U.S. patent application number 16/076922 was filed with the patent office on 2019-02-14 for ceramic matrix composite turbine component with graded fiber-reinforced ceramic substrate.
This patent application is currently assigned to Siemens Aktiengesellschaft. The applicant listed for this patent is Siemens Aktiengesellschaft. Invention is credited to Christian Xavier Campbell, Ramesh Subramanian.
Application Number | 20190048730 16/076922 |
Document ID | / |
Family ID | 52589834 |
Filed Date | 2019-02-14 |


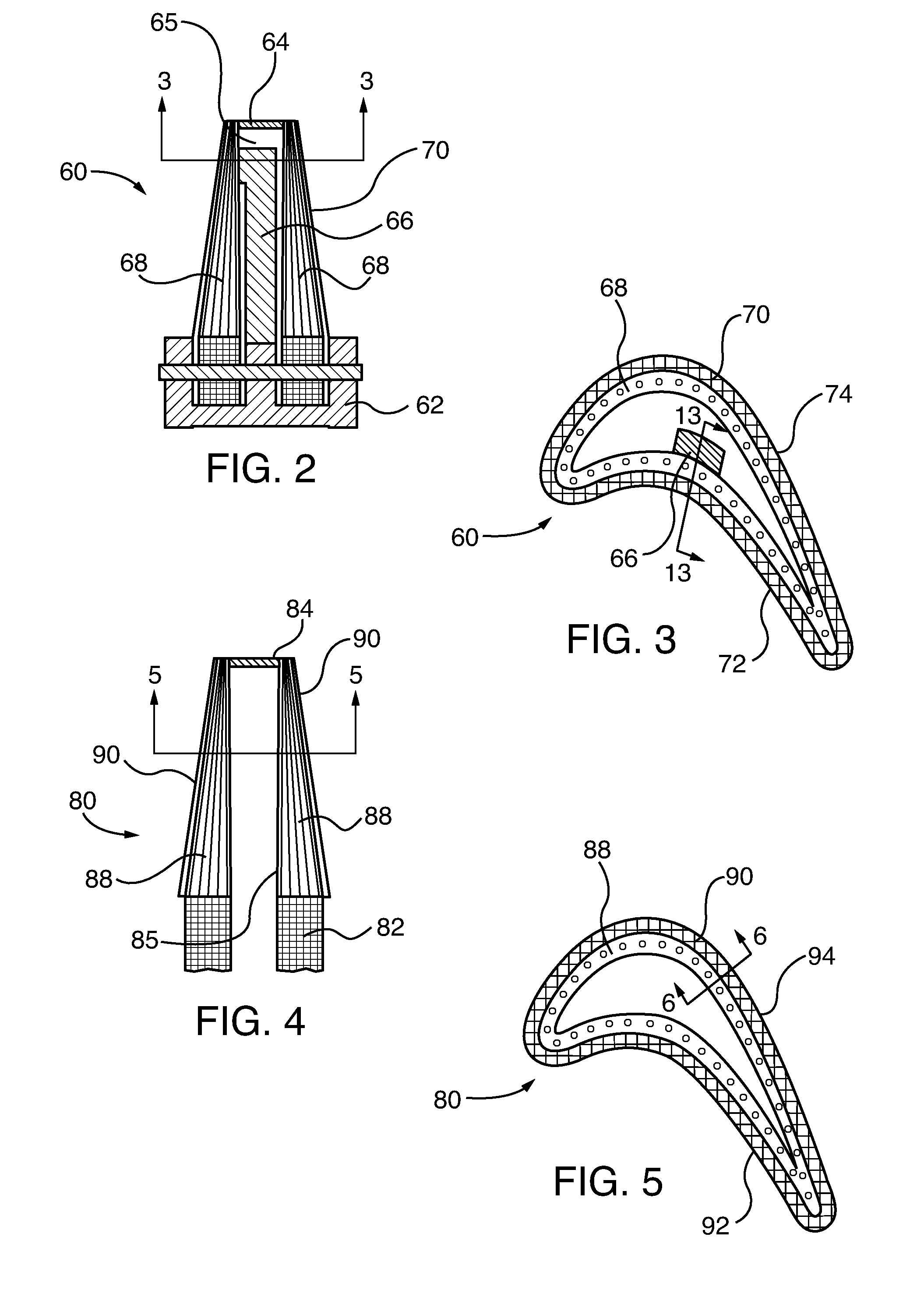


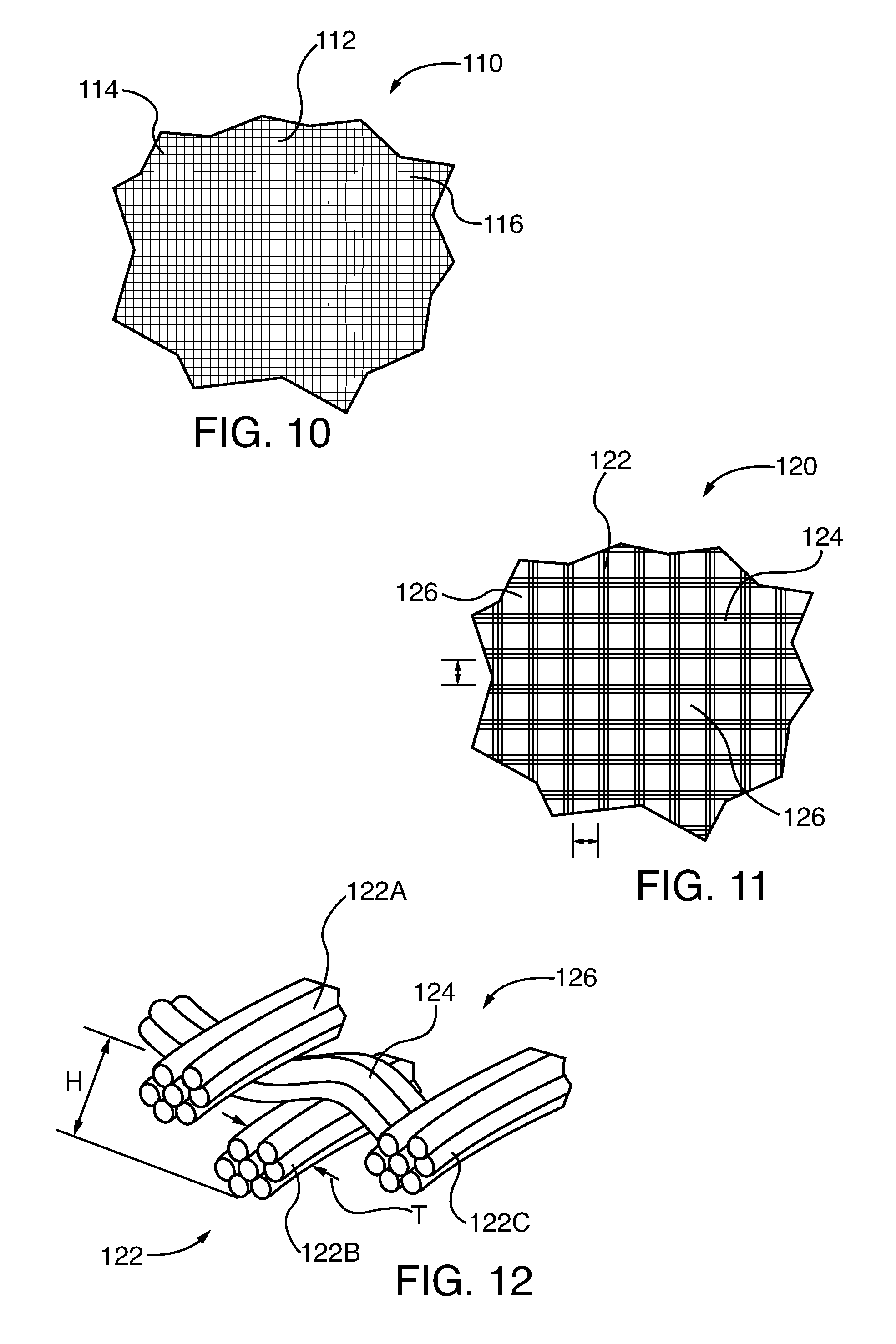

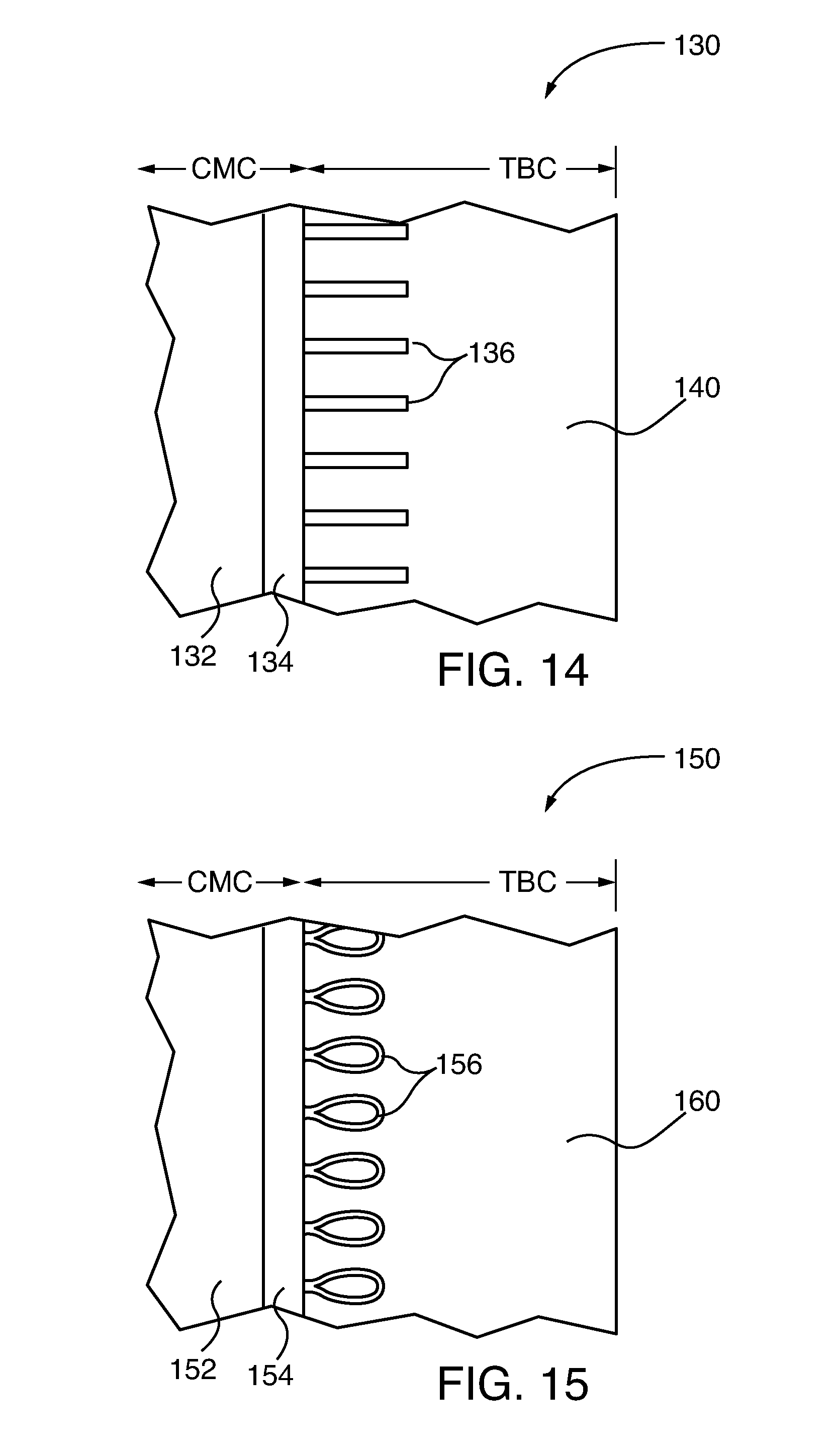
United States Patent
Application |
20190048730 |
Kind Code |
A1 |
Subramanian; Ramesh ; et
al. |
February 14, 2019 |
CERAMIC MATRIX COMPOSITE TURBINE COMPONENT WITH GRADED
FIBER-REINFORCED CERAMIC SUBSTRATE
Abstract
A ceramic matrix composite ("CMC") component, such as a turbine
blade for a combustion turbine engine that has a fiber-reinforced,
solidified ceramic substrate. The substrate has an inner layer of
fibers, for enhancing structural strength of the component. An
outer layer of fibers defines voids therein. A thermal barrier coat
("TBC") is applied over and coupled to the outer layer fibers,
filling the voids. The voids provide increased surface area and
mechanically interlock the TBC, improving adhesion between the
fiber-reinforced ceramic substrate and the TBC.
Inventors: |
Subramanian; Ramesh;
(Oviedo, FL) ; Campbell; Christian Xavier;
(Charlotte, NC) |
|
Applicant: |
Name |
City |
State |
Country |
Type |
Siemens Aktiengesellschaft |
Munchen |
|
DE |
|
|
Assignee: |
; Siemens
Aktiengesellschaft
Munchen
DE
|
Family ID: |
52589834 |
Appl. No.: |
16/076922 |
Filed: |
May 10, 2016 |
PCT Filed: |
May 10, 2016 |
PCT NO: |
PCT/US2016/031607 |
371 Date: |
August 9, 2018 |
Related U.S. Patent Documents
|
|
|
|
|
|
Application
Number |
Filing Date |
Patent Number |
|
|
PCT/US2016/018224 |
Feb 17, 2016 |
|
|
|
16076922 |
|
|
|
|
PCT/US2015/016318 |
Feb 18, 2015 |
