class: |
C21D 9/52 20060101
C21D009/52; C22C 38/12 20060101 C22C038/12; C22C 38/06 20060101
C22C038/06; C22C 38/04 20060101 C22C038/04; C21D 8/02 20060101
C21D008/02; C21D 6/00 20060101 C21D006/00 |
Foreign Application Data
Date |
Code |
Application Number |
Mar 23, 2016 |
EP |
16162073.7 |
Claims
1: Method for temperature-treating a manganese steel intermediate
product whose alloy comprises: a manganese content (Mn), which lies
in the following manganese range (MnB): 3 wt. %.ltoreq.Mn.ltoreq.12
wt. %, a content of one or more alloying elements of the group:
silicon (Si), aluminum (Al), nickel (Ni), chromium (Cr), molybdenum
(Mo), phosphorus (P), sulfur (S), nitrogen (N), copper (Cu), boron
(B), tungsten (W), cobalt (Co), an optional carbon content (C) of
less than 1 wt. %, an optional content of one or more microalloying
elements, wherein the total content of the micro-alloying elements
is less than 0.45 wt. %; and as a remainder an iron content (Fe)
and unavoidable impurities, wherein the temperature-treating of the
steel intermediate product comprises a first temperature treatment
process (S.1) and a subsequent second temperature treatment process
(S.2), characterized in that the first temperature treatment
process (S.1) is a high-temperature process in which the steel
intermediate product is subjected during a first holding period
(.DELTA.1) to a first annealing temperature (T1) lying above a
critical temperature limit (T.sub.KG), which is defined as follows:
T.sub.KG=(856-S.sub.K*manganese content) degrees Celsius, wherein
S.sub.K is a slope value, and wherein said slope value
S.sub.K=7.83.+-.10%, preferably S.sub.K=7.83, the second
temperature treatment process (S.2) is an annealing process in
which the steel intermediate product is subjected to a second
annealing temperature (T2) which is lower than the first annealing
temperature (T1).
2: Method according to claim 1, characterized in that the first
annealing temperature (T1) in said manganese range (MnB) has a
dependence defined as follows:
T.sub.1.apprxeq.(866-S.sub.K*manganese content) degrees
Celsius.
3: Method according to claim 1, characterized in that the first
holding period (.DELTA.1) is at least 10 seconds and preferably
between 10 seconds and 6000 minutes.
4: Method according to claim 1, characterized in that the second
annealing temperature (T2) is in the range between the temperatures
A.sub.1 and .DELTA..sub.3, wherein A.sub.1 is the start temperature
of austenitization and A.sub.3 is the start temperature of full
austenitization.
5: Method according to claim 1, characterized in that the second
annealing temperature (T2) is in the range of 630.degree. C. to
675.degree. C.
6: Method according to claim 1, characterized in that within the
scope of the second temperature treatment process (S.2), the second
annealing temperature (T2) is held during a second holding period
(.DELTA.2) of at least 10 seconds.
7: Method according to claim 1, characterized in that the second
temperature treatment process (S.2), including a heating process
(E2) of the steel intermediate product, the holding (H2) of the
second annealing temperature (T2) and a cooling process (A2) of the
steel intermediate product, takes less than 6000 minutes and
preferably less than 5000 minutes.
8: Method according to claim 1, characterized in that the content
of the one or more alloying elements is in the following range:
silicon (Si) .ltoreq.3 wt. %, and preferably .ltoreq.2 wt. %,
aluminum (Al) .ltoreq.8 wt. %, and preferably .ltoreq.6 wt. %,
nickel (Ni) .ltoreq.2 wt. %, and preferably .ltoreq.1 wt. %,
chromium (Cr) .ltoreq.2 wt. %, and preferably .ltoreq.0.5 wt. %,
molybdenum (Mo) .ltoreq.0.5 wt. %, and preferably .ltoreq.0.25 wt.
%, phosphorus (P) .ltoreq.0.05 wt. %, and preferably .ltoreq.0.025
wt. %, sulfur (S) .ltoreq.0.03 wt. %, and preferably .ltoreq.0.01
wt. %, nitrogen (N) .ltoreq.0.05 wt. %, and preferably
.ltoreq.0.025 wt. %, copper (Cu) .ltoreq.1 wt. %, and preferably
.ltoreq.0.5 wt. %, boron (B) .ltoreq.0.005 wt. %, and preferably
.ltoreq.0.0035 wt. %, tungsten (W) .ltoreq.1 wt. %, and preferably
.ltoreq.0.5 wt. %, cobalt (Co) .ltoreq.2 wt. %, and preferably
.ltoreq.1 wt. %.
9: Method according to claim 1, characterized in that the
micro-alloying elements are elements of the group: titanium (Ti),
niobium (Nb), vanadium (V).
10: Method according to claim 1, characterized in that the first
temperature treatment process (S.1) concerns a process which is
carried out in a continuous strip plant or in a discontinuously
operating plant.
