U.S. patent number 10,525,081 [Application Number 15/664,305] was granted by the patent office on 2020-01-07 for progressively tumorigenic cell lines.
This patent grant is currently assigned to Grove City College. The grantee listed for this patent is Grove City College. Invention is credited to David Jones, Durwood B. Ray.

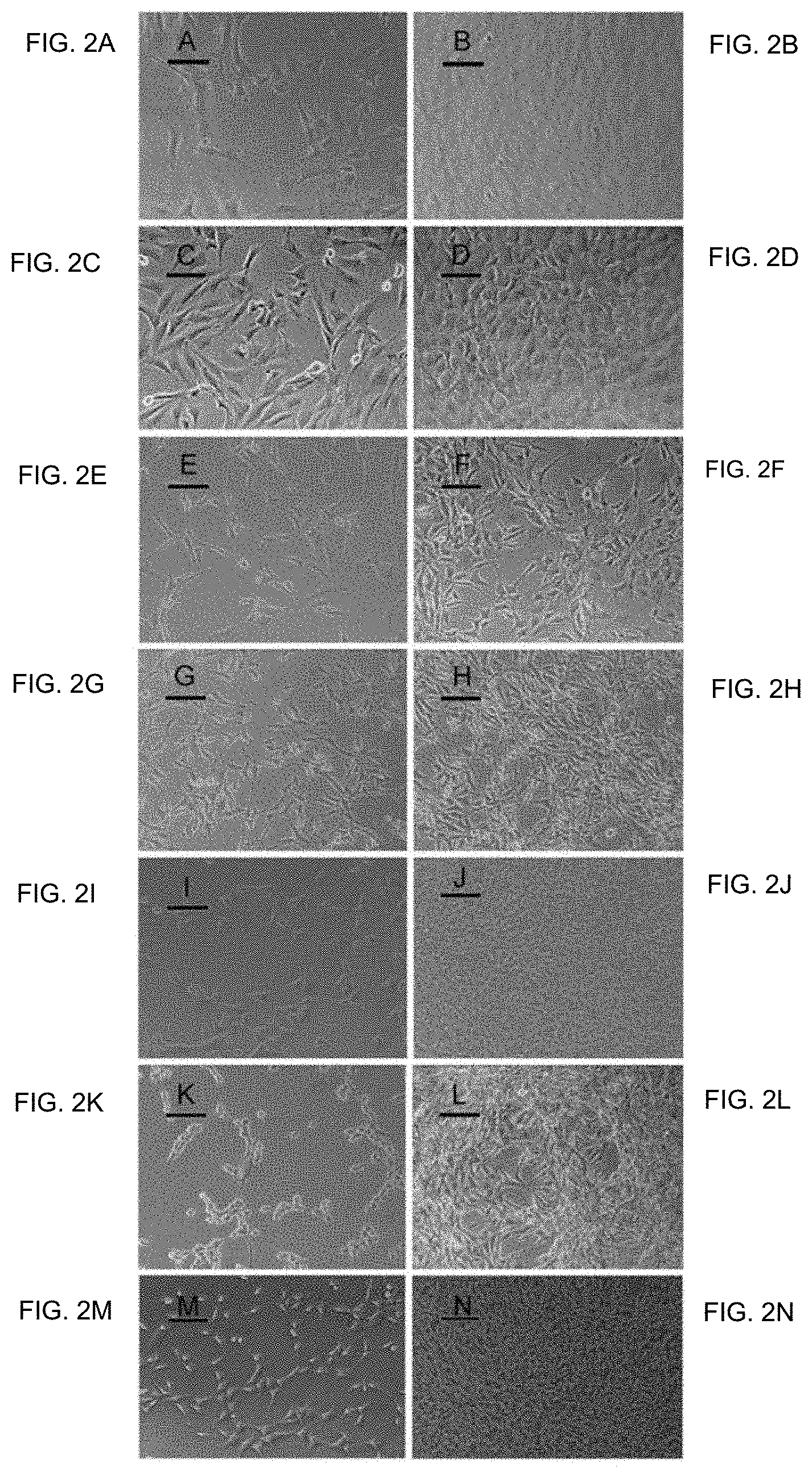
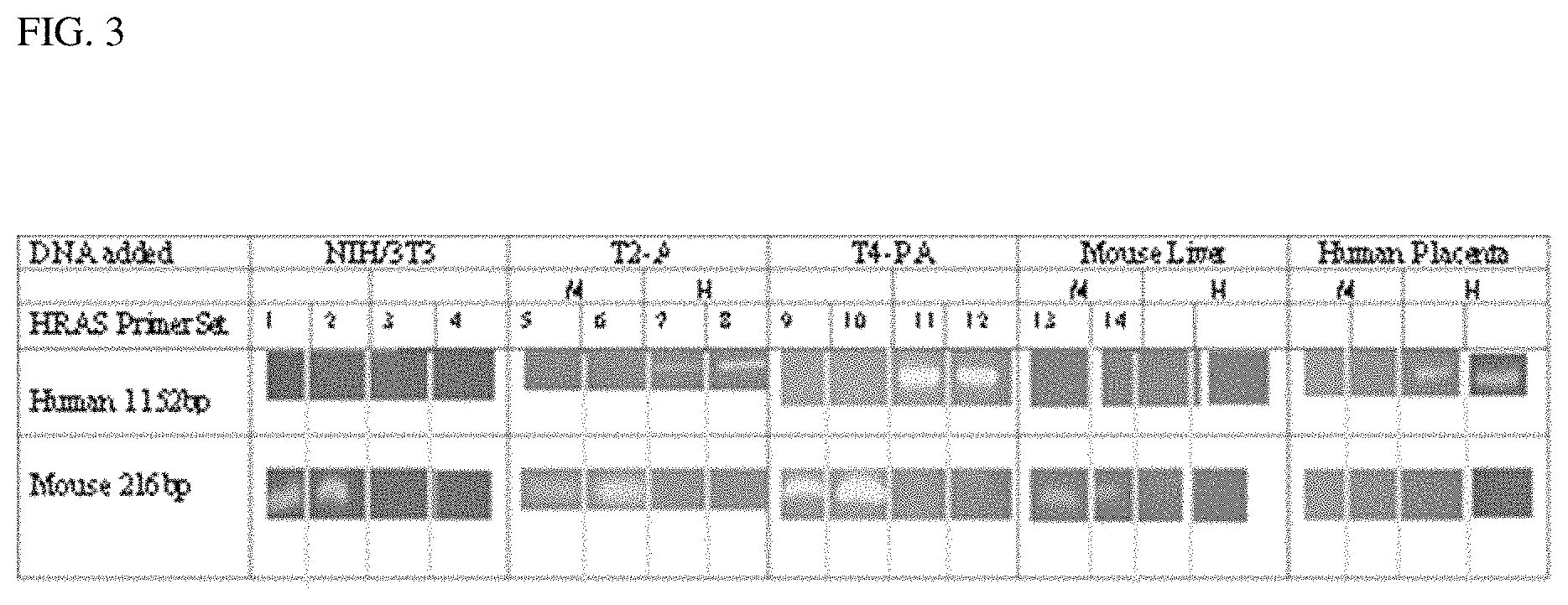
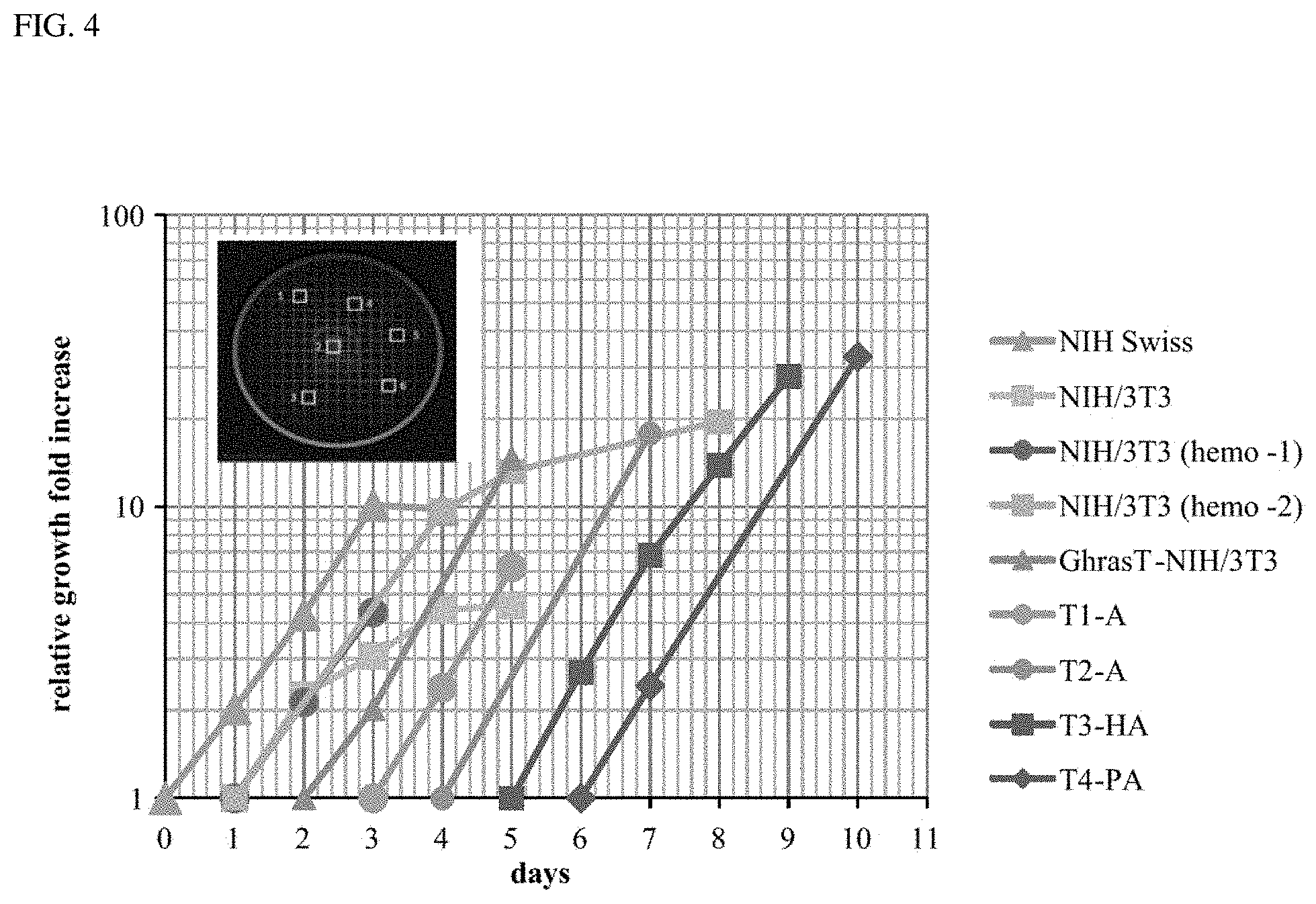



United States Patent |
10,525,081 |
Ray , et al. |
January 7, 2020 |
Progressively tumorigenic cell lines
Abstract
Provided herein, inter alia, are tumorigenic cell lines and
methods for using the same for deriving a series of cell culture
and non-human animal-based models which exhibit increasingly
aggressive tumorigenic potential as well as use of the same for
evaluating the anti-neoplastic properties of candidate therapeutic
agents.