|
|
|
PCT/US2016/018224 |
|
|
|
|
Current U.S.
Class: |
1/1 |
Current CPC
Class: |
B22C 7/02 20130101; F05D
2220/32 20130101; F05D 2240/11 20130101; F05D 2250/185 20130101;
F01D 5/284 20130101; F05D 2230/00 20130101; F05D 2250/23 20130101;
F04D 29/526 20130101; F01D 11/08 20130101; F01D 5/187 20130101;
F05D 2250/182 20130101; F05D 2250/60 20130101; F05D 2260/202
20130101; C23C 4/073 20160101; B28B 11/048 20130101; F01D 11/127
20130101; F04D 29/685 20130101; F05D 2250/183 20130101; F05D
2250/12 20130101; F05D 2250/75 20130101; F01D 9/023 20130101; Y02T
50/676 20130101; Y02T 50/6765 20180501; F05D 2250/282 20130101;
F01D 5/28 20130101; Y02T 50/672 20130101; F05D 2250/184 20130101;
F05D 2260/22141 20130101; C23C 4/18 20130101; F01D 5/18 20130101;
F01D 5/186 20130101; F01D 25/12 20130101; C23C 28/3215 20130101;
F01D 5/288 20130101; F05D 2230/30 20130101; F05D 2300/175 20130101;
B22D 25/02 20130101; B28B 19/0015 20130101; F05D 2250/15 20130101;
B22D 29/001 20130101; F05D 2230/90 20130101; F05D 2250/294
20130101; Y02T 50/60 20130101; F05D 2230/211 20130101; F05D 2230/31
20130101; F05D 2250/292 20130101; Y02T 50/673 20130101; C23C 4/02
20130101; B22C 9/24 20130101; C23C 28/3455 20130101; F01D 11/122
20130101 |
International
Class: |
F01D 5/28 20060101
F01D005/28; B28B 11/04 20060101 B28B011/04; B28B 19/00 20060101
B28B019/00 |
Claims
1. A ceramic matrix composite ("CMC") component for a combustion
turbine engine, comprising: a fiber-reinforced ceramic substrate,
having: an inner layer fibers, for enhancing structural strength of
the component, and an outer layer of fibers outboard of the inner
layer, the outer layer comprising voids therein defined by a
spacing distance between adjacent fibers of the outer layer; and a
thermally sprayed or vapor deposited or solution/suspension plasma
sprayed thermal barrier coat ("TBC"), including a TBC inner surface
applied over and coupled to the outer layer fibers, filling the
voids there between, and a TBC outer surface for exposure to
combustion gas.