11: Method according to claim 1, characterized in that the second
temperature treatment process (S.2) is a process which is carried
out in a continuous strip plant or in a discontinuously operating
plant, wherein the steel intermediate product in this plant is
exposed in the annealing process to a protective gas
atmosphere.
12: Method according to claim 11, characterized in that a hood-type
annealing device is used as a discontinuously operating plant.
13: Method according to claim 1, characterized in that the steel
intermediate product is subjected to a skin-pass rolling process in
a step which is downstream of the second temperature treatment
process (S.2), wherein this skin-pass rolling process is primarily
directed to condition the surface of the steel intermediate
product.
14: Method according to claim 1, characterized in that the first
temperature treatment process (S.1) is carried out during a hot
rolling process, wherein said hot rolling process is carried out
with a rolling end temperature which lies in the range above the
critical temperature limit (T.sub.KG).
15: Steel intermediate product which has been heat-treated
according to a method of claim 1, characterized in that it has a
Luders strain (A.sub.L) which is less than 3% and preferably less
than 1%.
16: Steel intermediate product according to claim 15, characterized
in that a Luders strain (A.sub.L) of less than 3% on the steel
intermediate product is measurable before the steel intermediate
product was subjected to a subsequent skin-pass rolling
process.
17: Steel intermediate product according to claim 15, characterized
in that, due to a reduced Luders strain (A.sub.L), in comparison to
the reduction of Luders strain with skin-pass rolling, it has a
greater useful technical elongation.
Description
[0001] The present invention relates to a method of temperature
treating a manganese steel intermediate product. It also relates to
a specific alloy of a manganese steel intermediate product, which
is temperature-treated by a special process to achieve a
significantly reduced Luders strain. This application claims the
priority of European Patent Application Number EP 16 162 073.7,
filed on 23 Mar. 2016.
[0002] Both the composition and the alloy, respectively, as well as
the heat treatment in the manufacturing process have a significant
influence on the properties of steel products.
[0003] It is known that in the course of a heat treatment, the
warming-up, holding and cooling can have an influence on the final
structure of a steel product. Furthermore, as already indicated,
the alloy composition of the steel product obviously also plays a
major role. The thermodynamic and material-technical relationships
in alloyed steels are very complex and depend on many
parameters.
[0004] It has been shown that a combination of different phases in
the microstructure of a steel product can influence the mechanical
properties and the deformability.
[0005] Depending on the specific requirement profile, different
steels are used.
[0006] An important component of today's new steel alloys is
manganese (Mn). These are so-called medium-manganese steels. The
manganese content in weight percent (wt. %) is often in the range
between 3 and 12. Due to its microstructure, a medium-manganese
steel has a high combination of tensile strength and elongation.
Typical applications in the automotive industry are complex
safety-relevant deep-drawn components.
[0007] In FIG. 1, a classical, highly schematic diagram is shown,
in which the elongation at break A.sub.80 (also known as total
elongation) is plotted in percent over the tensile strength in MPa.
The tensile strength is abbreviated here with R.sub.m. The diagram
of FIG. 1 gives an overview of the strength classes of currently
used steel materials for the automotive industry. In general, the
following statement applies: the higher the tensile strength
R.sub.m of a steel alloy, the lower the total elongation A.sub.80
of this alloy. In simple terms, it can be stated that the total
elongation A.sub.80 decreases with increasing tensile strength
R.sub.m and vice versa. It is therefore necessary to find an
optimal compromise between the total elongation A.sub.80 and the
tensile strength R.sub.m for each application.
[0008] In the automotive sector, one works with a whole range of
different steel alloys, each of which has been optimized
specifically for their particular application area on the vehicle.
For interior and exterior panels, structural parts and bumpers,
alloys are used which have good energy absorption. Steel panels for
the outer skin of a vehicle are relatively "soft" and have for
example a tensile strength R.sub.m of approx. 300 MPa and a good
total elongation A.sub.80>30%. The steel alloys of
safety-relevant components, for example, have a tensile strength
R.sub.m in the range between 600 and 1000 MPa. For example, the
TRIP (transformation-induced plasticity) steels (reference numeral
1 in FIG. 1) are very well suited for this purpose.
[0009] For steel barriers (e.g. for side impact protection), which
should prevent the ingress of vehicle parts in an accident, steel
alloys are used which have a high tensile strength R.sub.m of
usually more than 1000 MPa. In this case, for example, the new
generation of higher-strength AHSS (Advanced High-Strength Steels)
steels is suitable (reference numeral 2 in FIG. 1). This category
includes the TBF (Trip Bainitic Ferrite) steels and the Q&P
(Quenching & Partitioning) steels. These high-strength AHSS
steels have, for example, a manganese content in the range between
1.2 and 3 wt. % and a carbon content C which is between 0.05 and
0.25 wt. %.