Inventors: |
Ray; Durwood B. (Grove City,
PA), Jones; David (Grove City, PA) |
Applicant: |
Name |
City |
State |
Country |
Type |
Grove City College |
Grove City |
PA |
US |
|
|
Assignee: |
Grove City College (Grove City,
PA)
|
Family
ID: |
61558978 |
Appl.
No.: |
15/664,305 |
Filed: |
July 31, 2017 |
Prior Publication Data
|
|
|
|
Document
Identifier |
Publication Date |
|
US 20180071338 A1 |
Mar 15, 2018 |
|
Related U.S. Patent Documents
|
|
|
|
|
|
|
Application
Number |
Filing Date |
Patent Number |
Issue Date |
|
|
62369638 |
Aug 1, 2016 |
|
|
|
|
Current U.S.
Class: |
1/1 |
Current CPC
Class: |
G01N
33/5044 (20130101); A01K 67/0271 (20130101); C12N
5/0693 (20130101); A61K 35/13 (20130101); C12N
2510/00 (20130101); A01K 2227/105 (20130101); A01K
2207/12 (20130101); C12N 2501/998 (20130101); A01K
2267/0331 (20130101) |
Current International
Class: |
A61K
35/13 (20150101); G01N 33/50 (20060101); C12N
5/09 (20100101); A01K 67/027 (20060101) |
Other References
Navale (2013) "Animal Models of Cancer: A Review", International
Journal of Pharmaceutical Sciences and Research, 4(1): 19-28.
(Year: 2013). cited by examiner.
|
Primary Examiner: Kelly; Robert M
Attorney, Agent or Firm: Mintz, Levin, Cohn, Ferris, Glovsky
and Popeo P.C.
Government Interests
STATEMENT AS TO RIGHTS TO INVENTIONS MADE UNDER FEDERALLY SPONSORED
RESEARCH AND DEVELOPMENT
This invention was made with government support under Grant
#8602787 awarded by the National Science Foundation. The government
has certain rights in the present invention.
Parent Case Text
CROSS-REFERENCE TO RELATED APPLICATIONS
This application claims priority to U.S. provisional patent
application Ser. No. 62/369,638, filed on Aug. 1, 2016, the content
of which is incorporated by reference herein in its entirety.
Claims
We claim:
1. A mouse cell line designated T3-HA, deposited under the ATCC
accession number PTA-123513.
2. A mouse comprising a cell of the cell line T3-HA of claim 1,
wherein the cell is injected into the mouse and develops into a
metastatic liver or lung tumor in the mouse.
3. The mouse of claim 2, wherein the mouse is
immunocompromised.
4. The mouse of claim 3, wherein the mouse is a nude NIH Swiss
mouse.
5. A mouse cell line designated T4-PA, deposited under the ATCC
accession number PTA-123514.
6. A mouse comprising a cell of the cell line T4-PA of claim 5,
wherein the cell is injected into the mouse and develops into more
than one distant metastatic tumors in more than one organs or
extremities in the mouse.
7. The mouse of claim 6, wherein the injected cell develops into
more than one distantly metastatic tumors within 21-24days of
injection.
8. The mouse of claim 6, wherein the mouse is
immunocompromised.
9. The mouse of claim 8, wherein the mouse is a nude NIH Swiss
mouse.
10. A method for producing a series of mice with increasingly
aggressive tumors, the method compromising: a. injecting at least
one cell from a cell line having all of the characteristics of the
cell line designated T1-A, deposited under ATCC accession number
PTA-123697-subcutaneously into a first mouse, wherein the first
mouse develops a primary tumor; b. injecting at least one cell from
the T1-A cell line of (a) intravenously into a second mouse,
wherein the second mouse develops a locally metastatic tumor; c.
injecting at least one cell from a cell line having all of the
characteristics of the cell line designated T2A, deposited under
ATCC accession number PTA-123698-intravenously into a third mouse,
wherein the third mouse develops a distantly metastatic tumor; d.
injecting at least one cell from a cell line having all of the
characteristics of the cell line designated T3-PA, deposited under
ATCC accession number PTA-123699 intravenously into a fourth mouse,
wherein the fourth mouse develops a metastatic lung tumor; e.
injecting at least one cell from a cell line having all of the
characteristics of the cell line designated T3-HA, deposited under
ATCC accession number PTA-123513 into a fifth mouse, wherein the
fifth mouse develops a metastatic liver or lung tumor; and f.
injecting at least one cell from a cell line having all of the
characteristics of the cell line designated T4-PA, deposited under
ATCC accession number PTA-123514-intravenously into a sixth mouse,
wherein the sixth mouse develops more than one distant metastatic
tumors in more than one organs or extremities, thereby producing a
series of mice with increasingly aggressive tumors.
11. A mouse cell line designated T1-A, deposited under the ATCC
accession number PTA-123697.
12. A mouse cell line designated T2-A, deposited under the ATCC
accession number PTA-123698.
13. A mouse cell line designated T3-PA, deposited under the ATCC
accession number PTA-123699.
Description
SUBMISSION OF SEQUENCE LISTING ON ASCII TEXT FILE
The Sequence Listing submitted in an ASCII text file, in accordance
with 37 C.F.R. .sctn. 1.821(c) and (e), is incorporated herein by
reference. The text file name is
051004_501001US_Sequence_Lisiting_11062017.TXT, the date of
creation of the text file is Nov. 13, 2017, and the size of the
ASCII text file is 904 bytes.
FIELD OF THE INVENTION
Provided herein, inter alia, are tumorigenic cell lines and methods
for using the same for deriving a series of cell culture and
non-human animal-based models which exhibit increasingly aggressive
tumorigenic potential as well as use of the same for evaluating the
anti-neoplastic properties of candidate therapeutic agents.
BACKGROUND OF THE INVENTION
Cancer remains a pressing medical and public health problem, in
spite of decades of research into the molecular and genetic
mechanisms underlying the development of this disease. It is well
established that human cancers develop in a multistep sequence with
environmental influences including chemical, physical, and viral
agents being major etiological contributors. Numerous transitions
occur in a "tumorigenic progression" as cells change from "normal"
to "immortalized" to "transformed" and finally to the "metastatic
state." These changes that produce tumors all occur in a variety of
in vivo microenvironments. Recent studies indicate that cancer
cells release exosomes that migrate ahead and attach at distant
locations and somehow prepare these microenvironments for the
progressing tumorigenic cells to settle into as they arrive to
establish a metastatic tumor. The classical textbook view of a
gradual accumulation of genomic alterations in this progression has
been enlightened using next generation whole genome sequencing
(WGS) and spectral karyotyping (SKY) with the recent description of
stochastic cancer genome fragment rearrangements.
Current studies of transformation and tumor progression often rely
on the use of primary embryonic cells or cell lines as a model to
evaluate the myriad effects of various treatments on cells
progressing from normal to pre-malignant to malignant and even
metastatic potential. However, model systems that provide for the
ability to evaluate the anti-neoplastic potential of candidate
therapeutic agents against a series of increasingly aggressive
tumors which are derived sequentially from a single progenitor cell
type are needed. As the progressively aggressive tumors produced by
this model would be derived from the same progenitor cell type in
vivo in a variety of actual non-human animal microenvironments,
such a model would more closely recapitulate the normal transitions
observed in human cancers as they progress in vivo from localized
tumors into a more virulent metastatic state. The ability to
preserve and use these cells from these tumors for further
characterization and studies of anti-neoplastic agents illustrates
the uniqueness of this series of cells that have been generated by
this novel serially extended in vivo/in vitro approach.
Throughout this specification, various patents, patent applications
and other types of publications (e.g., journal articles, electronic
database entries, etc.) are referenced. The disclosure of all
patents, patent applications, and other publications cited herein
are hereby incorporated by reference in their entirety for all
purposes.
BRIEF SUMMARY OF THE INVENTION
Provided herein, inter alia, are tumorigenic cell lines and methods
of using the same for producing cell culture and non-human
animal-based model systems with increasingly aggressive tumors for
evaluating the anti-neoplastic potential of candidate
therapeutics.
Accordingly, in some aspects, provided herein is a T1-A cell line
designated ATCC PTA-123697 or a cell line having all of the
identifying characteristics of ATCC PTA-123697. In some
embodiments, the cell line develops into a tumor when a cell from
the cell line is injected into a non-human animal. In some
embodiments, the cell line develops into a tumor when a cell from
the cell line is injected into a non-human animal. In some
embodiments, the tumor develops within 7-10 days of injection of
the cell from the cell line into the non-human animal. In some
embodiments, the cell line develops into a locally metastatic tumor
when a cell from the cell line is injected into a non-human animal.
In some embodiments, the locally metastatic tumor develops within
13 days of injection of the cell from the cell line into the
non-human animal.
In other aspects, provided herein is a T2-A cell line designated
ATCC PTA-123698 or a cell line having all of the identifying
characteristics of ATCC PTA-123698. In some embodiments, the cell
line develops into a distantly metastatic tumor in at least one
organ or tissue when a cell from the cell line is injected into a
non-human animal. In some embodiments, the cell line develops into
a distantly metastatic tumor within 21-27 days of injection of the
cell from the cell line into the non-human animal.
In further aspects, provided herein is a T3-PA cell line designated
ATCC PTA-123699 or a cell line having all of the identifying
characteristics of ATCC PTA-123699. In some embodiments, the cell
line develops into a metastatic lung tumor when a cell from the
cell line is injected into a non-human animal.
In other aspects, provided herein is a T3-HA cell line designated
ATCC PTA-123513 or a cell line having all of the identifying
characteristics of ATCC PTA-123513. In some embodiments, the cell
line develops into a metastatic liver or lung tumor when a cell
from the cell line is injected into a non-human animal.
In another aspect, provided herein is a T4-PA cell line designated
ATCC PTA-123514 or a cell line having all of the identifying
characteristics of ATCC PTA-123514. In some embodiments, the cell
line develops into more than one distant metastatic tumors in more
than one organs or extremities when a cell from the cell line is
injected into a non-human animal. In some embodiments, the cell
line develops into more than one distantly metastatic tumors within
21-24 days of injection of the cell from the cell line into the
non-human animal. In some embodiments of any of the embodiments
disclosed herein, the cell lines of the present invention are all
derived from a single progenitor cell line that is transformed by
transfection with an a nucleic acid encoding an HRAS gene. In some
embodiments, the progenitor cell line is an NIH3T3 cell transformed
with an HRAS gene.
Also provided herein, in other aspects, are one or more non-human
animal(s) comprising tumorigenic cells derived from any of the cell
lines disclosed herein. In some embodiments, the non-human animal
is a mouse. In some embodiments, the mouse is immunocompromised. In
some embodiments, the mouse is a nude NIH Swiss mouse.