2. The engine component of claim 1, the outer layer fibers defining
a textured surface profile, having height variation greater than
the diameter of any single fiber, or bundle of fibers therein, for
increasing contact surface area with the TBC inner surface.
3. The engine component of claim 2, further comprising fiber
strands projecting outwardly away from an outer surface of a
pattern that is defined by the outer layer fibers, for increasing
contact surface area with the TBC inner surface.
4. The engine component of claim 2, further comprising fiber strand
loops projecting outwardly away from an outer surface of a pattern
that is defined by the outer layer fibers, for increasing contact
surface area with the TBC inner surface.
5. The engine component of claim 2, the TBC outer surface having
engineered groove features ("EGFs").
6. The engine component of claim 1, the TBC thickness between 0.5
mm to 2 mm.
7. The engine component of claim 2, a textured surface profile
height in the outer fiber layer varying between 0.1 to 1.5 mm, with
the voids in the outer fiber layer defined by a spacing distance
between individual fibers or fiber bundles of 0.1 mm to 8 mm.
8. The engine component of claim 2, further comprising an
intermediate layer of fibers interposed between the inner and outer
fiber layers, having a fiber pattern that defines a density and
cross sectional area less than those of the inner fiber layer
pattern, and greater than those of the outer fiber layer.
9. The engine component of claim 1, further comprising a metallic
member coupled to fiber-reinforced ceramic substrate, and
circumscribed by the inner layer of fibers.
10. The engine component of claim 1, further comprising an
intermediate layer of fibers interposed between the inner and outer
fiber layers, having a fiber pattern that defines a density and
cross sectional area less than those of the inner fiber layer
pattern, and greater than those of the outer fiber layer.
11. The engine component of claim 1, the fibers in the
fiber-reinforced ceramic substrate comprising: oxide ceramic
fibers, glass or glassy fibers, or non-oxide ceramic fibers.
12. The engine component of claim 11, the fibers in the
fiber-reinforced ceramic substrate selected from the group
consisting of alumina, mullite, mixtures of alumina and mullite,
and silicon carbon nitride ("SiCN").
13. A method for manufacturing a ceramic matrix composite ("CMC")
component for a combustion turbine engine, comprising: laying-up
ceramic fibers into a layered structure, including: an inner layer,
for enhancing structural strength of the component, and an outer
layer of fibers outboard of the inner layer, the outer layer
comprising voids therein defined by a spacing distance between
adjacent fibers of the outer layer; and; impregnating the ceramic
fibers with ceramic slurry material, if those fibers were not
previously impregnated with ceramic material prior to their lay-up;
curing the impregnated ceramic fibers, forming a solidified
fiber-reinforced ceramic substrate, which defines a ceramic
substrate outer surface; and applying a thermally sprayed, or vapor
deposited, or solution/suspension plasma sprayed thermal barrier
coat ("TBC") over and coupled to the ceramic substrate outer
surface.
14. The method of claim 13, further comprising laying-up the outer
layer fibers to define a textured surface profile, having height
variation greater than the diameter of any single fiber, or bundle
of fibers therein, for increasing contact surface area with the TBC
inner surface.
15. The method of claim 14, further comprising laying-up the outer
layer fibers to define fiber strands projecting outwardly away from
an outer surface of a pattern that is defined by the outer layer
fibers, for increasing contact surface area with the TBC inner
surface.
16. The method of claim 14, further comprising laying-up the outer
layer fibers to define fiber strand loops projecting outwardly away
from an outer surface of a pattern that is defined by the outer
layer fibers, for increasing contact surface area with the TBC
inner surface.
17. The method of claim 14, further comprising applying the TBC to
a thickness between 0.5 mm to 2 mm.
18. The method of claim 14, further comprising laying-up the
textured surface profile in the outer fiber layer with a height
between 0.1 mm to 1.5 mm, and with voids in the outer fiber layer
defined by a spacing distance between individual fibers or fiber
bundles of 0.1 mm to 8 mm.
19. The method of claim 14, further comprising laying-up an
intermediate layer of fibers interposed between the inner and outer
fiber layers, having a fiber pattern that defines a density and
cross sectional area less than those of the inner fiber layer
pattern, and greater than those of the outer fiber layer.
20. The method of claim 13, further comprising laying-up an
intermediate layer of fibers interposed between the inner and outer
fiber layers, having a fiber pattern that defines a density and
cross sectional area less than those of the inner fiber layer
pattern, and greater than those of the outer fiber layer.
Description
PRIORITY CLAIM
[0001] This application claims priority to International
Application No. PCT/US16/18224, filed Feb. 17 2016, and entitled
"CERAMIC MATRIX COMPOSITE TURBINE COMPONENT WITH ENGINEERED SURFACE
FEATURES RETAINING A THERMAL BARRIER COAT", which claims priority
to and the benefit of International Application No. PCT/US15/16318,
filed Feb. 18, 2015, and entitled "TURBINE COMPONENT THERMAL
BARRIER COATING WITH CRACK ISOLATING ENGINEERED GROOVE FEATURES",
the entire contents of which are incorporated by reference
herein.
TECHNICAL FIELD
[0002] The invention relates to components for combustion turbine
engines, with ceramic matrix composite ("CMC") structures that are
in turn insulated by a thermal barrier coating ("TBC"), and methods
for making such components. More particularly, the invention
relates to engine components for combustion turbines, with ceramic
matrix composite ("CMC") structures, having graded fiber-reinforced
ceramic substrates. An inner layer fiber pattern provides
structural support for the component and an outer layer fiber
pattern anchors the TBC to the CMC structure.
BACKGROUND
[0003] CMC structures comprise a solidified ceramic substrate, in
which are embedded ceramic fibers. The embedded ceramic fibers
within the ceramic substrate of the CMC improve elongation rupture
resistance, fracture toughness, thermal shock resistance, and
dynamic load capabilities, compared to ceramic structures that do
not incorporate the embedded fibers. The CMC embedded fiber
orientation also facilitates selective anisotropic alteration of
the component's structural properties. CMC structures are
fabricated by laying-up or otherwise orienting ceramic fibers, also
known as "rovings", into fabrics, filament windings, tows, or
braids. Fiber-reinforced ceramic substrate fabrication for CMCs is
comparable to what is done to form fiber-reinforced polymer
structural components for aircraft wings or boat hulls. Unless the
ceramic fibers are pre-impregnated with a resin containing ceramic
material, they are subsequently impregnated with ceramic material
by such techniques as gas deposition, melt infiltration, preceramic
polymer pyrolysis, chemical reactions, sintering, or
electrophoretic deposition of ceramic powders, creating a solid
ceramic structure with embedded, oriented ceramic fibers.
[0004] Ceramic matrix composite ("CMC") structures are being
incorporated into gas turbine engine components as insulation
layers and/or structural elements of such components, such as
insulating sleeves, vanes and turbine blades. These CMCs provide
better oxidation resistance, and higher temperature capability, in
the range of approximately 1150 degrees Celsius ("C") for oxide
based ceramic matrix composites, and up to around 1350 C for
Silicon Carbide fiber-Silicon Carbide core ("SiC--SiC") based
ceramic matrix composites, whereas nickel or cobalt based
superalloys are generally limited to approximately 950 to 1000
degrees Celsius under similar operating conditions within engines.