[0010] In the area designated by the reference numeral 3 in FIG. 1,
the already mentioned medium-manganese steels are schematically
summarized. The area designated by the reference numeral 3
comprises medium-manganese steels having an Mn content of between 3
and 12 wt. % and a carbon content of .ltoreq.1 wt. %.
[0011] Today's medium manganese steels have a pronounced yield
strength due to their ultra-fine grain (typically .ltoreq.1 .mu.m),
which is clearly visible in the tensile test. An exemplary tensile
curve 4 (also called stress-strain curve) is shown in FIG. 2. In
FIG. 2, the tension .sigma. (in MPa) is plotted over the elongation
.epsilon. (in %). The tensile curve 4 shows an intermediate maximum
5, which is referred to as the upper yield strength (R.sub.eH),
followed by a plateau 6. In the region of the lower yield strength
(R.sub.eL), the plateau 6 changes into a rising curve region. The
"length" of the plateau 6 is referred to as Luders strain
(A.sub.L), as shown in FIG. 2. A steel product with such a
pronounced yield strength can form undesirable Luders bands
(stretcher-strainer marks) on the surface of the components for the
automobile industry. Therefore, the pronounced yield strength
typically needs to be reduced by a re-rolling process. The
aftertreatment in a corresponding re-rolling plant (usually with a
skin-pass mill) is also referred to as skin-pass rolling.
[0012] The energetic and technical effort for skin-pass rolling is
sometimes quite high. In addition, this process leads to a
reduction of the usable elongation.
[0013] It is therefore the object to develop a method for the
production of manganese steel intermediate products in which the
Luders strain is less pronounced. Preferably, the manganese steel
intermediate products should have no (measurable) Luders
strain.
[0014] Investigations on numerous alloy compositions of
medium-manganese steels have shown that there is a correlation
between the original austenite grain size of these steels and the
Luders strain. This means that the original austenite grain size
has an influence on the mechanical properties of these steels. In
general, it can be postulated that the Luders strain behaves
inversely proportional to the original austenite grain size.
[0015] The partial object of the invention is thus to find an alloy
composition and a process for temperature treatment in order to
increase the original austenite grain size and to manifest the
increased austenite grains in the structure of the medium-manganese
steels. Unlike the prior art (see WO2014095082 A1, for example),
which concerns the provision of ultrafine structures (with an
ultrafine grain having an average particle size of about 1 .mu.m),
the invention aims in a different direction. In addition, in the
exemplified patent application WO2014095082 A1, a double annealing
process is used, which works with other temperatures and process
procedures. Steel products made by the method of WO2014095082A1
have a pronounced yield strength.
[0016] In accordance with the invention, there is provided a
particularly suitable manganese steel alloy and an optimized
process for temperature-treating a manganese steel intermediate
product.
[0017] The manganese steel alloy of the invention comprises: [0018]
a manganese content (Mn) which is in the following manganese range
3 wt. %.ltoreq.Mn.ltoreq.12 wt. %, [0019] a content of one or more
alloying elements of the group: silicon (Si), aluminum (Al), nickel
(Ni), chromium (Cr), molybdenum (Mo), phosphorus (P), sulfur (S),
nitrogen (N), Copper (Cu), boron (B), cobalt (Co), tungsten (W),
[0020] an optional carbon content (C) of less than 1 wt. %, [0021]
an optional content of one or more micro-alloying elements such as:
titanium (Ti), niobium (Nb) and vanadium (V), wherein the total
content of microalloying elements is less than 0.45 wt. %, and
[0022] the remainder being an iron content (Fe) and unavoidable
impurities.
[0023] The manganese steel intermediate products which have been
produced from a melt of this manganese steel alloy are subjected
within the scope of a temperature treatment according to the
invention to a first temperature treatment process and a subsequent
second temperature treatment process.
[0024] The first temperature treatment process is a
high-temperature process in which the steel intermediate product is
subjected during a first holding period to a first annealing
temperature which is above a critical temperature limit (referred
to as T.sub.KG), wherein this critical temperature limit (T.sub.KG)
is defined as follows: T.sub.KG.gtoreq.(856-S.sub.K*manganese
content) degrees Celsius, and wherein S.sub.K is a slope value.
[0025] The aforementioned formula, which serves as a definition of
the critical temperature limit (T.sub.KG), states that the critical
temperature limit (T.sub.KG) decreases in the manganese range
mentioned with increasing manganese content.
[0026] The aforementioned slope value is preferably defined in all
embodiments as follows: S.sub.K=7.83.+-.10% and particularly
preferably at S.sub.K=7.83.
[0027] The second temperature treatment process is an annealing
process in which the steel intermediate product is subjected to a
second annealing temperature T2, which is in each case lower than
the first annealing temperature T1.
[0028] Preferably, the first annealing temperature T1 shows in all
embodiments a dependence on said manganese range of the alloy,
which is defined as follows: T1.gtoreq.T.sub.KG.