In other aspects, provided herein is a method for producing a
series of non-human animals with increasingly aggressive tumors,
the method comprising: injecting at least one cell from the T1-A
cell line disclosed herein subcutaneously into a first non-human
animal, wherein the first non-human animal develops a primary
tumor; injecting at least one cell from the T1-A cell line
disclosed herein intravenously a second non-human animal, wherein
the second non-human animal develops a locally metastatic tumor;
injecting at least one cell from the T2-A cell line disclosed
herein intravenously into a third non-human animal, wherein the
third non-human animal develops a distantly metastatic tumor;
injecting at least one cell from the T3-PA cell line disclosed
herein intravenously into a fourth non-human animal, wherein the
fourth non-human animal develops a metastatic lung tumor; injecting
at least one cell from the T3-HA cell line disclosed herein
intravenously into a fifth non-human animal, wherein the fifth
non-human animal develops a metastatic liver or lung tumor; and
injecting at least one cell from the T4-PA cell line disclosed
herein intravenously into a sixth non-human animal, wherein the
sixth non-human animal develops more than one distant metastatic
tumors in more than one organs or extremities, thereby producing a
series of non-human animals with increasingly aggressive
tumors.
In further aspects, provided herein is a system for evaluating the
anti-neoplastic potential of a candidate therapeutic agent against
neoplastic cells from a specific stage of tumorigenesis comprising
two or more of the non-human animals produced by any of the methods
disclosed herein and one or more candidate therapeutic agents.
In another aspect, provided herein is a method for evaluating the
anti-neoplastic potential of a candidate agent against neoplastic
cells from a specific stage of tumorigenesis, the method
comprising: contacting the agent with any of the cell lines
disclosed herein or one or more of the non-human animals disclosed
herein; and evaluating the anti-neoplastic potential of the
candidate agent by determining the effect of said agent on
neoplastic cell growth inhibition or tumor growth inhibition from a
specific stage of tumorigenesis. In some embodiments, the agent is
selected from the group consisting of a ribozyme, an antisense
nucleic acid, a morpholino, a small inhibitory (si)RNA a double
stranded (ds)RNA, a micro (mi)RNA, a small molecule chemical
compound, a non-antibody binding polypeptide, and an antibody or
functional fragment thereof.
In further aspects, provided herein is a kit comprising: one or
more of the cell lines of disclosed herein. In another embodiment,
the kit further comprises one or more of the non-human animals
disclosed herein. Each of the aspects and embodiments described
herein are capable of being used together, unless excluded either
explicitly or clearly from the context of the embodiment or
aspect.
In still other aspects, provided herein are cell lines obtained
from: transfecting ATCC CRL1658 cells with a Harvey-ras oncogene
(HRAS) to form a transformed parent cell population; injecting the
transformed parent cell population into a mouse, wherein the
transformed parent cell population forms a tumor; excising the
resultant tumor; culturing the cells of the excised tumor;
injecting the cultured cells of the excised tumor into a second
mouse; excising tumor from the second mouse; and culturing the
cells of the tumor of the second mouse. In some embodiments, the
mouse is an NIH Swiss mouse. In some embodiments of any of the
embodiments disclosed herein, the injection is into the
hindquarter. In some embodiments of any of the embodiments
disclosed herein, the second mouse is a nude NIH Swiss mouse. In
some embodiments of any of the embodiments disclosed herein, the
injection is into the tail vein of the second mouse. In some
embodiments of any of the embodiments disclosed herein, the
embodiment further comprises injecting the cultured cells of the
excised tumor into a third mouse; excising a locally metastatic
tumor from the third mouse; and culturing the cells of the locally
metastatic tumor of the third mouse. In some embodiments, the
embodiment further comprises injecting the cultured cells of the
locally metastatic lesion into a fourth mouse; excising a
metastatic lung or liver tumor from the fourth mouse; and culturing
the cells of the metastatic lung or liver tumor of the fourth
mouse. In some embodiments, the embodiment further comprises
injecting the cultured cells of the locally metastatic lesion into
a fifth mouse; excising a distantly metastatic tumor from the fifth
mouse; and culturing the cells of the distantly metastatic tumor of
the fifth mouse.
BRIEF DESCRIPTION OF THE DRAWINGS
The patent application file contains at least one drawing executed
in color. Copies of this patent or patent application publication
with color drawing(s) will be provided by the Office upon request
and payment of the necessary fee.
FIG. 1 is a schematic overview of a method of producing cell lines
of the present invention.
FIG. 2A-FIG. 2N show photomicrographs of normal and transformed
cells. FIG. 2A and FIG. 2B show NIH Swiss embryonic 100.times.,
lower and higher cell densities, respectively. FIG. 2C and FIG. 2D
show NIH/3T3 100.times., lower and higher cell densities,
respectively. FIG. 2E and FIG. 2F show GhrasT-NIH/3T3 100.times.,
lower and higher cell densities, respectively. FIG. 2G and FIG. 2H
show T1-A 100.times., lower and higher cell densities,
respectively. FIG. 2I and FIG. 2J show T2-A 100.times., lower and
higher cell densities, respectively. FIG. 2K and FIG. 2L show T3-HA
100.times., lower and higher cell densities, respectively. FIG. 2M
and FIG. 2N show T4-PA 100.times., lower and higher cell densities,
respectively.
FIG. 3 shows detection of mouse and human ras genes by PCR
analysis. Agarose gel electrophoresis of mouse and human HRAS PCR
products: total DNA was isolated from NIH/3T3, T-2A and T4-PA cell
lines and mouse liver. Human placenta DNA was purchased
commercially. PCR Reactions were performed using human primer set
(HN-3630 with HT-4781) or mouse primer set (MN-1121 with MT-1336)
on each DNA sample. Products were analyzed by agarose gel
electrophoresis and portions of each gel photograph are shown at
the human 1152 bp and mouse 216 bp size regions detected in
ethidium bromide stained slab gels. Lanes 1, 2, 5, 6, 9, 10, 13,
14, 17 and 18 show results from the mouse primer set and lanes 3,
4, 7, 8, 11, 12, 15, 16, 19 and 20 show results from the human
primer set. No products appeared in controls with each primer set
without added DNA (not shown). M1/4 primer set, H1/4 Human primer
set.
FIG. 4 is a graph plotted using a gridded plate method to determine
growth rates for seven cell types. Each cell type was plated,
allowed to grow at least one day and fresh media was added.
Photomicrographs were recorded at 100.times. of 6 predetermined
2.times.2 mm.sup.2 grids on each plate at the various time
intervals indicated. A depiction of selecting random grids for a
plate is illustrated in the FIG. 4 insert. The average number of
cells in the 6 grids counted in the plate for each cell type each
day was determined along with a standard deviation. Relative growth
fold increase was determined by normalizing each average cell count
to the cell count average of the earliest time point shown in the
semi-log graph. To plot all the data on the same graph, the first
time point for each cell type was displaced by a one-day on this
graph. The graph for each cell type includes some portion of the
log phase of growth from which the doubling times were determined
as listed in Table 3. The R2 value for each growth curve was
determined when possible. Doubling times were determined from each
curve and compared, in the case of the NIH/3T3 cells, to the
doubling times determined from two experiments using the standard
hemocytometer method of counting viable cells harvested each day
from 4 multiple plates. Six random grids marked on the bottom of a
60 mm diameter plate with 2.times.2 mm.sup.2 grids is depicted here
and grids are not shown to scale.
DETAILED DESCRIPTION OF THE INVENTION
The human transforming HRAS gene cloned from either the T24 or EJ
bladder carcinoma cell lines is well known to transform the
immortal NIH/3T3 cell line [21]. The ras oncogenes (HRAS, KRAS and
NRAS) are known to exploit their signaling pathways to help drive
tumorigenesis in a large number of cancer types [26]. The
transforming DNA from the EJ human bladder carcinoma cell line
(EJ-Ha-ras) was identified as the activated HRAS oncogene. The
EJ-6-2-Bam-6a cell line (ATTC CRL-1888) was established by
transfection of this activated HRAS oncogene into immortalized
NIH/3T3 cells. This transfected cell line, paired with the
untransfected NIH/3T3 cell line, is being used globally to evaluate
the effects of HRAS transformation in the tumorigenic process. They
are being used for many recent approaches to understand the control
of cell death in the malignant cell [13], and to evaluate the
mechanisms cancer cells utilize to alter their metabolism [15].
They have also been employed to devise ways to target cancer cells
with specific decorated nanoparticles to image and ultimately kill
cancer cells when the particles are attached to tumor-specific
toxins [24].
The present application provides a series of cell lines established
from an HRAS transfection system using T24 human bladder carcinoma
DNA as well as use of the cell lines to generate non-human
animal-based systems and models for evaluating the anti-neoplastic
potential of candidate therapeutics. The inventors of the present
invention used a novel serially extended in vivo/in vitro approach
and HRAS transfected NIH/3T3 cells to derive a series of
increasingly aggressive tumorigenic cell lines representative of
the various stages in tumor progression and which include several
additional metastatic cell types (FIG. 1, graphical abstract). The
advantage of the cell lines and model systems disclosed herein lies
in the fact that all transformed cell lines in the series are
derived from a common transfected parent cell population, namely, a
transformed GhrasT-NIH/3T3 cell line, which was generated by
transfection of a non-transformed NIH/3T3 cell line with DNA from
the T24 human bladder carcinoma.
The first cell line in the series (T1-A) was cultured from a
primary tumor in a NIH/Swiss mouse injected subcutaneously with the
GhrasT-NIH/3T3 cells. The second cell line (T-2A) was cultured from
a subsequent secondary local metastasis in a NIH/Swiss mouse
injected intravenously with T1-A cells. The third cell line (T3-HA)
was cultured from a tertiary liver metastatic tumor in a nude
NIH/Swiss mouse injected intravenously with T2-A cells. The fourth
cell line (T4-PA) was cultured from a quaternary metastatic tumor
in a nude NIH/Swiss mouse injected intravenously with T3-HA cells.
These T4-PA cells caused wide spread metastases following
intravenous injection into nude mice.
Accordingly, the cell lines and non-human animal-based in vivolin
vitro model systems disclosed herein represent a unique tool for
evaluating the therapeutic potential of candidate anti-neoplastic
drugs against a series of increasingly aggressive tumorigenic cells
and lesions derived from a single progenitor population.
Furthermore, the cell lines described herein offer the opportunity
to evaluate single cell variations within and among a variety of
cell lines serially derived from a common tumorigenic event(s).
Consequently, the cell lines of the present invention may be used
to provide insights into potential stochastic chromosomal
rearrangements, eukaryotic-phenotypic, genetic, epigenetic, and
metabolic network changes that have occurred within the progression
leading from localized tumors to highly metastatic and invasive
cells.