While 1150 C (1350 C for SiC--SiC based CMCs) operating capability
is an improvement over traditional superalloy temperature limits,
mechanical strength (e.g., load bearing capacity) of CMCs is also
limited by grain growth and reaction processes with the matrix
and/or the environment at 1150 C/1350 C and higher. With desired
combustion turbine engine firing temperatures as high as 1600-1700
C, the CMCs need additional thermal insulation protection
interposed between themselves and the combustion gasses, to
maintain their temperature below 1150 C/1350 C.
[0005] CMCs are receiving additional thermal insulation protection
by application of overlayer(s) of thermal barrier coats or coatings
("TBCs"), as has been done in the past with superalloy components.
However, TBC application over CMC or superalloy substrates presents
new and different thermal expansion mismatch and adhesion
challenge. During gas turbine engine operation superalloy, CMC and
TBC materials all have different thermal expansion properties. In
the case of TBC application over superalloy substrates, the
superalloy material expands more than the overlying TBC material,
which in extreme cases leads to crack formation in the TBC layer
and its delamination from the superalloy surface. Along with
thermal mismatch challenges, metallic substrate/TBC interfaces have
adhesion challenges. While TBC material generally adheres well to a
fresh metallic superalloy substrate, or in an overlying metallic
bond coat ("BC") substrate, the metals generate oxide surface
layers, which subsequently degrade adhesion to the TBC at the
respective layer interface.
[0006] TBC/metallic substrate interface integrity is maintained by
use of the inventions in International Application No.
PCT/US15/16318, entitled "TURBINE COMPONENT THERMAL BARRIER COATING
WITH CRACK ISOLATING ENGINEERED GROOVE FEATURES"; and International
Application No. PCT/US15/16331, entitled "TURBINE COMPONENT THERMAL
BARRIER COATING WITH CRACK ISOLATING ENGINEERED SURFACE FEATURES",
both of which are in the priority chain of this application and
which are incorporated by reference during National Phase
prosecution in those jurisdictions that allow such incorporation.
Some embodiments described in these priority applications
incorporate engineered surface features ("ESFs") on the substrate
surface of the metallic superalloy substrate, or in an overlying
metallic bond coat ("BC"), or a combination in both metallic
surfaces. The ESFs at the metal surface/TBC layer interface
mechanically anchor the TBC material, to inhibit delamination or at
least confine delamination damage to boundaries defined by adjacent
ESFs. Other embodiments in the priority applications incorporate
engineered groove features ("EGFs") on the TBC layer outer surface,
to control surface crack propagation. Additional embodiments in
those applications incorporate both ESFs and EGFs. Therefore, as
the metal material is heated (forming surface oxides) and expands
during engine operation, the lesser expanding TBC material is
mechanically interlocked with the metal, despite degradation of
interlayer adhesion.
[0007] Turning back to CMC/TBC thermal expansion mismatch and
general interlayer adhesion challenges, relative layer expansion is
opposite that experienced by superalloy/TBC components. The TBC
material tends to expand more than underlying CMC material. As the
TBC heats, it tends to lose adhesion with and delaminate from the
CMC surface. Many CMC materials already contain oxides in the
solidified ceramic core and in their embedded ceramic fibers, which
adversely affect inter-layer adhesion at the CMC/TBC interface. In
the case of SiC--SiC composites, the thermal barrier coatings can
react with the underlying Silicon based matrix to form new chemical
compounds, more brittle than the matrix or coating. Therefore,
application of the TBC on the CMC surface of the component without
subsequent delamination during engine operation is difficult.
Depending upon the local macro roughness of the embedded ceramic
fibers in the preform, and the infiltration characteristics of the
ceramic material, which embed the preform into the solidified
ceramic core, the adhesion of TBC coatings, is generally poorer
than that of TBC coating on metallic substrates. TBC/CMC adhesion
is particularly poor where the ceramic substrate's embedded fibers
are oriented parallel to the component surface. TBC layer thickness
is limited to that which will maintain adhesion to the CMC surface,
despite its higher rate of thermal expansion. In other words, TBC
layer thickness is kept below a threshold that accelerates the
TBC/CMC thermal expansion delamination, within the already
relatively limited bounds of TBC/CMC material adhesion
capabilities. Unfortunately, limiting the TBC layer thickness
undesirably limits its insulation properties. Generally, a thicker
TBC layer offers more insulation protection to the underlying CMC
substrate/layer than a thinner layer.
SUMMARY OF INVENTION
[0008] Exemplary embodiments described herein enhance TBC retention
on CMC components in combustion turbine engines, by utilizing
graded fiber or graded patterned fabric embedded in different zones
within the CMC ceramic substrate. Inner fibers, in the more
inwardly facing zone of the ceramic substrate provide greater
structural strength of the component, than the outer fibers along
the outer surface of the substrate, which interface with the TBC
layer's inner surface. The outer fiber patterns have anchoring
voids between fibers and/or fiber bundles for retention and
anchoring of the TBC layer as the latter is applied to the ceramic
core. In some embodiments, the outer fiber patterns have textured
surfaces, including in some embodiments three-dimensional textured
surfaces, for anchoring of the TBC layer within peaks and valley
voids formed in the fabric weave. Other embodiments include fiber
strands and/or fiber loops that project from the outer fabric weave
pattern, for additional TBC layer anchoring. The outer fabric
weaves voids and/or textured surface features mechanically
interlock the CMC structure, and in particular, the fibers, to the
TBC, and provide increased surface area and additional interlocking
for interlayer adhesion. Optionally, as described in the
incorporated by reference priority document, PCT/US16/18224, filed
Feb. 17 2016, and entitled "CERAMIC MATRIX COMPOSITE TURBINE
COMPONENT WITH ENGINEERED SURFACE FEATURES RETAINING A THERMAL
BARRIER COAT", engineered surface features ("ESFs") are cut into
the outer surface ceramic core and fibers of the preform. A
thermally sprayed or vapor deposited or solution/suspension plasma
sprayed TBC is applied over and coupled to the ceramic substrate
outer surface and any cut ESFs. Increased adherence capabilities
afforded by the outer fiber pattern voids and/or projections
facilitate application of thicker TBC layers to the component,
which increases insulation protection for the underlying CMC
structure/layer. The increased adhesion surface area and added
mechanical interlocking of the respective materials facilitates
application of greater TBC layer thickness to the CMC substrate
without risk of TBC delamination. The greater TBC layer thickness
in turn provides more thermal insulation to the CMC structure, for
higher potential engine operating temperatures and efficiency. In
some embodiments, the CMC component covers an underlying substrate,
such as a superalloy metallic substrate. In other embodiments, the
CMC component is a sleeve over a metallic substrate. In other
embodiments, the CMC component has no underlying metallic
substrate, and provides its own internal structural support within
the fiber-reinforced ceramic substrate. In additional embodiments,
a plurality of CMC components are joined together to form a larger,
composite CMC component, such as a laminated turbine blade or vane.
In other embodiments, the CMC component is a unistructural,
non-laminated, turbine blade, or vane.
[0009] The CMC component is made by laying-up ceramic fibers into a
layered structure, having an inner layer, for structural support,
and an outer layer, for TBC anchoring. If the layed-up fabric
structure is not already pre-impregnated with ceramic material
prior to laying them up, non-impregnated fibers are subsequently
infiltrated with ceramic material, forming a solidified ceramic
core. The TBC is then applied to the core outer surface. The outer
fabric layer voids and projections assist in anchoring the TBC
layer to the ceramic substrate's outer surface, in order to resist
the aforementioned oxide layer and thermal expansion induced
delamination challenges inherent in CMC/TBC components for gas
turbine engines.