[0029] Particularly preferred are embodiments of the invention at a
critical temperature T.sub.K.gtoreq.(866-S.sub.K*manganese content)
degrees Celsius, where the following applies:
S.sub.K=7.83.+-.10%.
[0030] Preferably, the first holding period is at least 10 seconds
in all embodiments. Particularly preferably, the first holding
period in all embodiments is between 10 seconds and 7000
minutes.
[0031] Preferably, the second annealing temperature T2 is in all
embodiments in the range between the temperatures A.sub.1 and
A.sub.3.
[0032] Advantageous results are obtained if the second temperature
treatment process, including the heating of the steel intermediate
product, the holding the second annealing temperature and the
cooling of the steel intermediate product, takes less than 6000
minutes. Preferably, this total time is even less than 5000
minutes.
[0033] The invention can be applied particularly advantageously to
alloys in which the proportion of the one or more alloying elements
lies in the following range: [0034] silicon (Si) .ltoreq.3 wt. %,
and preferably .ltoreq.2 wt. %, [0035] aluminum (Al) .ltoreq.8 wt.
%, and preferably .ltoreq.6 wt. %, [0036] nickel (Ni) .ltoreq.2 wt.
%, and preferably .ltoreq.1 wt. %, [0037] chromium (Cr) .ltoreq.2
wt. %, and preferably .ltoreq.0.5 wt. %, [0038] molybdenum (Mo)
.ltoreq.0.5 wt. %, and preferably .ltoreq.0.25 wt. %, [0039]
phosphorus (P) .ltoreq.0.05 wt. %, and preferably .ltoreq.0.025 wt.
%, [0040] sulfur (S) .ltoreq.0.03 wt. %, and preferably
.ltoreq.0.01 wt. %, [0041] nitrogen (N) .ltoreq.0.05 wt. %, and
preferably .ltoreq.0.025 wt. %, [0042] copper (Cu) .ltoreq.1 wt. %,
and preferably .ltoreq.0.5 wt. %, [0043] boron (B) .ltoreq.0.005
wt. %, and preferably .ltoreq.0.0035 wt. %, [0044] tungsten (W)
.ltoreq.1 wt. %, and preferably .ltoreq.0.5 wt. %, [0045] cobalt
(Co) .ltoreq.2 wt. %, and preferably .ltoreq.1 wt. %.
[0046] Advantageous results are shown in all embodiments in which
elements of the following group are used as micro-alloying
elements: titanium (Ti), niobium (Nb), vanadium (V).
[0047] For the first time, the invention makes it possible to
provide steel intermediate products having a Luders strain A.sub.L
which is less than 3% and preferably less than 1%.
[0048] At the same time, the steel intermediate products of the
invention preferably have an average primary austenite grain size
greater than 3 .mu.m in all embodiments.
[0049] The alloy of the steel intermediate products of the
invention preferably has an average manganese content according to
the invention, which means that the manganese content is in the
range of 3 wt. %.ltoreq.Mn.ltoreq.12 wt. %. Preferably, the
manganese content in all embodiments is in the range 3.5 wt.
%.ltoreq.Mn.ltoreq.8.5 wt. %.
[0050] The carbon content of the steel products of the invention is
generally rather low. In addition, the carbon content is optional
in all embodiments. That is, the carbon content is in the range C 1
wt. % in the invention. Embodiments in which the carbon content is
in one of the following ranges are particularly preferred
[0051] a) 0.01.ltoreq.C.ltoreq.0.8 wt. %, or
[0052] b) 0.05.ltoreq.C.ltoreq.0.3 wt. %.
[0053] In a preferred method of the invention, the first
temperature treatment process is carried out in a continuous strip
plant (annealing plant). This process is also known as continuous
annealing. Or another possibility is a discontinuous heat treatment
(hood-type annealing) of the steel intermediate product.
[0054] If the temperature treatment of a hot strip is concerned,
the first temperature treatment of the invention can also be
carried out by a special temperature control during hot rolling.
With this special temperature control, care is taken to ensure that
the rolling end temperature of the hot strip during hot rolling is
in the range above the critical temperature limit T.sub.KG.
[0055] In a preferred method of the invention, the second
temperature treatment process is carried out in a discontinuously
operating plant, wherein the steel intermediate product is
subjected to the annealing process in this plant in a protective
gas atmosphere. This process is preferably carried out in a
hood-type annealing plant. However, the second temperature
treatment process can also be carried out in all embodiments in a
continuous strip plant (annealing plant) or in a hot-dip
galvanizing plant.
[0056] The steel intermediate product of all embodiments may
optionally be subjected to a skin-pass rolling process, which is
primarily directed to conditioning the surface of the steel
intermediate product. A more intensive skin-pass rolling is not
required because the steel intermediate products of the invention
have a low Luders strain.