I. General References
The practice of the present invention will employ, unless otherwise
indicated, conventional techniques of molecular biology,
microbiology, cell biology, biochemistry, nucleic acid chemistry,
and immunology, and mouse breeding and genetics which are well
known to those skilled in the art. Such techniques are explained
fully in the literature, such as, Molecular Cloning: A Laboratory
Manual, fourth edition (Sambrook et al., 2012) and Molecular
Cloning: A Laboratory Manual, third edition (Sambrook and Russel,
2001), (jointly referred to herein as "Sambrook"); Current
Protocols in Molecular Biology (F. M. Ausubel et al., eds., 1987,
including supplements through 2014); PCR: The Polymerase Chain
Reaction, (Mullis et al., eds., 1994); Antibodies: A Laboratory
Manual, Second edition, Cold Spring Harbor Laboratory Press, Cold
Spring Harbor, N.Y. (Greenfield, ed., 2014), Beaucage et al. eds.,
Current Protocols in Nucleic Acid Chemistry, John Wiley & Sons,
Inc., New York, 2000, (including supplements through 2014).
II. Definitions
"Neoplastic," as used herein, refers to any form of dysregulated or
unregulated cell growth, whether malignant or benign, resulting in
abnormal tissue growth. Thus, "neoplastic cells" include malignant
and benign cells having dysregulated or unregulated cell growth. In
some embodiments, neoplastic cells are tumor cells or cancer cells
(such as metastatic cancer cells).
The term "anti-neoplastic" as used herein is intended to encompass
both destruction/killing/lysis of neoplastic cells as well as
preventing their growth and/or replication. It refers to the
ability to inhibit or prevent the development or spread of a
neoplasm, and to limit, suspend, terminate, or otherwise control
the maturation and proliferation of cells in a neoplasm. Thus, the
activity of an "anti-neoplastic agent," can be tumoricidal or
tumoristatic in nature.
As used herein, "tumorigenic" means an ability to form a tumor in a
host non-human animal (such as a rodent, for example, a mouse).
"Tumorigenesis" is understood as the production of a new tumor or
tumors; or the process involved in the production of a new tumor or
tumors. Tumorigenesis as used herein includes both the generation
of tumors de novo from neoplastic cells and/or the generation of
metastatic tumors from an established tumor.
"Metastasis," as used herein, is understood as the propagation of
neoplastic cells from the organ or tumor where they originated to a
different organ or tissue. It generally occurs through the blood or
lymphatic system. When the neoplastic cells spread and form a new
tumor, the latter is called a secondary or metastatic tumor. As
used herein, the term "metastatic lesion" refers to a tumor cell
introduced into the blood system that escapes the circulation to
initiate or form a tumor.
As used herein, "primary tumor" refers to a tumor that develops at
the site of subcutaneous injection of neoplastic cells in a
non-human animal (such as a rodent) and also includes the site of
occurrence of a spontaneous natural-occurring tumor. When
neoplastic cells from a primary tumor spread and form a new tumor,
the latter is called a "secondary" or "metastatic tumor."
"Locally metastatic," as used herein, refers to a metastatic tumor
that forms near a primary tumor and is derived from the primary
tumor. It also refers to a tumor that may occur near the site of a
primary tumor after the primary tumor has been surgically removed
and is derived from the original tumor. In some embodiments, a
locally metastatic tumor is formed from cells that have migrated a
short distance from the site of intravenous injection before
escaping the blood circulation and forming a tumor near the site of
the primary tumor.
"Distantly metastatic," as used herein, refers to a metastatic
tumor that forms a significant distance from the site of the
primary tumor and is derived from the primary tumor. In some
embodiments, a metastatic tumor is formed from cells that have
migrated a significant distance from the site of intravenous
injection before escaping the blood circulation and forming a
distant tumor (such as a tumor located in a different organ or
tissue compared to the location of the primary tumor).
"Transformed," as used herein, refers to a cell that was changed
from a normal cell to a malignant cell that is capable of producing
a cancerous tumor. It can also produce a growth in soft agar when
cultured in vitro. Transformed cells have lost the property of
contact-inhibited growth in vitro when grown in cell culture
dishes. A transformed cell will grow in multi-layers in cell
culture dishes, are immortal, and can divide indefinitely. In some
embodiments, the GhrasT-NIH/3T3, T1-A, T-2A, T3-PA, T3-HA, and
T3-PA cells disclosed herein are all transformed. It is understood
that aspects and embodiments of the invention described herein
include "consisting of" and "consisting essentially of" aspects and
embodiments.
Reference to "about" a value or parameter herein includes (and
describes) variations that are directed to that value or parameter
per se. For example, description referring to "about X" includes
description of "X."
Unless defined otherwise herein, all technical and scientific terms
used herein have the same meaning as commonly understood by one of
ordinary skill in the art to which this invention pertains.
As used herein, the singular terms "a," "an," and "the" include the
plural reference unless the context clearly indicates
otherwise.
It is intended that every maximum numerical limitation given
throughout this specification includes every lower numerical
limitation, as if such lower numerical limitations were expressly
written herein. Every minimum numerical limitation given throughout
this specification will include every higher numerical limitation,
as if such higher numerical limitations were expressly written
herein. Every numerical range given throughout this specification
will include every narrower numerical range that falls within such
broader numerical range, as if such narrower numerical ranges were
all expressly written herein.
III. Compositions
Provided herein is a series of tumorigenic cell lines wherein cells
from each cell line in the series are capable of developing into
increasingly aggressive tumors when injected into a non-human
animal. The table below lists the claimed cell line designations
and their corresponding deposit dates.
TABLE-US-00001 Cell line ATCC Deposit Designation Designation ATTC
Deposit Date T1-A PTA-123697 26 Apr. 2017 T2-A PTA-123698 12 Apr.
2017 T3-PA PTA-123699 5 Apr. 2017 T3-HA PTA-123513 12 Oct. 2016
T4-PA PTA-123514 19 Oct. 2016
The cell lines were deposited and will be made available to the
public without restriction, but subject to patent rights, with the
American Type Culture Collection (ATCC), 10801 University
Boulevard, Manassas, Va., 20110. The claimed cell lines were
deposited on behalf of Grove City College. These deposits were made
and will be maintained in accordance with, and under the terms of,
the Budapest Treaty with respect to cell line deposits for the
purposes of patent procedure. These deposits will be maintained
without restriction at the ATCC depository, which is a public
depository, for a period of 30 years, or five years after the most
recent request, or for the effective life of the patent, whichever
is longer, and will be replaced if they become nonviable during
that period.
A. Cell Lines
As described in Example 1 and as illustrated in FIG. 1, the cell
lines of the present invention were all generated from a progenitor
GhrasT-NIH/Swiss cell line that itself was produced from
transformation of NIH/3T3 cells by transfection with HRAS oncogene
DNA from the T24 human bladder carcinoma. These GhrasT-NIH/Swiss
cells were injected subcutaneously into NIH/Swiss mice to produce
primary tumors from which one was used to establish the T1-A cell
line. T1-A cells injected intravenously into the tail vein of a
NIH/Swiss mouse produced a local metastatic tumor near the base of
the tail from which the T2-A cell line was established. T2-A cells
injected intravenously into the tail vein of a nude NIH/Swiss mouse
produced metastases in the liver and lung of that mouse from which
the T3-HA (H=hepatic) and T3-PA (P=pulmonary) cell lines were
developed, respectively. T3-HA cells injected intravenously into
the tail vein of a nude mouse produced a metastasis in the lung
from which the T4-PA cell line was established.
1. T1-A Cells
Provided herein are transformed T1-A cells which were derived from
the transformed GhrasT-NIH/Swiss cell line which was transformed by
transfection of non-transformed NIH/3T3 cells with HRAS oncogene
DNA from the T24 human bladder carcinoma. Specifically, T1-A cells
are derived from a primary tumor caused by injection of the
progenitor GhrasT-NIH/Swiss cell line and are themselves less
tumorigenic than the T1-A cells. In some embodiments, T1-A cells
can be used to generate primary tumors in non-human animals by
injecting them subcutaneously into the non-human animals. In such
instances, about 85% to about 100%, such as any of about 85%, 86%,
87%, 88%, 89%, 90%, 91%, 92%, 93%, 94%, 95%, 96%, 97%, 98%, 99%, or
100% of animals injected subcutaneously with T1-A cells develop
primary tumors. In some embodiments, the non-human animals develop
primary tumors within about 7-10 days following injection, such as
any of about 7, 8, 9, or 10 days following injection. In some
embodiments, the non-human animal is a rodent, such as a mouse or a
rat. In other embodiments, the non-human animal is a mouse, such as
an immunocompromised mouse or a non-immunocompromised mouse. In
other embodiments, T1-A cells can be used in vitro as a model for
assaying the anti-neoplastic properties of candidate therapeutics
against cancer cells that form primary tumors, but which have not
yet acquired metastatic potential. In further embodiments, T1-A
cells exhibit a doubling time of about 17.5 hours in vitro.
In other embodiments, T1-A cells can be used to generate locally
metastatic tumors in non-human animals by injecting them
intravenously into non-human animals. In such instances, about 85%
to about 100%, such as any of about 85%, 86%, 87%, 88%, 89%, 90%,
91%, 92%, 93%, 94%, 95%, 96%, 97%, 98%, 99%, or 100% of animals
injected intravenously with T1-A cells develop locally metastatic
tumors. In some embodiments, the non-human animals develop primary
tumors within about 12-14 days following injection, such as any of
about 12, 13, or 14 days following injection. In some embodiments,
the non-human animal is a rodent, such as a mouse or a rat. In
other embodiments, the non-human animal is a mouse, such as an
immunocompromised mouse or a non-immunocompromised mouse. In other
embodiments, T1-A cells can be used in vitro as a model for
assaying the anti-neoplastic properties of candidate therapeutics
against cancer cells that form locally metastatic tumors but which
have not yet acquired distantly metastatic potential.
In some embodiments, T1-A cells are cells from ATCC deposit number
PTA-123697, or cells having all of the identifying characteristics
of ATCC deposit number PTA-123697.
2. T2-A Cells
T2-A cells were derived from tumors produced in immunocompromised
mice by injecting them intravenously with locally metastatic T1-A
cells. In some embodiments, T2-A cells can be used to generate
locally metastatic or distantly metastatic tumors in at least one
organ or tissue in non-human animals by injecting them
intravenously into the non-human animals. For example, non-human
animals injected with T2-A cells can develop tumors in one or more
of the liver, lung, pericardium, or throat. About 50% to about
100%, 60% to about 100%, about 70% to about 100%, about 80% to
about 100%, about 90% to about 100% such as any of about 50%, 51%,
52%, 53%, 54%, 55%, 56%, 57%, 58%, 59%, 60%, 61%, 62%, 63%, 64%,
65%, 66%, 67%, 68%, 69%, 70%, 71%, 72%, 73%, 74%, 75%, 76%, 77%,
78%, 79%, 80%, 81%, 82%, 83%, 84%, 85%, 86%, 87%, 88%, 89%, 90%,
91%, 92%, 93%, 94%, 95%, 96%, 97%, 98%, 99%, or 100% of animals
injected intravenously with T2-A cells develop locally metastatic
or distantly metastatic tumors. In some embodiments, the non-human
animal is a rodent, such as a mouse or a rat. In other embodiments,
the non-human animal is a mouse, such as an immunocompromised mouse
or a non-immunocompromised mouse. In other embodiments, T2-A cells
can be used in vitro as a model for assaying the anti-neoplastic
properties of candidate therapeutics against cancer cells that have
progressed passed the primary tumor or locally metastatic stage and
which have become at least partly metastatic. In further
embodiments, T2-A cells exhibit a doubling time of about 15.5
hours.