[0010] Exemplary embodiments feature a ceramic matrix composite
("CMC") component for a combustion turbine engine, which has a
solidified ceramic substrate, with ceramic fibers, embedded
therein. The fiber-reinforced ceramic substrate has an inner layer
of fibers, for enhancing structural strength of the component. The
substrate also has an outer layer of fibers outboard of the inner
layer, which defines voids therein. A thermally sprayed, or vapor
deposited, or solution/suspension plasma sprayed thermal barrier
coat ("TBC") is applied over and coupled to the substrate's outer
layer fibers, filling the voids. The voids provide increased
surface area and mechanically interlock the TBC, improving adhesion
between the fiber-reinforced ceramic substrate and the TBC. The TBC
outer surface is suitable for combustion gas exposure when
installed in an operating gas turbine engine.
[0011] Other exemplary embodiments feature a component for a
combustion turbine engine, which component includes a metallic
member for structural support. The metallic member is circumscribed
by a ceramic matrix composite ("CMC"), such that the inner layer of
fibers also circumscribe the metallic member. In some embodiments,
the CMC layer also functions as a support substrate, with or
without an additional metallic member. The CMC layer includes the
solidified ceramic fiber-reinforced substrate, with a substrate
inner surface that is shaped to conform to and abut the metallic
member's surface profile. In some embodiments, engineered surface
features ("ESFs") are cut into the ceramic substrate's outer
surface and its outer layer fibers. A thermally sprayed or vapor
deposited or solution/suspension plasma sprayed thermal barrier
coat ("TBC"), including a TBC inner surface, is applied over and
coupled to the ceramic substrate's outer surface, anchored by voids
in the outer layer fibers. In some embodiments, the outer layer
fibers define a textured, surface profile, having height variation
greater than the diameter of any single fiber, or bundle of fibers
therein, for increasing contact surface area with the TBC inner
surface. In some embodiments, fiber strands or fiber strand loops
project outwardly from the second layer, for increasing contact
surface area with the TBC inner surface. In other embodiments, an
intermediate layer of fibers is interposed between the inner and
outer fiber layers. In some embodiments, the intermediate layer has
a pattern that defines a third density and cross sectional area
less than those of the first layer do, and greater than those of
the second layer.
[0012] Other exemplary embodiments feature methods for
manufacturing a CMC component for a combustion turbine engine. A
three-dimensional preform is fabricated with ceramic fibers.
Ceramic fibers are layed-up into a layered structure, which
includes an inner layer, for structural strength of the component.
The layered structure also has an outer layer of fibers outboard of
the inner layer, having a second weave pattern that defines voids
therein. In some embodiments, the inner and outer layer fibers are
pre-impregnated with ceramic material prior to being layed-up into
the layered structure. If not already pre-impregnated with ceramic
material, the layered structure is infiltrated with ceramic
material, forming a solidified, fiber-reinforced ceramic substrate,
which defines a substrate outer surface. A thermally sprayed, or
vapor deposited, or solution/suspension plasma sprayed thermal
barrier coat ("TBC") is applied over and coupled to the substrate
outer surface and its outer layer fibers, filling the voids. The
voids provide increased surface area and mechanically interlock the
TBC, improving adhesion between the ceramic substrate and the TBC.
The TBC outer surface is suitable for combustion gas exposure when
installed in an operating gas turbine engine. In some method
embodiments, the outer layer fibers have a textured surface
profile, having height variation greater than the diameter of any
single fiber, or bundle of fibers therein, for increasing contact
surface area with the TBC inner surface. In other embodiments, the
outer layer fibers have outwardly projecting fiber strands or fiber
loops, for increasing contact surface area with the TBC inner
surface. In yet other embodiments, the fiber-reinforced ceramic
substrate is fabricated with an intermediate layer of fibers
interposed between the inner and outer fiber layers, having a third
weave pattern that defines a third weave density and cross
sectional area less than those of the inner layer weave pattern,
and greater than those of the outer layer weave pattern.
[0013] The respective features of the exemplary embodiments of the
invention that are described herein may be applied jointly or
severally in any combination or sub-combination.
BRIEF DESCRIPTION OF DRAWINGS
[0014] The exemplary embodiments are further described in the
following detailed description in conjunction with the accompanying
drawings, in which:
[0015] FIG. 1 is a partial axial, cross sectional view of a gas or
combustion turbine engine, incorporating one or more CMC components
constructed in accordance with exemplary embodiments of the
invention;
[0016] FIG. 2 is a cross sectional elevation view of a metal
reinforced, CMC turbine blade component for a combustion turbine
engine, in accordance with an exemplary embodiment of the
invention;
[0017] FIG. 3 is a cross sectional plan view of the turbine blade
of FIG. 2;
[0018] FIG. 4 is a cross sectional elevation view of another
embodiment of a CMC turbine blade component for a combustion
turbine engine, which does not have internal metal
reinforcement;
[0019] FIG. 5 is a cross sectional plan view of the turbine blade
of FIG. 4;
[0020] FIG. 6 is a partial cross sectional elevation view through
the side wall of the turbine blade of FIGS. 4 and 5, showing the
graded fiber layers that are embedded within the solidified,
fiber-reinforced ceramic substrate;
[0021] FIG. 7 is an elevational view of inner layer fibers of the
blade side wall of FIG. 6, prior to their incorporation into the
fiber-reinforced ceramic substrate, or application of a TBC;
[0022] FIG. 8 is an elevational view of an alternate embodiment of
the inner layer fibers of the blade side wall of FIG. 6, prior to
their incorporation into the fiber-reinforced ceramic substrate, or
application of a TBC;
[0023] FIG. 9 is a detailed elevational view of the inner layer
fibers of FIG. 8;
[0024] FIG. 10 is an elevational view of intermediate layer fibers
of the blade side wall of FIG. 6, prior to their incorporation into
the fiber-reinforced ceramic substrate, or application of a
TBC;
[0025] FIG. 11 is an elevational view of outer layer fibers of the
blade side wall of FIG. 6, prior to their incorporation into the
fiber-reinforced ceramic substrate, or application of a TBC;
[0026] FIG. 12 is a detailed elevational view of the outer layer
fibers of FIG. 11;
[0027] FIG. 13 is a partial cross sectional elevation view through
the side wall of the turbine blade of FIGS. 2 and 3, showing the
blade's metallic support member, and the graded fibers that
embedded within the fiber-reinforced ceramic substrate;
[0028] FIG. 14 is a partial cross sectional elevation view through
the side wall of another embodiment of turbine blade, showing fiber
strands projecting outwardly from the outer layer fibers, for
anchoring the TBC; and
[0029] FIG. 15 is a partial cross sectional elevation view through
the side wall of another embodiment of turbine blade, showing fiber
loops projecting outwardly from the outer layer fibers, for
anchoring the TBC.
[0030] To facilitate understanding, identical reference numerals
have been used, where possible, to designate identical elements
that are common to the figures. The figures are not drawn to
scale.
DESCRIPTION OF EMBODIMENTS
[0031] Exemplary embodiments herein are utilized in combustion
turbine engines. Embodiments of the CMC components of the invention
are combined to form composite structures, such as turbine blades
or vanes, which are structurally self-supporting. In other
embodiments, the CMC components cover other structural elements,
such as internal metallic (e.g., superalloy metal) members,
including by way of example structural reinforcement ribs or other
types of supports. In some embodiments, the ceramic matrix
composite ("CMC") components of the invention are utilized as
insulative covers or sleeves for other structural components, such
as metallic superalloy components or other types of metallic
support members. In other embodiments, the CMC component is
entirely structurally self-supporting, relying on internally
embedded fibers to provide additional strength to its
fiber-reinforced, ceramic substrate. Embodiments of the CMC
components of the invention have a solidified, fiber-reinforced
ceramic substrate, with ceramic fibers embedded therein. The
fiber-reinforced ceramic substrate utilizes a graded fiber or
graded patterned fabric embedded in different zones within the CMC
substrate. Inner fibers in the more inwardly facing zone of the
ceramic substrate have relatively higher fiber density and cross
section, for greater structural support of the component, than the
outer fibers along the outer surface of the core, which interface
with the TBC layer's inner surface. The outer fiber patterns have
voids between fibers and/or fiber bundles for retention and
anchoring of the TBC layer as the latter is applied to the
fiber-reinforced ceramic substrate. In some embodiments, the outer
fiber patterns have textured surfaces, including in other
embodiments textured three-dimensional surfaces, for anchoring of
the TBC layer within peaks and valley voids, or fiber-spacing voids
formed in the fabric pattern or weave. Other embodiments include
fiber strands and/or fiber loops that project from the outer fabric
pattern or weave (including by further example knitted fabric
weaves), for additional TBC layer anchoring. The outer fabric voids
and surface features mechanically interlock the CMC structure, and
in particular, the fibers, to the TBC, and provide increased
surface area and additional interlocking for interlayer adhesion.