[0057] Thus, with the invention, the degree of skin-pass rolling
can be reduced or completely avoided.
[0058] It is an advantage of the invention that steel intermediate
products can be made which have a Luders strain which is less than
3% and which is preferably less than 1%.
[0059] It is an advantage of the invention that steel intermediate
products can be produced which have a tensile strength R.sub.m
(also called minimum strength) which is greater than 490 MPa.
[0060] It is an advantage of the invention that steel intermediate
products can be produced which as a result of the reduced Luders
strain have a (minimum) total elongation (A.sub.80) which is
greater than 10%.
[0061] It is an advantage of the invention that the steel
intermediate products have an increased technically usable
elongation due to the reduced Luders strain.
[0062] The invention can be used, for example, to provide cold
rolled steel products in the form of cold rolled flat products
(e.g. coils). The invention can also be used, for example, to
produce thin sheets or also wires and wire products.
[0063] The invention can also be used to provide hot strip steel
products.
[0064] Further advantageous embodiments of the invention form the
subject matters of the dependent claims.
DRAWINGS
[0065] Embodiments of the invention will be described in more
detail below with reference to the drawings.
[0066] FIG. 1 shows a highly schematic diagram in which the
(minimum) total elongation (A.sub.80) is plotted in percent versus
the tensile strength (R.sub.m) in MPa for various steels for the
automotive industry;
[0067] FIG. 2 shows a schematic stress-strain diagram of a steel
product, which has a pronounced yield strength (Luders strain
A.sub.L);
[0068] FIG. 3 shows a schematic diagram showing the two temperature
treatment processes;
[0069] FIG. 4 shows in the form of a schematic diagram the critical
Temperature T.sub.K and the course of the corresponding critical
temperature limit T.sub.KG;
[0070] FIG. 5 shows a schematic diagram, which on the one hand
shows the Luders strain A.sub.L in percent and on the other hand
the average original austenite grain size (D.sub.UAK M) as a
function of the first annealing temperature T1, wherein, in this
diagram, the corresponding curves of two different samples are
shown;
[0071] FIG. 6 shows a schematic diagram showing the tension .sigma.
in MPa as a function of elongation .epsilon. in % (analogous to
FIG. 2), wherein four identical alloys were subjected in this case
to four different temperature treatment processes.
DETAILED DESCRIPTION
[0072] According to the invention, steel products or steel
intermediate products are concerned which are characterized by a
special microstructure constellation and properties.
[0073] In the following, the term "intermediate steel products" is
sometimes used when it is intended to stress that the finished
steel product is not concerned but instead a preliminary or
intermediate product in a multi-stage production process. The
starting point for such manufacturing processes is usually a melt.
In the following, the alloy composition of the melt is given, since
on this side of the manufacturing process, it is possible to
influence the alloy composition relatively accurately (for example,
by adding components such as alloying elements and optional
micro-alloying elements). The alloy composition of the steel
intermediate product usually deviates only insignificantly from the
alloy composition of the melt.
[0074] Quantities or content information are given here largely in
weight percent (in short wt. %), unless stated otherwise. If
information is provided on the composition of the alloy, or the
steel product, respectively, then the composition includes, in
addition to the explicitly listed materials or substances, iron
(Fe) as basic material and so-called unavoidable impurities that
always occur in the molten bath and that also show up in the
resulting steel intermediate product. All statements in wt. % must
always be added to 100 wt. % and all % by volume must always be
added to 100% of the total volume.
[0075] In addition to the special combination of alloying elements,
a specially optimized process for temperature treatment is used. A
corresponding diagram is shown in FIG. 3 and will be explained in
more detail below.
[0076] The temperature treatment of the steel intermediate product
comprises a first temperature treatment process S.1 and a
subsequent second temperature treatment process S.2. These two
temperature treatment processes S.1 and S.2 are shown in FIG. 3 in
two temperature-time diagrams shown next to one another.
[0077] The first temperature treatment process S.1 is a
high-temperature process in which the steel intermediate product is
subjected to a first annealing temperature T1 during a first
holding period .DELTA.1 (this step is also referred to as holding
H1). The annealing temperature T1 lies above a critical temperature
limit T.sub.KG during the holding H1.
[0078] The course of this critical temperature limit T.sub.KG is
dependent (inter alia) on the manganese content Mn of the alloy of
the manganese steel intermediate product, as determined by numerous
examinations. In FIG. 4, the critical temperature T.sub.K
(represented by the straight line 7) and the course of the
corresponding critical temperature limit T.sub.KG (represented by
the straight line 8) are shown.
[0079] On the horizontal axis, the manganese range MnB is plotted
in percent by weight. As already mentioned, the invention gives
excellent results especially with a manganese content in the
following manganese range MnB: 3 wt. %.ltoreq.Mn.ltoreq.12 wt. %.
This manganese range MnB is shown in FIG. 4 by two vertical
boundary lines at Mn=3 wt. % and Mn=12 wt. %.