In some embodiments, T2-A cells are cells from ATCC deposit number
PTA-123698, or cells having all of the identifying characteristics
of ATCC deposit number PTA-123698.
3. T3-PA Cells
T3-PA cells were derived from tumors produced in immunocompromised
mice by injecting them intravenously with metastatic T2-A cells
that had formed tumors in the lungs. In some embodiments, T3-PA
cells can be used to generate metastatic lung tumors in non-human
animals. About 50% to about 100%, 60% to about 100%, about 70% to
about 100%, about 80% to about 100%, about 90% to about 100% such
as any of about 50%, 51%, 52%, 53%, 54%, 55%, 56%, 57%, 58%, 59%,
60%, 61%, 62%, 63%, 64%, 65%, 66%, 67%, 68%, 69%, 70%, 71%, 72%,
73%, 74%, 75%, 76%, 77%, 78%, 79%, 80%, 81%, 82%, 83%, 84%, 85%,
86%, 87%, 88%, 89%, 90%, 91%, 92%, 93%, 94%, 95%, 96%, 97%, 98%,
99%, or 100% of non-human animals injected intravenously with T3-PA
cells develop lung tumors. In some embodiments, the non-human
animal is a rodent, such as a mouse or a rat. In other embodiments,
the non-human animal is a mouse, such as an immunocompromised mouse
or a non-immunocompromised mouse. In other embodiments, T3-PA cells
can be used in vitro as a model for assaying the anti-neoplastic
properties of candidate therapeutics for cancer cells that have
progressed into a stage corresponding to metastatic tumors
localized in the lung.
In some embodiments, T3-PA cells are cells from ATCC deposit number
PTA-123699, or cells having all of the identifying characteristics
of ATCC deposit number PTA-123699.
4. T3-HA Cells
T3-HA (H=hepatic) cells were derived from a metastatic tumor formed
in the liver of an immunocompromised mouse injected intravenously
with metastatic T2-A cells that had formed tumors in the liver. In
some embodiments, T3-HA cells can be used to generate metastatic
lung and liver tumors in non-human animals. About 50% to about
100%, 60% to about 100%, about 70% to about 100%, about 80% to
about 100%, about 90% to about 100% such as any of about 50%, 51%,
52%, 53%, 54%, 55%, 56%, 57%, 58%, 59%, 60%, 61%, 62%, 63%, 64%,
65%, 66%, 67%, 68%, 69%, 70%, 71%, 72%, 73%, 74%, 75%, 76%, 77%,
78%, 79%, 80%, 81%, 82%, 83%, 84%, 85%, 86%, 87%, 88%, 89%, 90%,
91%, 92%, 93%, 94%, 95%, 96%, 97%, 98%, 99%, or 100% of non-human
animals injected intravenously with T3-PA cells develop liver and
lung tumors. In some embodiments, the non-human animal is a rodent,
such as a mouse or a rat. In other embodiments, the non-human
animal is a mouse, such as an immunocompromised mouse or a
non-immunocompromised mouse. In other embodiments, T3-HA cells can
be used in vitro as a model for assaying the anti-neoplastic
properties of candidate therapeutics against cancer cells that have
progressed into a stage corresponding to metastatic tumors
localized in the liver. In further embodiments, T3-HA cells exhibit
a doubling time of about 17.5 hours.
In some embodiments, T3-HA cells are cells from ATCC deposit number
PTA-123513, or cells having all of the identifying characteristics
of ATCC deposit number PTA-123513.
5. T4-PA Cells
T4-PA cells were derived from tumors produced in immunocompromised
mice by injecting them intravenously with T3-HA cells that had
formed tumors in the liver. In some embodiments, T4-PA cells can be
used to generate metastatic tumors in non-human animals to more
than one location (other than the liver), such as, without
limitation, the liver, neck, lungs, intestines, legs, and in the
groin and pancreas areas. In some embodiments, T4-PA cells can be
used to generate primary tumors in non-human animals by injecting
them subcutaneously into the non-human animals. In some
embodiments, T4-PA cells can be used to generate locally
metastastic and metastatic tumors in non-human animals by injecting
them intravenously into the non-human animals. In such instances,
about 85% to about 100%, such as any of about 85%, 86%, 87%, 88%,
89%, 90%, 91%, 92%, 93%, 94%, 95%, 96%, 97%, 98%, 99%, or 100% of
animals injected intravenously with T4-PA cells develop multiple
(such as 2, 3, 4, 5 or 6) metastatic tumors. In some embodiments,
the non-human animal is a rodent, such as a mouse or a rat. In
other embodiments, the non-human animal is a mouse, such as an
immunocompromised mouse or a non-immunocompromised mouse. In other
embodiments, T4-PA cells can be used in vitro as a model for
assaying the anti-neoplastic properties of candidate therapeutics
against cancer cells that have progressed into a highly metastatic
stage. In further embodiments, T4-PA cells exhibit a doubling time
of about 18.5 hours.
In some embodiments, T4-PA cells are cells from ATCC deposit number
PTA-123514, or cells having all of the identifying characteristics
of ATCC deposit number PTA-123514.
B. Non-Human Animals
Also provided herein are non-human animals comprising any of the
cells or cell lines disclosed herein (such as T1-A, T2-A, T3-PA,
T3-HA, or T4-PA cells). Such non-human animals can be used as model
systems, alone or in series (such as, for example, wherein one or
more animals comprises T1-A cells, one or more animals comprises
T2-A cells, one or more animals comprises T3-PA cells, etc.), in
order to evaluate the anti-neoplastic potential of a candidate
agent against neoplastic cells from a specific stage of
tumorigenesis.
The term "non-human animal" refers to any animal other than a
human. Examples of non-human animals include, without limitation,
aquatic animals (e.g., fish, sharks, dolphin, and the like), farm
animals (e.g., pigs, goats, sheep, cows, horses, rabbits, and the
like), rodents (e.g., rats, guinea pigs, and mice), non-human
primates (e.g., baboon, monkeys, and chimpanzees), and domestic
animals (e.g., dogs and cats). The non-human animals provided
herein can be immunocompromised or immunodeficient. For example, a
non-human animal can be a severe combined immunodeficiency (SCID)
animal, such as a mouse (e.g., an X-linked SCID or a RAG1.sup.-/-
or RAG2.sup.-/- mouse).
Methods for generating non-human animals comprising any of the
cells of the present invention are well known in the art,
particularly for rodent model organisms such as mice and rats.
Cells can be introduced into the animal by any means known in the
art such as, without limitation, by intravenous administration,
subcutaneous administration, or by graft.
IV. Methods of the Invention
Also provided herein are methods for evaluating the anti-neoplastic
potential of a candidate agent against neoplastic cells from a
specific stage of tumorigenesis. The method involves contacting one
or more of the cells described herein (such as one or more of a
T1-A, T2-A, T3-PA, T3-HA, or T4-PA cells) with a candidate
anti-neoplastic therapeutic agent and evaluating the
anti-neoplastic potential of the candidate agent by determining the
effect of the candidate therapeutic agent on neoplastic cell growth
inhibition and/or tumor growth inhibition on a specific stage of
tumorigenesis (such one or more of a primary tumor, a locally
metastatic tumor, a lung or liver metastatic tumor, or a highly
aggressive distant metastatic tumor). In some embodiments, the
cells can be present in a non-human animal (such as a rodent, for
example, a mouse) that has been injected with the cells in order to
form a tumor or tumorigenic lesion. Cell culture is routine and can
be accomplished via any method known in the art.
Accordingly, "contacting" used herein includes physically
contacting any of the cells disclosed herein or non-human animals
that have neoplasms or tumors caused by any of the cells disclosed
herein with a candidate therapeutic agent or introducing (for
example by injecting or via ingestion) the compound into the
non-human animals. In some embodiments, the cells are contained in
an aqueous medium in a microtiter well, such as in a multi-well
plate, e.g., a 96-well plate. In some embodiments, the candidate
therapeutic agents are administered to the cells or to the
non-human animals by electroporation, lipofection, or ingestion or
by using bolistic cell loading technology in which particles coated
with the candidate therapeutic agent are introduced into the cell
or tissue of interest as a bolus using a high-pressure gun. The
cells of the present invention may be pretreated prior to exposure
to the candidate therapeutic agent, for example to facilitate the
penetration and/or contacting of the candidate therapeutic
agent.
In some embodiments, the candidate therapeutic agent is
administered to the cells by dissolving the candidate therapeutic
agent in media containing the cells. Alternatively, the candidate
therapeutic agent may first be dissolved in the medium and the
cells submerged in the media subsequently. In some embodiments, the
candidate therapeutic agent is administered to the cells by
microinjecting the candidate therapeutic agent into the cells. The
candidate therapeutic agent may be brought into contact with the
cells of the present invention alone, in conjunction with a variety
of solvents (e.g., dimethylsulfoxide (DMSO) or the like) or
carriers (including, e.g., peptide, lipid or solvent carriers), or
in conjunction with other compounds. In some embodiments,
contacting comprises injecting said candidate therapeutic agent
into the non-human animals comprising any of the cells disclosed
herein. Suitable vehicles for injection include, but are not
limited to, E3 buffer and DMSO.
As used herein, the phrase "cell growth inhibition" or "tumor
growth inhibition" means a reduction or cessation of the growth of
a neoplastic cell or a reduction or cessation in the growth of a
tumor. Cell growth inhibition can be determined using any means
known in the art. In some embodiments, cells undergoing growth
inhibition exhibit one or more signs of apoptosis (such as, but not
limited to, membrane blebbing, annexin V staining, DNA
fragmentation, caspase activation, or any other marker of
programmed cell death known in the art). In other embodiments,
tumor growth inhibition can be determined by observing a reduction
in the size of the tumor, for example, by using a caliper or by
ascertaining the weight of an excised tumor.
Candidate anti-neoplastic agents for use in the methods of the
present invention can be an antibody or a non-antibody polypeptide.
Additionally, the agent can be an inhibitory nucleic acid, such as
an antisense oligonucleotide or a siRNA. Further, the agent can
also be a small molecule chemical compound.