In some embodiments, engineered surface features ("ESFs") are cut
into an outer surface of the fiber-reinforced ceramic substrate. A
thermally sprayed or vapor deposited or solution/suspension plasma
sprayed TBC is applied over and coupled to the fiber-reinforced
ceramic substrate's outer surface and any cut ESFs.
[0032] The outer fabric layer voids and surface features provide
increased surface area, and mechanically interlock the TBC,
improving adhesion between the ceramic fiber-reinforced ceramic
substrate and the TBC. The mechanical interlocking and improved
adhesion afforded by the voids and surface features within the
outer fabric layer facilitate application of relatively thick TBC
layers, from 0.5 mm to 2.0 mm. Because of the thick TBC
application, embodiments of the CMC components of the invention are
capable of operation in combustion environments up to 1950 degrees
Celsius, with the thick TBC limiting the CMC ceramic core
temperature to below 1150/1350 degrees Celsius.
[0033] In accordance with method embodiments of the invention, the
CMC component is made by laying-up ceramic fibers into a layered
structure. If the ceramic fibers are not already pre-impregnated
with ceramic material prior to their laying-up, they are
subsequently infiltrated with ceramic material, forming a
solidified, fiber-reinforced ceramic substrate. In some
embodiments, engineered surface features ("ESFs") are cut into the
ceramic substrate's outer surface and its outer layer fibers. The
TBC is then applied to the ceramic substrate's outer surface and
any ESFs. If the CMC component is structurally self-supporting, the
ceramic substrate's inner fabric layer provides structural support
to the component, such as a blade or vane of a gas turbine engine.
If the CMC component is an insulative cover for another structural
component, such as a metallic member, superalloy substrate, the
component is dimensioned to cover, or otherwise circumscribe, the
metallic member. In some applications, the CMC component or a
plurality of CMC components are configured as insulative sleeves to
cover the metallic member. In some embodiments, a plurality of such
sleeves are stacked and laterally joined over a metallic member or
other metallic substrate, prior to TBC application. In other
embodiments, the CMC component is a unistructural, self-supporting
blade, or vane for a gas turbine engine.
[0034] FIG. 1 shows a gas turbine engine 20, having a gas turbine
casing 22, a multi-stage compressor section 24, a combustion
section 26, a multi-stage turbine section 28 and a rotor 30. One of
a plurality of basket-type combustors 32 is coupled to a downstream
transition 34 that directs combustion gasses from the combustor to
the turbine section 28. Atmospheric pressure intake air is drawn
into the compressor section 24 generally in the direction of the
flow arrows F along the axial length of the turbine engine 20. The
intake air is progressively pressurized in the compressor section
24 by rows rotating compressor blades 50 and directed by mating
compressor vanes 52 to the combustion section 26, where it is mixed
with fuel and ignited. The ignited fuel/air mixture, now under
greater pressure and velocity than the original intake air, is
directed through a transition 34 to the sequential vane 56 and
blade 50 rows in the turbine section 28. The engine's rotor 30 and
shaft retains the plurality of rows of airfoil cross sectional
shaped turbine blades 54. Embodiments of the CMC components
described herein are designed to operate in engine temperature
environments of up to 1950 degrees Celsius. In some embodiments,
the CMC components are insulative sleeves or coverings for metallic
members, including metallic-substrate structural components, such
as the subcomponents within the combustors 32, the transitions 34,
the blades 54, or the vanes 56. In other embodiments, the CMC
components of the invention are structurally self-supporting,
without the need for metallic members or other supporting metallic
substrates. Exemplary self-supporting CMC components include
compressor blades 50 or vanes 52 (which do not necessarily require
the insulation of a TBC, internal subcomponents of combustors 32 or
transitions 34). In some embodiments, entire turbine section 28
blades 54 or vane 56 airfoils are CMC structures; with outer
surfaces of their fiber-reinforced ceramic substrates having
surface textures, through use of graded fabrics, that mechanically
interlock a relatively thick TBC layer of 0.5 to 2.0 mm.
[0035] A schematic cross section of an exemplary engine component,
a turbine blade 60, is shown in FIGS. 2 and 3. The turbine blade 60
has a blade root 62 for affixation to the turbine engine's rotor 30
and a distal blade tip 64. The blade internal side wall 65 includes
a metallic member, which is a metallic reinforcement rib 66. The
rib 66 is covered or otherwise circumscribed by a CMC,
fiber-reinforced ceramic substrate 68. Internal structural details
of the internal core within the turbine blade 60 are known, and for
brevity are not shown or described in detail. The ceramic substrate
68 includes graded ceramic fibers embedded therein, which are
described in detail below. A thermally sprayed or vapor deposited
or solution/suspension plasma sprayed thermal barrier coat ("TBC")
70 is applied over and coupled to the substrate 68 outer surface
about the blade side wall pressure side 72 and its suction side 74,
including the leading and trailing edges.
[0036] The turbine blade 80 embodiment of FIGS. 4 and 5 has a blade
root 82, blade tip 84, and a self-supporting, CMC fiber-reinforced
ceramic substrate 88, which form the blade airfoil side wall,
including the internal side wall 85 and an outer portion onto which
is applied a TBC 90. The turbine blade 80 has no internal metallic
ribs or other support structure to carry loads imparted on the
blade's pressure side 92 or suction side 94. The self-supporting
CMC, fiber-reinforced ceramic substrate 88 utilizes graded fibers
for performing localized structural functions. Some fibers are
primarily incorporated for structural strength within the CMC
ceramic substrate, while others are primarily incorporated for
anchoring the TBC layer 90. In this embodiment, the fibers forming
the layered structure of the CMC fiber-reinforced ceramic substrate
88 are incorporated into sheets of fabric, which in some
embodiments are stacked in layers or plies, and wrapped about the
blade, i.e., outboard of the boundaries of the internal side wall
85, during blade fabrication, analogous to a cigar wrapper.
Generally, fiber orientation within the fiber structures, which are
embedded within fiber-reinforced ceramic substrate 88, incorporate
one or more of woven fibers (two- or three-dimensional weaves,
including knit weaves), braided fibers (including tows of braided
fibers), and/or uniaxial fibers.
[0037] The graded fabric plies, which are incorporated into the
layered structure of the fiber-reinforced ceramic substrate 88, are
shown schematically in FIG. 6. The description that follows with
respect to FIG. 6 is also applicable to FIG. 13. The schematic of
FIG. 13, directed to the blade embodiment of FIGS. 2 and 3,
incorporates the metal member, blade-reinforcing rib 66, but is
otherwise identical to FIG. 6. For simplicity, a plane view of each
exemplary type of fabric sheet within the ceramic core 88 is shown
in FIG. 6.