[0080] FIG. 4 shows by way of example the measurement results of
four samples on the basis of small circle symbols. Further details
on these four exemplary samples and on further samples of the
invention are shown in Tables 1 and 2.
TABLE-US-00001 TABLE 1 Alloy C Mn Al Nb S.1 S.2 Type 1 0.096 5.08
Cont. annealing Hood Type 2 0.097 5.13 0.09 Cont. annealing Hood
Type 3 0.100 6.38 Cont. annealing Hood Type 4 0.106 3.53 Cont.
annealing Hood Type 5 0.110 3.56 0.095 Cont. annealing Hood Type 6
0.148 7.73 2.09 Cont. annealing Cont. annealing Type 7 0.098 9.95
Cont. annealing Hood
TABLE-US-00002 TABLE 2 No Alloy T1, .degree. C. T2, .degree. C.
R.sub.p0.2 or R.sub.eL MPa R.sub.m, MPa A.sub.80, % RA, vol. %
A.sub.L, % D.sub.UAK M, .mu.m 1 Type 1 830 660 550 850 27.1 20.9
2.1 5 2 Type 1 875 660 551 878 28.7 20.1 0 13.7 3 Type 1 900 660
561 890 28.3 21.3 0 15.5 4 Type 1 920 660 561 898 28.5 21.1 0 18 5
Type 1 935 660 559 894 30.2 19.8 0 20 6 Type 1 950 660 522 820 30.7
21.4 0 22 7 Type 1 1100 660 560 852 27.9 21.2 0 45.7 8 Type 2 980
660 632 928 23.4 21.4 2.8 8.5 9 Type 2 1000 660 640 928 23.7 21.9
2.6 9.5 10 Type 2 1025 660 646 931 22.9 22.7 2.2 11.1 11 Type 2
1050 660 643 929 23.4 19.2 2 12 12 Type 2 1075 660 673 962 24.3
19.9 0 13.5 13 Type 2 1100 660 642 898 22.9 22.5 0 14.7 14 Type 3
810 640 635 901 33.3 32.1 2.6 6.4 15 Type 3 850 640 615 903 35.8
32.6 1.8 9.4 16 Type 3 900 640 595 898 36.1 32.2 0 13.8 17 Type 3
950 640 552 893 37 31.8 0 18.9 18 Type 4 840 660 413 559 18.5 6.4
2.2 6 19 Type 4 950 660 391 641 18.5 6.5 1.5 15 20 Type 5 950 630
352 543 19.2 2.7 1.2 7 21 Type 5 1025 630 432 631 17.1 4.9 0 11 22
Type 5 1100 630 623 710 11.8 4.1 0 15 23 Type 6 900 675 829 1083
19.8 20.3 2.3 4.86 24 Type 7 790 630 601 1145 25.2 39.1 2.2 4.8 25
Type 7 900 630 588 1130 28.1 37.2 0 8.8 26 Type 7 950 630 582 1122
29.3 34.6 0 12.9
[0081] The alloy composition of the respective type is shown in
Table 1, wherein only the essential alloying components are
mentioned here. For each type, there are a number of embodiments
that have been tested. The corresponding examples are numbered in
the left column in Table 2 with the numbers 1 to 26.
[0082] In FIG. 4, the following four samples are shown by the
circle symbols mentioned: Type 4, 18; Type 1, 1; Type 3, 14 and
Type 7, 24 (the designation Type 4, 18 represents, for example, the
alloy composition of Type 4, Example No. 18).
[0083] If the circle symbols of FIG. 4, or the measurement results
respectively, are interpolated by a straight line, then a straight
line 7 descending constantly results, as shown in FIG. 4. This
straight line 7 can be circumscribed by the following equation (1),
wherein T.sub.K is given in degrees Celsius:
T.sub.K=(866-S.sub.K*manganese content) (1)
[0084] The absolute value 866 in degrees Celsius defines the
intersection with the vertical axis and the value S.sub.K defines
the slope. S.sub.K is therefore also called the slope value.
[0085] The examinations have shown that the slope value S.sub.K is
preferably 7.83.+-.10% in all embodiments.
[0086] In addition, it could be shown that the critical temperature
T.sub.K for alloy compositions according to the invention always
lies above a lower critical temperature limit T.sub.KG. This lower
critical temperature limit T.sub.KG is shown in FIG. 4 as a
straight line 8.
[0087] This straight line 8 can be circumscribed by the following
equation (2), wherein T.sub.KG is given in degrees Celsius:
T.sub.KG=(856-S.sub.K*manganese content) (2)
[0088] The straight line 8 lies parallel to the straight line
7.
[0089] The following condition can be postulated: For steel alloys
of the manganese steel intermediate product, as already defined,
the first annealing temperature T1 must always be above the lower
critical temperature limit T.sub.KG to ensure that a manganese
steel intermediate product is obtained in which the Luders strain
A.sub.L is less than 3%.