A. Antibodies
In some aspects, the candidate anti-neoplastic agent is an
antibody. Antibodies are proteins that bind, preferably
specifically, to other proteins, nucleic acids, lipids, or any
other antigen. Variants of antibodies can be made based on
information known in the art, without substantially affecting the
activity of antibody. For example, antibody variants can have at
least one amino acid residue in the antibody molecule replaced by a
different residue. Fragments of antibodies may also be used (such
as, but not limited to, Fv, Fab, Fab', or F(ab).sub.2 fragments),
as well as genetically-engineered antibodies or antigen binding
fragments thereof, including single chain antibodies, humanized
antibodies, antibodies that can bind to more than one epitope
(e.g., bi-specific antibodies), or antibodies that can bind to one
or more different antigens (e.g., bi- or multi-specific
antibodies), may also be employed. For antibodies, the sites of
greatest interest for substitutional mutagenesis generally include
the hypervariable regions, but framework region (FR) alterations
are also contemplated. For antibodies, one type of substitutional
variant involves substituting one or more hypervariable region
residues of a parent antibody (e.g. a humanized or human antibody).
Generally, the resulting variant(s) selected for further
development will have improved biological properties (e.g.,
affinity) relative to the parent antibody from which they are
generated.
A convenient way for generating such substitutional variants
involves affinity maturation using phage display. Briefly, several
hypervariable region sites (e.g. 6-7 sites) are mutated to generate
all possible amino acid substitutions at each site. The antibodies
thus generated are displayed from filamentous phage particles as
fusions to the gene III product of M13 packaged within each
particle. The phage-displayed variants are then screened for their
biological activity (e.g. binding affinity) as herein disclosed. In
order to identify candidate hypervariable region sites for
modification, alanine scanning mutagenesis can be performed to
identify hypervariable region residues contributing significantly
to antigen binding. Alternatively, or additionally, it may be
beneficial to analyze a crystal structure of the antigen-antibody
complex to identify contact points between the antibody and
antigen. Such contact residues and neighboring residues are
candidates for substitution according to the techniques elaborated
herein. Once such variants are generated, the panel of variants is
subjected to screening as described herein and antibodies with
superior properties in one or more relevant assays may be selected
for further development.
Nucleic acid molecules encoding amino acid sequence variants of the
antibody are prepared by a variety of methods known in the art.
These methods include, but are not limited to, isolation from a
natural source (in the case of naturally occurring amino acid
sequence variants) or preparation by oligonucleotide-mediated (or
site-directed) mutagenesis, PCR mutagenesis, and cassette
mutagenesis of an earlier prepared variant or a non-variant version
of the antibody. It may be desirable to introduce one or more amino
acid modifications in an Fc region of the immunoglobulin
polypeptides of the invention, thereby generating a Fc region
variant. The Fc region variant may comprise a human Fc region
sequence (e.g., a human IgG1, IgG2, IgG3 or IgG4 Fc region)
comprising an amino acid modification (e.g. a substitution) at one
or more amino acid positions including that of a hinge
cysteine.
B. Non-Antibody Binding Polypeptides
In some aspects, the candidate anti-neoplastic agent is a
non-antibody binding polypeptide. Binding polypeptides may be
chemically synthesized using known polypeptide synthesis
methodology or may be prepared and purified using recombinant
technology. Binding polypeptides are usually at least about 5 amino
acids in length, alternatively at least about 6, 7, 8, 9, 10, 11,
12, 13, 14, 15, 16, 17, 18, 19, 20, 21, 22, 23, 24, 25, 26, 27, 28,
29, 30, 31, 32, 33, 34, 35, 36, 37, 38, 39, 40, 41, 42, 43, 44, 45,
46, 47, 48, 49, 50, 51, 52, 53, 54, 55, 56, 57, 58, 59, 60, 61, 62,
63, 64, 65, 66, 67, 68, 69, 70, 71, 72, 73, 74, 75, 76, 77, 78, 79,
80, 81, 82, 83, 84, 85, 86, 87, 88, 89, 90, 91, 92, 93, 94, 95, 96,
97, 98, 99, or 100 amino acids in length or more. Binding
polypeptides may be identified without undue experimentation using
well known techniques. In this regard, it is noted that techniques
for screening polypeptide libraries for binding polypeptides that
are capable of binding to a polypeptide target are well known in
the art (see, e.g., U.S. Pat. Nos. 5,556,762, 5,750,373, 4,708,871,
4,833,092, 5,223,409, 5,403,484, 5,571,689, 5,663,143; PCT
Publication Nos. WO 84/03506 and WO84/03564; Geysen et al., Proc.
Natl. Acad. Sci. U.S.A., 81:3998-4002 (1984); Geysen et al., Proc.
Natl. Acad. Sci. U.S.A., 82:178-182 (1985); Geysen et al., in
Synthetic Peptides as Antigens, 130-149 (1986); Geysen et al., J.
Immunol. Meth., 102:259-274 (1987); Schoofs et al., J. Immunol.,
140:611-616 (1988), Cwirla, S. E. et al., (1990) Proc. Natl. Acad.
Sci. USA, 87:6378; Lowman, H. B. et al., (1991) Biochemistry,
30:10832; Clackson, T. et al., (1991) Nature, 352: 624; Marks, J.
D. et al., (1991), J. Mol. Biol., 222:581; Kang, A. S. et al.,
(1991) Proc. Natl. Acad. Sci. USA, 88:8363, and Smith, G. P. (1991)
Current Opin. Biotechnol., 2:668).
C. Small Molecule Chemical Compounds
In some aspects, the candidate anti-neoplastic agent is a small
molecule chemical compound. Small molecules can be molecules other
than binding polypeptides or antibodies as defined herein. Small
molecules may be identified and chemically synthesized using known
methodology (see, e.g., International Patent Application
Publication Nos. WO00/00823 and WO00/39585). Small molecules are
usually less than about 2000 Daltons in size or alternatively less
than about 1500, 750, 500, 250 or 200 Daltons in size. In this
regard, it is noted that techniques for screening small molecule
libraries for molecules that are capable of binding to a
polypeptide target are well known in the art (see, e.g., PCT
Publication Nos. WO00/00823 and WO00/39585). Small molecules may
be, for example, aldehydes, epoxides, or fluoro methyl ketones.
The small molecule chemical compound may be a component of a
combinatorial chemical library. Combinatorial chemical libraries
are a collection of multiple species of chemical compounds
comprised of smaller subunits or monomers. Combinatorial libraries
come in a variety of sizes, ranging from a few hundred to many
hundreds of thousand different species of chemical compounds. There
are also a variety of library types, including oligomeric and
polymeric libraries comprised of compounds such as carbohydrates,
oligonucleotides, and small organic molecules, etc. Such libraries
have a variety of uses, such as immobilization and chromatographic
separation of chemical compounds, as well as uses for identifying
and characterizing ligands capable of binding an acceptor molecule
or mediating a biological activity of interest (such as, but not
limited to, inhibition of cellular proliferation or tumor cell
growth). Various techniques for synthesizing libraries of compounds
on solid-phase supports are known in the art. Solid-phase supports
are typically polymeric objects with surfaces that are
functionalized to bind with subunits or monomers to form the
compounds of the library. Synthesis of one library typically
involves a large number of solid-phase supports. To make a
combinatorial library, solid-phase supports are reacted with one or
more subunits of the compounds and with one or more numbers of
reagents in a carefully controlled, predetermined sequence of
chemical reactions. In other words, the library subunits are
"grown" on the solid-phase supports. The larger the library, the
greater the number of reactions required, complicating the task of
keeping track of the chemical composition of the multiple species
of compounds that make up the library. In some embodiments, the
small molecules are less than about 2000 Daltons in size,
alternatively less than about 1500, 750, 500, 250 or 200 Daltons in
size.
D. Inhibitory Nucleic Acids
In another aspect, the candidate anti-neoplastic agent is one or
more inhibitory nucleic acid(s). The inhibitory nucleic acid can
be, without limitation, any of an RNA interference agent, such as
any of those disclosed herein (for example, an antisense
oligonucleotide, a siRNA, a dsRNA, or a ribozyme). While preferred,
absolute complementarity of an inhibitory nucleic acid to a target
is not required. As used herein, an inhibitory nucleic acid
sequence is "complementary" to a target nucleic acid when the
inhibitory nucleic acid has a sequence sufficiently complementary
to be able to hybridize with the target, thereby forming a stable
duplex. The ability to hybridize will depend on both the degree of
complementarity and the length of the oligonucleotide. Generally,
the longer the hybridizing inhibitory nucleic acid, the more base
mismatches with a given target it may contain and still form a
stable duplex. A person having ordinary skill in the art can
ascertain a tolerable degree of mismatch by use of standard
procedures to determine the melting point of the hybridized
complex.
Inhibitory nucleic acids can include one or more alternate
internucleoside linkages, such as, but not limited to,
phosphorothioate (Mag at al., Nucleic Acids Res. 19: 1437-1441,
1991; and U.S. Pat. No. 5,644,048),peptide nucleic acid or PNA
(Egholm, Nature, 3685:566-568, 1993; and U.S. Pat. No. 6,656,687),
phosphoramide (Beaucage, Methods Mol. Biol. 20:33-61, 1993),
phosphorodithioate (Capaldi et al., Nucleic Acids Res., 28:E40,
2000). Other oligonucleotide analogs include, but are not limited
to, morpholino (Summerton, Biochim. Biophys. Acta, 1489: 141-158,
1999), locked oligonucleotides (Wahlestedt wt al., Proc. Natl.
Acad. Sci. USA, 97:5633-5638, 2000), peptidic nucleic adds or PNA
(Nielsen et al., 1993; Hyrup and Nielsen, 1996) or
2-o-(2-methoxy)ethyl modified 5' and 3' end oligonucleotides (McKay
et al., Biol. Chem., 274: 1715-1722, 1999). All of the preceding
publications are hereby expressly incorporated by reference.
Further, any of the inhibitory nucleic acids disclosed herein may
additionally contain any combination of deoxyribo- and/or
ribonucleotides, as well as any combination of natural and/or
synthetic bases, including uracil, adenine, thymine, cytosine,
guanine, inosine, xathanine hypoxathanine, isocytosine, isoguanine,
etc.
The inhibitory nucleic acids discussed herein can include one or
more modified base moiety such as, but not limited to,
5-fluorouracil, 5-bromouracil, 5-chlorouracil, 5-Iodouracil,
hypoxanthine, xanthine, 4-acetylcytosine,
5-(carboxyhydroxylmethyl)uracil,
5-carboxymethylaminomethyl-2-thiouridine,
5-carboxymethylaminomethyluracil, dihydrouracil,
beta-D-galactosylqueosine, inosine, N6-isopentenyladenine,
1-methylguanine, 1-methylinosine, 2,2-dimethylguanine,
2-methyladenine, 2-methylguanine, 3-methylcytosine,
5-methylcytosine, N6-adenine, 7-methylguanine,
5-methylaminomethyluracil, 5-methoxyaminomethyl-2-thiouracil,
beta-D-mannosylqueosine, 5'-methoxycarboxymethyluracil,
5-methoxyuracil, 2-methylthio-N6-isopentenyladenine,
uracil-5-oxyacetic acid (v), wybutoxosine, pseudouracil, queosine,
2-thiocytosine, 5-methyl-2-thiouracil, 2-thiouracil, 4-thiouracil,
5-methyluracil, uracil-5-oxy acetic acid methylester,
uracil-5-oxyacetic acid (v), 5-methyl-2-thiouracil,
3-(3-amino-3-N2-carboxypropyl)uracil, (acp3)w, and/or
2,6-diaminopurine.