[0038] Referring to FIGS. 6 and 7, an inner layer of parallel
fibers, such as the woven fabric fibers shown therein, have a first
weave pattern 100 that defines a first weave density and cross
sectional area, providing structural strength to the component
ceramic substrate 88 of the blade component 80. The primary
function of the inner layer fibers is to provide structural support
to the ceramic substrate 88 and the overall blade component 80. The
shown inner layer weave pattern has parallel vertical or axially
oriented fibers 102 that are woven in bundles or tows 112, which
enhance axial and torsional tensile strength of the blade component
80. The inner layer weave pattern 100 also has horizontal fibers
104, which maintain parallel orientation of the
vertical/axially-oriented fibers 102. The vertical fibers 102 are
tightly packed in the horizontal direction of FIG. 7, with no
intentional spacing between them. In the alternative embodiment of
FIGS. 8 and 9, the inner layer fabric 100A has a tight, flat weave
fiber pattern, with an equal distribution and density of vertical
fibers 102A and horizontal fibers 104A. Both the embodiments 100
and 100A for the inner layer fabric weave have relatively flat
surface profiles. As previously noted, such flat weave profiles do
not promote good bonding with TBC overlays, leaving the TBC
susceptible to separation from the fiber-reinforced ceramic
substrate 88 during thermal expansion or from relatively poor oxide
bonding between the ceramic substrate 88 and the TBC material 90.
Therefore, the embodiments 100 and 100A of the inner layer fiber
weave are less than optimal for use in the outermost layer of a
fiber-reinforced ceramic substrate, such as the turbine blade
ceramic substrate 88. However, the embodiments described herein
incorporate graded fibers in the fiber-reinforced ceramic substrate
88, which vary orientation locally within the substrate, and which
are chosen for their structural and functional suitability.
[0039] Referring to FIGS. 6 and 10, the fiber-reinforced ceramic
substrate 88 incorporates an intermediate layer of fibers, such as
the woven fiber fabric 110, which are outboard of the inner woven
fiber layer(s) 100. The intermediate layer 110 has a weave pattern
that defines a weave density and cross sectional area, less than
those of the first weave pattern 100 do. The intermediate layer
comprises vertical fibers (one or more fibers alone or in
bundles/tows) 112, with lateral spacing distance S2, and horizontal
fibers 114 (one or more fibers alone or in bundles/tows), with
vertical spacing S1. Voids 116 are formed between the spaced fibers
112 and 114, facilitating bonding and structural integrity within
the CMC ceramic core 88.
[0040] As shown in FIGS. 6, 11 and 12, an outer layer of fibers,
such as the fabric woven fibers 120 is oriented in the CMC
component's fiber-reinforced ceramic substrate 88, outboard of the
inner layer 100 and the intermediate layer 110. In some
embodiments, scrim fabric, which generally comprises an open fiber
grid of non-woven, fused fibers, is used to form the outer layer
fibers 120. In the embodiments of FIGS. 6, 11 and 12, the outer
layer fibers 120 have a weave pattern that defines a weave density
and cross sectional area less than those of the inner layer weave
pattern 100 or the intermediate layer weave pattern 110. This outer
layer 120 employs a textured surface, with a weave pattern of
vertical fibers 122 (one or more fibers alone or in bundles/tows,
or a non-woven, scrim-type fabric), with lateral spacing distance
S4, and horizontal fibers 124 (one or more fibers alone or in
bundles/tows, or in scrim fabric fused fibers), with vertical
spacing S3. In some embodiments, the spacing S3 or S4 between
fibers is between 0.1 mm to 8 mm. Voids 126 are formed between the
net-like array of spaced fibers 122 and 124, facilitating bonding
and structural integrity within the CMC ceramic core 88 and the TBC
layer 90.
[0041] As shown in the embodiment of FIG. 12, the
three-dimensional, textured weave also creates vertical gaps or
voids 126 of radial distance or height H, between the fiber strands
122A, 122B, and 122C, in addition to the net-like voids 126 formed
between fibers in the open spacing distances S3 and S4. In some
embodiments, vertical voids in the outer fiber layer defined by
spacing between individual fibers or fiber bundles of textured
surface profile height H in the outer fiber layer 120, varies
between 0.1 to 1.5 mm. In this embodiment, the height H within the
outer fiber layer 120 is greater than diameter T of any single
fiber, or bundle of fibers that form the layer. The vertical and
horizontal voids or gaps create spaces for embedding and anchoring
of the TBC, as it is applied to the CMC component's
fiber-reinforced ceramic substrate 88. Given the lesser weave
density and cross sectional area of the outer fabric layer 120, as
compared to the inner layer 110, the former provides less
structural strength to the CMC turbine blade component 80 and its
ceramic substrate 88, but it provides considerably more surface
area and voids for anchoring of the TBC layer 90.
[0042] A thermally sprayed or vapor deposited or
solution/suspension plasma sprayed thermal barrier coat ("TBC") 90
is applied over and coupled to the CMC ceramic substrate 88 outer
surface and its outer fabric layer 120. The TBC 90 bonds and
anchors to the outer fabric layer 120, with its relatively large
surface area along the bonding zone, compared to a relatively flat
planar bonding zone, which would otherwise be formed by the weave
pattern of the inner layer fabric 110. Experience has shown that
TBC tends to delaminate and spall from a flat CMC outer surface,
especially if the reinforcing fibers, such as those of the inner
fabric layer 110, are oriented parallel to the CMC ceramic
substrate 88 outer surface. In embodiments herein, the voids or
interstices 126, including the exemplary three-dimensional voids
and interstices, skew orientation of the fibers 122 and 124
relative to the TBC layer 90, which creates abutting interfaces,
rather than the parallel interfaces of the inner fabric layer 110.
Optional engineered groove features (EGFs) 91 are cut into the TBC
outer surface.
[0043] Referring to the component embodiments of FIGS. 14 and 15,
TBC adhesion to the CMC component's fiber-reinforced ceramic
substrate is enhanced by bonding between the TBC material and
fibers that project from the outer fabric layer outer surface. In
FIG. 14, the component 130 CMC component's fiber-reinforced ceramic
substrate 132 has an outer layer fiber pattern 134, with fiber
strands 136 projecting from the outer layer. Cutting ceramic fiber
strands in non-structural load bearing outer layer fabric 134
peripheral zones of the CMC component's ceramic substrate 132 does
not impair structural integrity of the CMC component 130. The fiber
strands 136 provide additional anchoring for the TBC layer 140.
[0044] Referring to FIGS. 6 and 13, optional engineered groove
features ("EGFs") 71, 91 are cut into the respective TBC 70, 90
outer surfaces, as described in the incorporated by reference
priority International Application No. PCT/US16/18224, filed Feb.
17 2016, and entitled "CERAMIC MATRIX COMPOSITE TURBINE COMPONENT
WITH ENGINEERED SURFACE FEATURES RETAINING A THERMAL BARRIER COAT".
In some embodiments, as described in the priority documents, the
EGFs 71, 91 are cut in pattern arrays, including pattern arrays
that intersect engineered surface features ("ESFs") of the CMC
ceramic core, for enhanced spallation isolation. In embodiments of
the priority document, the CMC ceramic substrate outer surfaces and
embedded ceramic fibers are cut by milling arrays of dimple- or
cylindrical-shaped ESFs into them. Other profile ESFs are
optionally formed by selectively varying the ceramic substrate's
outer profiles, symmetrically or asymmetrically.
[0045] Exemplary methods for manufacturing a ceramic matrix
composite ("CMC") component for a combustion turbine engine are now
described. Such components include the oxide fiber-oxide ceramic
core CMC components 60, 80, 130 and 150 of FIGS. 6 and 13-15, Using
any known technique, graded ceramic fibers are layed-up into a
layered structure. Exemplary layered structures are layed-up by
orienting ceramic fibers into symmetrical or asymmetrical patterns.
In some embodiments the fibers are already incorporated into a two-
or three-dimensional fabric weave, or various fabric bundles, or
within non-woven scrim fabric, ready to be layed-up into the
layered structure. In some embodiments, the fiber pattern is
selectively varied to provide anisotropic structural properties,
for example if the finished CMC component is to function as a
self-supporting or partially self-supporting structural element, as
opposed to a non-structural insulative cover over a metallic member
or another substrate.