[0090] It could be shown that also the second temperature treatment
process S.2 has an influence on the Luders strain. In order to
maintain the grain size of the austenite grains in the structure,
the second annealing temperature T2 must be lower than the first
annealing temperature T1 in any case. Since the first annealing
temperature T1 is always above the lower critical temperature limit
T.sub.KG, it can be concluded that the second annealing temperature
T2 should preferably be below the lower critical temperature limit
T.sub.KG.
[0091] It can be seen from the schematic example of FIG. 3 that the
first annealing temperature T1 is above the temperature limit
T.sub.KG and that the second annealing temperature T2 is in the
range between A.sub.1 and A.sub.3. The second temperature treatment
S.2 is also referred to in this case as intercritical
annealing.
[0092] The first holding period .DELTA.1 is preferably at least 10
seconds in all embodiments, and preferably between 10 seconds and
6000 minutes.
[0093] The second holding period .DELTA.2 is at least 10 seconds in
all embodiments. In FIG. 3, the two holding periods .DELTA.1 and
.DELTA.2 are shown only by way of example. The interval between the
first temperature treatment process S.1 and the second temperature
treatment process S.2 can be selected as needed. Typically, the
second temperature treatment process S.2 is performed shortly after
the first temperature treatment process S.1.
[0094] Preferred embodiments are those in which the first
temperature treatment process S.1, including the heating E1 of the
steel intermediate product, the holding H1 of the first annealing
temperature T1 and the cooling Ab1 of the steel intermediate
product takes less than 7000 minutes.
[0095] Preferred embodiments are those in which the second
temperature treatment process S.2, including the heating E2 of the
intermediate steel product, the holding H2 of the second annealing
temperature T2 and the cooling Ab2 of the steel intermediate
product takes less than 6000 minutes and preferably less than 5000
minutes.
[0096] Furthermore, it could be shown that the significant
reduction of Luders strain A.sub.L is independent of whether the
first temperature treatment process S.1 and/or the second
temperature treatment process S.2 is/are carried out in a
continuous strip plant (for example in a continuous plant) or in a
discontinuous plant (for example in a hood-type annealer).
[0097] The invention can be applied to both cold strip intermediate
products and hot strip intermediate products. In both cases, a
significant reduction in Luders strain A.sub.L can be seen.
[0098] Increasing the first annealing temperature T1 to a value
above the critical temperature limit T.sub.KG clearly leads to an
increase in the average original austenite grain size and to a
significant reduction in the Luders strain A.sub.L.
[0099] FIG. 5 shows both the reduction of Luders strain A.sub.L in
percent and the dependence of the average original austenite grain
size (D.sub.UAK M) in .mu.m with increasing annealing temperature
T1 for two exemplary samples of Type 1 and Type 2 (see also Table
1), as follows.
[0100] Chemical composition of alloy samples of Type 1 without
microalloying:
Mn=5.08 wt. %,
C=0.096 wt. %,
[0101] the remainder iron Fe and unavoidable impurities.
[0102] Chemical composition of alloy samples of Type 2 with
microalloying:
Mn=5.13 wt. %,
C=0.097 wt. %,
Nb=0.90 wt. %,
[0103] the remainder iron Fe and unavoidable impurities.
[0104] It can be seen from FIG. 5 that in the examined alloy
composition of Type 1 (represented by the curve 9), the critical
temperature limit T.sub.KG is .about.820.degree. C. when it is
desired to obtain a Luders strain for this alloy composition of
Type 1 which is smaller than 3%. The curve 10 shows the associated
course of the average original austenite grain size D.sub.UAK M 1,
as a function of the temperature T1. For the example Type 1, a
grain size for this is obtained with >3 .mu.m.
[0105] It can be seen from FIG. 5 that in the examined alloy
composition of Type 2 (represented by the curve 11), the critical
temperature limit T.sub.KG2 is .about.970.degree. C. when it is
desired to obtain a Luders strain for this alloy composition of
Type 2 which is smaller than 3%. The curve 12 shows the
corresponding curve of the average original austenite grain size
(D.sub.UAK M) as a function of the temperature T1. For the example
Type 2, a grain size for this results with >8 .mu.m. The
micro-alloying element niobium (Nb) has a recognizable influence,
which is expressed as a shift of T.sub.KG2 (compared to T.sub.KG1)
to a higher critical temperature for A.sub.L<3%.
[0106] The curves 10 and 12 in FIG. 5 show that the original
austenite grain size increases with increasing temperature T1.
[0107] On the basis of the above equation (2), for the alloy
compositions of Type 1, the lower temperature limit T.sub.KG1 can
be determined as follows:
T.sub.KG1=(856-7.83*5)=.about.817.degree. C. (2.1)
[0108] In FIG. 5, the corresponding lower temperature limit
T.sub.KG1 is shown as a dashed vertical line. It can be seen that
the alloy compositions of the Type 1 have an average grain size
from an annealing temperature T1>T.sub.KG1 on which is >3
.mu.m. The lower temperature limit T.sub.KG1 is indicated in FIG. 4
by a small black triangle.