Inhibitory nucleic acids contemplated within the scope of the
present invention can also have one or more modified sugar moiety
such as, but not limited to, arabinose, 2-fluoroarabinose,
xylulose, and hexose. The inhibitory nucleic acids of the present
invention should be at least ten nucleotides in length, and may
range from 10 to about 50 nucleotides in length, such as 15, 20,
30, 35, 40, 45, or 50 nucleotides in length, inclusive, including
any values falling in between these numbers.
IV. Systems and Kits
Additionally provided herein are systems for evaluating the
anti-neoplastic potential of a candidate agent against neoplastic
cells from a specific stage of tumorigenesis. The system comprises
a) cells from at least two (such as any of about 2, 3, 4, or 5) of
the cell lines disclosed herein, with each cell line representative
of a specific stage of tumorigenesis; and b) one or more candidate
anti-neoplastic agents. In a further embodiment, the system
comprises two or more non-human animals (such as any of about 2, 3,
4, or 5), wherein each animal has been injected with one of the
cell lines disclosed herein, with each cell line representative of
a specific stage of tumorigenesis and one or more candidate
anti-neoplastic agents.
The invention further comprises kits containing one or more of the
cell lines disclosed herein (such as one or more of a T1-A, T2-A,
T3-PA, T3-HA, or T4-PA cells). Alternatively, the kits may contain
additional items for culturing the cells, such as, without
limitation, cell culture plates, cell culture media, and written
instructions for culturing the cells and using the same to
investigate the anti-neoplastic potential of candidate therapeutic
agents. The kit may further include one or more of the non-human
animals disclosed herein (such as a rodent, for example, a mouse).
In some embodiments, the non-human animal is immunocompromised.
The invention can be further understood by reference to the
following examples, which are provided by way of illustration and
are not in any way meant to be limiting.
EXAMPLES
Example 1
Materials and Methods
Animals. Breeding pairs of NIH Swiss and NFS/NCr mice were obtained
from L. Watson at NIH or the Jackson Labs and bred as outbred or
inbred strains, respectively, as described by the Jackson Lab
protocols. All mice at the Oral Roberts University Medical School
were housed in the animal facility of the Biomedical Research
Center, fully accredited by the American Association for the
Accreditation of Laboratory Animal Care, and cared for in
accordance with the guidelines of the National Research Council for
the care and use of laboratory animals under the supervision of a
licensed veterinarian. All mice at Grove City College were cared
for with the same animal studies guidelines for animal use as
approved by the Grove City College Institutional Review Board,
which served as the animal oversight committee at the time. Nude
mice (Nu-/Nu-) were obtained from Jackson Labs, Farmington, Conn.
(common name: nu/nu; strain name: NU/J; stock number: 002019) or
obtained from a breeding pair (Nu/Nu-) offspring. These are from a
spontaneous mutation athymic (nude) inbred mice on an N:NIH (s)
background homozygous for nude marker, Nu-/N- and
immunocompromised. The two main defects of mice homozygous for the
nude spontaneous mutation (Foxn1nu, formerly Hfh11nu) are abnormal
hair growth and defective development of the thymic epithelium. The
Jackson Laboratory imported the nude mutation from the NIH on an
outbred stock. As of 2008, the strain has been inbred for at least
100 generations. The studies reported here with these mice were
completed between 1995 and 2000. NFS/NCr inbred mice were obtained
from the Jackson Lab and maintained as an inbred colony. They have
a very low incidence of spontaneous lymphoma.
Source, Derivation and Growth Characteristics of Each Cell or Cell
Line (FIG. 1). All cells and cell lines were grown at 37.degree. C.
in 5% CO2/95% air in Dulbecco's modified Eagle's medium (DMEM)
(catalogue #12320-032, GIBCO, Grand Island, N.Y.) supplemented with
extra added D- () glucose (catalogue #G-5400, Sigma Chemical
Company, St. Louis, Mo.) (4.5 g/L), 10% fetal calf serum (catalogue
#SH30070.02, Hyclone Laboratories, Logan, Utah), and 20 Units/ml
penicillin, and 20 .mu.g/ml streptomycin (catalogue #15140-122,
Sigma Chemical Company). Extra glucose was included to insure a
rich glucose source for rapidly growing tumor cells that are known
to utilize aerobic glycolysis as a major source of energy and
glycolytic intermediates [39,41].
Normal Primary NIH Swiss Embryonic Cell Cultures (Mortal).These
cells were prepared from 17 to 19 days old NIH Swiss mouse embryos
by fine mincing of whole embryos with scissors by the methods
employed by Todaro and Green [37]. These minces were used for
growing primary normal cell cultures and for DNA extractions as
needed. The tissue was disaggregated with 0.25% trypsin-EDTA
(catalogue #25200-056, GIBCO), cells were collected by
centrifugation, resuspended in saline (0.9% NaCl) (catalogue
#R5200-01, B. Braum Medical, Inc., Irvine Calif.) and when useful,
viable cells were identified by Trypan blue exclusion (catalogue
#15250061, GIBCO), and counted in a hemocytometer. Cells were
plated at 4.3.times.105 cells/60 mm diameter dishes and grown in
D-MEM supplemented as described above. Cultures were expanded and
subcultures were frozen in Recovery Cell Culture Freezing Media
(catalogue #12648-010, GIBCO) and stored in liquid nitrogen tanks
as stocks.
NIH/3T3 Cells (Immortal). The "immortal" NIH/3T3 cells (ATCCs
CRL1658.TM.) cells were purchased from the American Type Culture
Collection (Manassas, Va.) at passage #126. These cells were
originally developed [10] by growing primary embryonic cells,
prepared as described above [37], using a rigid transfer schedule
(every 3-4 days). These cells were non-tumorigenic when
1.times.10.sup.6 cells were injected s.c. into 23 NFS/Ncr inbred
mice or 105 outbred NIH Swiss mice (Table 1). Cultures were
expanded and subcultures were frozen and stored in liquid nitrogen
as stocks.
TABLE-US-00002 TABLE 1 Primary tumor occurances in mice injected
with various cell lines.sup.a. Cell line Recipient mouse Injection
size # Of tumors per # of mice injected % Tumors Time period
NIH/3T3 NFS/NCr (inbred) Hind quarter 0/23 0 NIH/3T3 NIH Swiss
(outbread) Hind quarter 0/105 0 GhrasT-NIH/3T3 NFS/NCr (inbred)
Hind quarter 14/45 31 6-10 weeks GhrasT-NIH/3T3 NIH Swiss
(outbread) Hind quarter 4/104 4 6-10 weeks GhrasT-NIH/3T3 nude
NIH/Swiss Hind quarter 2/2 100 N.A..sup.b T1-A NFS/NCr (inbred)
Hind quarter NIL.sup.c T1-A NIH Swiss (outbread) Hind quarter 20/20
100 7-10 days T1-A NIH Swiss (outbread) Tail vein, i.v. 4/4 100 13
days.sup.d T1-C NFS/NCr (inbred) Hind quarter NIL.sup.c T1-C NIH
Swiss (outbread) Hind quarter 13/14 93 7-10 days .sup.a10 .times.
10.sup.6 cells injected per mouse. .sup.bNot available. .sup.cNot
determined. .sup.dThese 4 tumors were local metastases in the base
of the tails and the T-2A cell line was established from one of
them.
Tumorigenic Ras Oncogene-Transfected NIH/3T3 Cells
(Transformed).The HRAS oncogene-transfected NIH/3T3 cells were
obtained from Dr. David A. Goldthwait at the Department of
Biochemistry, Case Western Reserve University in Cleveland, Ohio
and designated as G-hrasT-NIH/3T3 cells. (G denotes their source
from the Goldthwait Lab, T denotes their transformed cell type.)
The Goldthwait cells were isolated from a focus of transformed
cells following transfection with DNA from the T24 Human bladder
carcinoma. This cell line contains the Harvey-ras oncogene isolated
from the T24 human bladder carcinoma that has been shown to have a
glycine to valine substitution at the twelfth amino acid residue of
the T24 oncogene encoded p21 protein [28]. The pT24-C3 plasmid
(ATCC 41000.TM.) used for the transfection of the NIH/3T3 cell line
by Dr. Goldthwait is a pBR322 derived plasmid containing the 6.6
Kilo base pair BamHI fragment of the T24-Ha-ras1 oncogene and is
carried in E. coli C-600 strain (Goldthwait, D. A., unpublished
results). GhrasT-NIH/3T3 cultures were expanded and subcultures
were frozen and stored in liquid nitrogen as stocks.
Highly Tumorigenic Ras T1-A Cells. Following s.c. injection of
1.times.10.sup.6 GhrasT-NIH/3T3 cells/mouse into hindquarters of
104 outbred NIH Swiss mice, four mice formed single primary tumors
within 6 to 10 weeks at their injection sites (Table 1). Two of
these tumors from separate mice were excised, minced and cultured
as described above giving rise to the T1-A and T1-C cell lines. The
T1-A and T1-C cells were subcultured several times and stocks were
saved frozen in liquid nitrogen. Additionally, to assess the
tumorigenic potential of the T1-A and T1-C cell lines, 20 and 14
outbred NIH Swiss mice were injected s.c. in the hindquarters with
T1-A (1.times.10.sup.6 cells/mouse) or T1-C (1.times.10.sup.6
cells/mouse) cells respectively and observed for tumors at the
injection sites.
T2-A Cell Line from Local Metastasis in NIH Swiss Mouse. To seek a
metastatic lesion derived from these T1-A cells, 1.times.10.sup.6
cells were injected into the tail veins of several NIH Swiss mice.
A tumor was located in the rump near the base of the tail of one
mouse within 13 days of tail vein injection. This local metastasis
was excised, minced and cultured, establishing the T2-A cell line.
These cells were expanded and subcultures were frozen and stored in
liquid nitrogen. Thus, this T2-A cell line was derived from a fast
growing local metastasis close to but not at the site of injection
in the tail.
T3-HA and T3-PA Metastatic Cells from Distant Liver Lung Metastasis
in a Nude NIH Swiss Mouse. In attempts to acquire a highly
metastatic cell line in this series, 1.times.10.sup.6 T2-A cells
were injected into the tail veins of four immune-compromised nude
NIH/Swiss mice. One mouse was sacrificed periodically and examined
for evidence of tumors. After 3.5 weeks a mouse was sacrificed
which contained a metastatic lesion in both the liver and a lung
that were excised, minced, cultured and designated T3-HA
(H=hepatic) and T3-PA (P=pulmonary) cells, respectively. These
cells were expanded in culture and stored in liquid nitrogen.