[0046] The graded fiber layers in the CMC component are selected to
vary locally structural strength, as well as to enhance impregnated
ceramic slurry material or TBC anchoring capabilities. The layered
fabric's surface texture (e.g., within a two- or three-dimensional
weave pattern fabric or non-woven scrim fabric) can be selectively
varied during its laying-up or prior to the lay-up by selecting
fabrics with desired fiber patterns. In some embodiments, the
layed-up fiber surface texture is varied through application of
different scrim fabric fiber spacing and/or fiber thickness, or
weave/tow patterns within woven fabrics. This allows selective
alteration of fiber orientation and anisotropic structural strength
in some layers or zones within the fiber-reinforced ceramic
substrate, and for future bonding with an applied TBC in other
fabric layers or zones within the ceramic substrate. For example,
in some embodiments, the fabric layers within the layed-up layered
structure can be varied to accommodate future cut ESF orientation
between fiber bundles or outwardly jutting projections in the
completed fiber-reinforced ceramic substrate.
[0047] In some embodiments, the fiber-reinforced ceramic substrate
88, within the CMC-composite turbine blade 80, is made from: (i)
oxide ceramic fibers (e.g., yttrium aluminum garnet ("YAG") fibers
commercially available under the trademarks NEXTEL.RTM. 440,
NEXTEL.RTM. 610, and NEXTEL.RTM. 720), or alternatively, zirconium
oxide ("ZrO.sub.2"); (ii) glass or glassy fibers (e.g.,
commercially available under the trademarks NEXTEL.RTM. 312,
Fiberglass, E-glass); or (iii) non-oxide ceramic fibers (silicon
carbide ("SiC"), or alternatively, silicon carbon nitride
("SiCN")). Oxide ceramic fiber composites are typically formed
using oxide ceramic slurry, such as alumina, mullite, zirconia, or
zirconia toughened alumina ("ZTA"). Glass fiber composites
typically have a glassy matrix. Non-oxide fiber ceramics (typically
SiC, commercially available under trademarks SYLRAMIC.RTM.,
HI-NICALON.RTM., TYRANO.RTM.) are formed using a non-oxide ceramic
matrix (SiC, SiCN) from ceramic powders, ceramic precursors
(silicon polyborosilazane), chemical vapor infiltration, or melt
infiltrated processing.
[0048] In some embodiments, the fibers used to lay-up the layered
structure that will be incorporated into the fiber-reinforced
ceramic substrate 88 are pre-impregnated with ceramic material
("pre-preg" fiber or fabrics). After the pre-preg lay-up is
completed, it is cured into the solidified and hardened
fiber-reinforced ceramic substrate 88, which is in turn processed
into the final CMC component, such as the turbine blade 80. If
pre-preg fiber material is not utilized, it is layed-up into a
layered structure, which is subsequently impregnated with ceramic
material prior to curing, solidification and hardening into the
fiber-reinforced ceramic substrate 88. Exemplary ceramic materials
used to impregnate the layered structure, for subsequent
solidification into the fiber-reinforced ceramic substrate 88,
include alumina silicate, alumina zirconia, alumina, yttria
stabilized zirconia, silicon, or silicon carbide polymer
precursors. The post lay-up infiltration is performed, by any known
technique, including gas deposition, melt infiltration, chemical
vapor infiltration, slurry infiltration, preceramic polymer
pyrolysis, chemical reactions, sintering, or electrophoretic
deposition of ceramic powders, creating a solid, fiber-reinforced
ceramic structure with embedded, graded ceramic fiber layers 100,
120, and in some embodiments 110.
[0049] Optional engineered surface features ("ESFs") are cut into
the outer surface of the fiber-reinforced ceramic substrate, and
into its embedded fibers 120, with any known cutting technique,
including mechanical machining, ablation by laser or electric
discharge machining, grid blasting, or high pressure fluid. While
general CMC fabrication generally disfavors cutting fibers within a
preform, for fear of structural weakening, cutting fibers proximate
the outer surface of the fiber-reinforced ceramic substrate, such
as those incorporated within the CMC components 60, 80, 130, and
150 of FIGS. 6 and 13-15, have not structurally weakened those
components. The optional ESFs are mechanically cut by milling, or
by laser ablation, of the outer surface of the ceramic substrate
that is within the CMC components 60, 80, 130, and 150.
[0050] A known composition, thermally sprayed, or vapor deposited,
or solution/suspension plasma sprayed thermal barrier coat ("TBC")
is applied over the fiber-reinforced ceramic substrate 88.
Exemplary TBC compositions include single layers of 8-weight
percent yttria stabilized zirconia ("8YSZ"), or 20-weight percent
yttria stabilized zirconia ("20YSZ"). For pyrochlore containing
thermal barrier coatings, an underlayer of 8YSZ is required to form
a bilayer 8YSZ/59 weight percent gadolinium stabilized zirconia
(8YSZ/59GZO) coating, or a bilayer 8YSZ/30-50 weight percent yttria
stabilized zirconia ("30-50 YSZ") coating, or combinations thereof.
The TBC adheres to the outer surface of the ceramic substrate,
including the outer layer fibers 120 and any optional ESFs. The
outer layer fibers 120 and any optional ESFs increase surface area
for TBC to ceramic substrate adhesion, and provide mechanical
interlocking of the materials. Cut ceramic fiber ends along sides
of the optional ESFs, as well as the fiber strands 136 of the CMC
component 130 of FIG. 14 or the fiber loops 156 of the CMC
component 160 adhere to and abut the TBC material, further
increasing adhesion strength. Optionally, a rough surface ceramic
bond coat is applied over the fiber-reinforced ceramic substrate
outer surface, including its outer layer fabric and any optional
ESFs, by a known deposition process, further enhancing adhesion of
the TBC layer to the ceramic substrate. In exemplary embodiments,
the bond coat material is alumina or YAG, to enable oxidation
protection, in case of complete TBC spallation from the ceramic
substrate outer surface.
[0051] Increased ceramic substrate/TBC adhesion, attributable to
increased adhesion surface area, mechanical interlocking, and
exposed outer layer ceramic fiber/TBC adhesion facilitate
application of thicker TBC layers in the range of 0.5 mm to 2.00
mm, which would otherwise potentially delaminate from a comparable
flat surface TBC/ceramic substrate interface. Thicker TBC increases
insulation protection to the underlying ceramic substrate and
fibers of the CMC component, such as a blade or vane for a
combustion turbine engine. Exemplary simulated turbine component
structures, fabricated in accordance with embodiments described
herein, withstand TBC outer layer exposure to 1950 degrees Celsius
combustion temperatures, while maintaining the underlying
fiber-reinforced ceramic substrate and its embedded fiber layers
temperatures below 1150 degrees/1350 degrees Celsius. As previously
discussed, exposure of the underlying fiber-reinforced ceramic
substrate and its embedded fiber layers within CMC components to
temperatures above 1150 C/1350 C, within a combustion turbine
engine, thermally degrade those components.
[0052] Although various embodiments that incorporate the invention
have been shown and described in detail herein, others can readily
devise many other varied embodiments that still incorporate the
claimed invention. The invention is not limited in its application
to the exemplary embodiment details of construction and the
arrangement of components set forth in the description or
illustrated in the drawings. The invention is capable of other
embodiments and of being practiced or of being carried out in
various ways. In addition, it is to be understood that the
phraseology and terminology used herein is for the purpose of
description and should not be regarded as limiting. The use of
"including," "comprising," or "having" and variations thereof
herein is meant to encompass the items listed thereafter and
equivalents thereof as well as additional items. Unless specified
or limited otherwise, the terms "mounted", "connected",
"supported", and "coupled", and variations thereof are used broadly
and encompass direct and indirect mountings, connections, supports,
and couplings. Further, "connected" and "coupled" are not
restricted to physical, mechanical, or electrical connections or
couplings.
* * * * *