[0109] On the basis of the equation (2), for the alloy compositions
of Type 2, the lower critical temperature limit T.sub.KG2 can be
determined as follows:
T.sub.KG2=(856-7.83*5)=.about.817.degree. C.=T.sub.KG1 (2.2)
[0110] For alloy compositions having an Nb content, the micro-alloy
leads to an increase in the critical temperature limit T.sub.KG. In
FIG. 5, it can be seen, using the example of Type 2, that the
critical temperature limit T.sub.KG2 is approx. 150.degree. C.
higher than for the alloy compositions of Type 1. In FIG. 5, the
corresponding effective lower critical temperature limit T*.sub.KG2
is shown as a dashed vertical line. In the case of alloy
compositions of Type 2, the annealing temperature must be
T1>T*.sub.KG2=T.sub.KG2+150.degree. C. The resulting average
original austenitic grain size is .gtoreq.8 .mu.m in this case.
[0111] FIG. 6 shows a schematic diagram indicating the tension
.sigma. in MPa as a function of the elongation .epsilon. in %. The
representation of FIG. 6 is to be compared with the representation
of FIG. 2, wherein FIG. 6 shows only a small section.
[0112] Specifically, four identical samples (Type 3 alloys of Table
1) were compared here. The Type 3 alloys also meet the requirements
of the invention. All four samples were each subjected to a first
temperature treatment process S.1 and a subsequent second
temperature treatment process S.2. All process parameters were
identical, except that in the first temperature treatment process
S.1, the first annealing temperature T1 was varied as follows (see
column 2 of the following Table 3):
TABLE-US-00003 TABLE 3 T1 T2 Alloy [.degree. C.] [.degree. C.]
Curve Type 3 810 640 13.1 Type 3 850 640 13.2 Type 3 900 640 13.3
Type 3 950 640 13.4
[0113] The alloys of Type 3 had the following main composition in
these experiments:
Mn=6.38 wt. %,
C=0.1 wt. %,
[0114] remaining iron Fe and unavoidable impurities.
[0115] The solid curve 13.1 of FIG. 6 (Type 3, 14 of Table 2) shows
a clearly visible pronounced yield strength and has a Luders strain
of A.sub.L.about.2.6%. The temperature T1 here was 810.degree. C.,
which for a Type 3 alloy and a slope value S.sub.K=7.83 is slightly
above the lower critical temperature limit T.sub.KG.
[0116] The curve 13.2 represents another exemplary sample (Type 3,
15 of Table 2) of Type 3, wherein here the yield strength is still
slightly pronounced.
[0117] Another identical sample (see the dash-dotted curve 13.3 in
FIG. 6) was temperature-treated at a higher temperature
T1=900.degree. C. (i.e. at T1>T.sub.KG) and no pronounced yield
strength is visible any more. This concerns Type 3, 16 of Table
2.
[0118] The curve 13.4 represents a further exemplary sample of the
Type 3, wherein in this case too no pronounced yield strength is
visible any more. This concerns Type 3, 17 of Table 2.
[0119] When considering the manganese steel intermediate products
of the invention in connection with FIG. 1, the corresponding
measured values (e.g. for the alloy compositions of Type 1, Type 2
and Type 3) lie in the range of about 700 to 1000 MPa and with a
total elongation A.sub.80 in the range of about 20 to 40%.
TABLE-US-00004 List of reference numerals TRIP steels 1 Q&P and
TBF steels 2 Medium-manganese steels 3 Tensile curve 4 Intermediate
maximum 5 Plateau 6 Straight line 7 Straight line 8 Curve 9 Curve
10 Curve 11 Curve 12 Curves 13.1, 13.2, 13.3, 13.4 Start
temperature of austenitization A.sub.1 Start temperature of full
austenitization A.sub.3 Total elongation A.sub.80 Luders strain
A.sub.L First cooling Ab1 Second cooling Ab2 Average original
austenite grain boundary D.sub.UAK M First holding period .DELTA.1
Second holding period .DELTA.2 First heating E1 Second heating E2
Elongation .epsilon. First holding H1 Second holding H2 Manganese
range MnB Residual austenite content RA Upper yield strength
R.sub.eH Lower yield strength R.sub.eL Tensile strength R.sub.m
0.2% yield strength R.sub.p0.2 First temperature treatment process
S.1 Second temperature treatment process S.2 Tension .sigma. Slope
value S.sub.K First annealing temperature T1 Second annealing
temperature T2 Critical temperature limit T.sub.KG Critical
temperature limit T.sub.KG1 Critical temperature limit T.sub.KG2
Effective critical temperature limit T*.sub.KG2
* * * * *