T4-PA metastatic cells from distant lung metastasis in nude NIH
Swiss Mouse. To determine if the T3-HA cells derived from the first
distant metastatic lesion would generate second metastatic lesions
in the liver, 0.7.times.10.sup.6 T3-HA cells were injected into the
tail veins of four NIH/Swiss nude mice. After 4 weeks, a metastatic
lesion was found in the lung of one mouse. The lesion was excised,
minced, cultured and the cells were designated T4-PA (P=pulmonary)
cells. These cells were expanded and stored in liquid nitrogen.
DNA Extraction. Total DNA was isolated from tissue or cell pellets
by a modification of the methods of Davis et al. [5].
Human Ras Oncogene Polymerase Chain Reaction (PCR) Analysis. The
presence of this human oncogene in the ras transfected
G-hrasT-NIH/Swiss and its descendent cell lines was confirmed by
PCR amplification with the use of human specific H-ras primers.
Mouse specific primers were used for a control PCR. Primers were
designed by methods similar to those of [20] as published
previously [27, p. 422].
Primers Designed: HN, MN=human or mouse primer that becomes the
nontemplate strand of the DNA. HT, MT=human or mouse primer that
becomes the template strand of the DNA. Human ras primers: designed
and named from GenBank: J00277; formerly V00574 [29,36]. These
human specific primers will amplify the same region in either the
normal human proto-oncogene or the human T24-Ha-ras1 oncogene.
TABLE-US-00003 HN-3630 (SEQ ID NO: 1)
5'-CTG-TCT-TCA-ACA-TCC-CAA-ATG-CC-3' HT-4781 (SEQ ID NO: 2)
5'-AGT-GTG-GTA-TTC-CCT-GGA-CAA-AAG-G-3'
Mouse Ras Primers: designed and named from GenBank: Z50013.1 [25].
These mouse specific primers will amplify a region in the normal
mouse proto-oncogene.
TABLE-US-00004 MN-1121 (SEQ ID NO: 3)
5'-GGC-CTT-AGT-TCT-TCT-TGT-CC-3' MT-1336 (SEQ ID NO: 4)
5'-AAC-CAA-CAC-AAA-TAG-GGA-GC-3'
PCR was performed as described previously [27, p. 422]. DNA was
purified as described above and purity and concentration were
analyzed via UV/VIS spectrophotometry. Each 50 .mu.L reaction
contained 10 mM Tis-HCl, pH 8.3, 50 mM KCl, 1.5 mM MgC12, 200 .mu.M
each of dATP, dCTP, dGTP, dTTP, 0.2 .mu.M primer one (forward) and
0.2 .mu.M primer two (reverse), .about.0.5 gig DNA, 1.25 Units of
Taq-Gold polymerase (Applied Biosystems, Grand Island, N.Y.). The
thermocycler program included 10 min at 95.degree. C.; a 40-cycle
repeat of: 1 min at 94.degree. C., 1 min at 55.degree. C., and 1
min at 72.degree. C., and followed by a polishing time of 6 min at
72.degree. C. Agarose slab gel electrophoresis was performed and
EtBr stained gels were photographed by a Gel Doc XR from BIO-RAD
using Quantity Ones software (BIO-RAD, Hercules, Calif.) to analyze
the gels and assign a specific base pair value to each DNA fragment
based on the sizing standards.
Determination of Cell Growth Rates. Cells were plated in the DMEM
described above in 60 mm diameter dishes with 2.times.2 mm.sup.2
square grids (Corning cat #430196, Corning, N.Y.). Six
predetermined squares were premarked with marking pen on each dish
prior to use. The squares were scattered in such a way that each
2.times.2 mm.sup.2 square area would be representative of the
entire plate. Photographs were taken daily when cells reached
20-40% confluency (day 0) with an Olympus DP12 inverted Microscope
(Olympus America, Inc., Melville, N.Y.) with a Digital Camera
System at 100.times. in the center of each selected 2.times.2
mm.sup.2 square. Cell counts for each square were determined from
the photomicrographs and the average for the 6 squares was
determined for each day. Results for each day were normalized to
fold increase relative to day 0. Statistical significance was
determined via T-test.
Example 2
Normal NIH Swiss Embryonic Cells
The normal primary NIH Swiss embryonic cells used to establish the
other cell lines in this tumorigenic progression model were
obtained from 17 to 19 days old NIH Swiss mouse embryos [37]. They
are anchorage dependent, contact inhibited, and mortal, surviving
about 30 cell divisions before they become senescent as typical for
most diploid cell cultures [17,37].
Example 3
NIH/3T3 Cell Line
The NIH/3T3 cell line was derived from NIH Swiss embryonic cells
that have escaped this senescent "crisis" to become, immortal and
contact inhibited. Although not transformed, they already have
obtained properties associated with transformed cells [17]. They
were not tumorigenic (Table 1) in the inbred NFS/NCr mice (0 tumors
in 23 mice injected) or in the outbred NIH Swiss strain of mice (0
tumors in 105 mice injected). Each mouse was injected with
1.times.10.sup.6 cells s.c. This negative result with NFS/NCr mice
was the same as the results of Bernstein and Weinberg [3]. Variable
tumorigenic and metastatic capabilities of NIH/3T3 cells have been
reported. NIH/3T3 cells produce tumors in BALB-c nude mice [8].
These cells grow rapidly and when transferred at 4.3.times.105
cells/50 mm diameter culture dish they form confluent monolayers
within 2-3 days. Differences in NIH/3T3 cell stocks, passage
numbers, site of tumor cell inoculation and cell number
inoculations may be responsible for different outcomes. In
addition, in some transfection experiments, untransfected control
NIH/3T3 cells have been shown to undergo "spontaneous
transformation", thought to be more frequent when these cells are
maintained at confluency for extended times prior to subculturing
[30,8].
Example 4
GhrasT-NIH/3T3
Transfecting the Harvey-ras oncogene into the NIH/3T3 cell line is
well known to transform it into a tumorigenic cell type [3]. To
confirm this, the tumorigenic capability of the ras-oncogene
transfected NIH/3T3 cells (GhrasT-NIH/3T3) was tested for tumor
formation in normal mice. Injection of 1.times.10.sup.6 cells s.c.
into the rear flank of mice produced (20-30 mm diameter) primary
tumors in 14 of 45 (31%) of inbred NFS/NCr mice and in 4 of 104
(4%) of outbred NIH/Swiss mice all within 6-10 weeks (Table 1).
This was different than the findings of Bernstein and Weinberg who
reported 100% tumor formation when injecting equivalent amounts of
the EJ-6-2-Bam-6a cells (ATCC-1888TM) into the NFS/NCr mouse
strain.
Without being bound to theory, these differences may indicate that
the immortalized NIH/3T3 cells transfected with the plasmid
containing the T24 DNA that yielded the GhrasT-NIH/3T3 cells may
have undergone a more severe chaotic genomic rearrangement during
transfection than the NIH/3T3 cells transfected with the plasmid
containing the EJ-6-2DNA that yielded EJ-6-2Bam-6a cell line. Since
several studies have indicated significant phenotypic changes can
occur with transfection with empty vectors into several cell lines
[33], the role of different vectors used to produce these two cell
lines and the stochastic events that occurred during each
transfection process may account for some of the phenotypic
differences.
Consistent with the results of Bernstein and Weinstein's
EJ-6-2-Bam-6a cells' results [3], injection of these GhrasT-NIH/3T3
cells s.c. into the rear flank of two nude NIH Swiss mice produced
tumors at the site of injection in each mouse (Table 1). Other
studies [8] using a third ras oncogene transfected NIH/3T3 cell
line, A51 [7], injected s.c. into BALB/c nude mice generated
primary tumors in 15-25 days. Some of the differences between the
findings with the GhrasT-NIH/3T3 cells and the EJ-6-2-Bam-6a cells
could be related to the number of transfections used to produce
these two cell lines or the specific site where the ras oncogene
BamHI fragment inserted into the two different NIH/3T3 mouse cell
genomes during each transfection process. In summary,
GhrasT-NIH/3T3 cells are transformed, immortal and tumorigenic
(Table 1).
Example 5
Highly Tumorigenic Ras T1-A Cell Line
Two primary tumors selected from the four observed tumors produced
at the cell's injection sites following s.c. injection of
GhrasT-NIH/3T3 cells into 104 outbred NIH Swiss mice were excised,
minced and cultured as described above giving rise to the T1-A and
T1-C cell lines. Their tumorigenic capability was tested by s. c.
injection into the hindquarters of NIH Swiss mice. T1-A cells
produced 20 tumors in 20 mice (100%) and T1-C produced 13 tumors in
14 mice (93%) all within 7-10 days (Table 1). This indicated the
T1-A and T1-C cell lines were significantly more aggressive at
producing tumors than the GhrasT-NIH/3T3 cells that required 6 to
10 weeks for tumor growth. All but one of the 34 mice injected
developed a primary tumor and the tumors developed 4-10 times
faster than the ras-transformed GhrasT-NIH/3T3 cells did in outbred
NIH/Swiss mice (Table 1). Thus, the inherent tumorigenic
capabilities of the T1-A and T-1C cells have each increased in
NIH/Swiss mice as this GhrasT-NIH/3T3 tumor line was moved from
cultured cells to growths in vivo. The similar highly tumorigenic
phenotypes of these two cell lines originating from tumors in
similar in vivo microenvironments in separate mice is consistent
with the concept they each may contain some transitional or
dominant CCAs they acquired from the GhrasT-T-NIH/3T3 cells that
gave them similar selective growth advantages. WGS and SKY analysis
of these cell lines and tumors and downstream cell lines generated
from them could test this possibility. These analyses may help shed
some light on what chromosomal/gene arrangements may give these
cells selective tumorigenic advantages.
Example 6
T2-A Cell Line from Local Metastasis in NIH Swiss Mouse
T1-A cells (1.times.10.sup.6/mouse) were injected i.v. into the
tail veins of NIH Swiss mice (Table 2). A local metastatic tumor
developed in the rump near the base of the tail of one mouse within
13 days, again illustrating a more rapid tumorigenic process than
the original GhrasT-NIH/3T3 cells. No distant metastatic lesions
were found in the mice injected. Cells cultured from this local
metastatic tumor were designed T2-A cells. Therefore, this T2-A
Cell line was derived from a fast growing local metastasis close
to, but not at the site of injection in the tail.
TABLE-US-00005 TABLE 2 Metastatic tumor occurrence in mice after
tail vein injection with various cell lines. Cell line (passage #s)
# t # of mice Of mice injected Outcomes Recipient injected Outcomes
T1-A (n.a.).sup.a NIH Swiss 4 Four had a local metastatic tumor in
rumps near tails after 13 days, none with obvious (outbred)
internal metastatic lesions. The T2-A cell line was established
from one of these local metastases. T2-A (7, 9) Nude NIH/Swiss 10
Four died within 21-27 days; most of the other 6 all had local mets
in rump near tail; two others developed distant metastatic tumors
in the liver, lung pericardium, or throat. The T3-HA cell line was
established from one met in the liver. T3-HA (2, 3) Nude NIH Swiss
3 One died after 58 days, one had no mets after 34 days and one had
metastatic tumors in (inbred) the liver and lung after 27 days.
T4-PA cell line was established from this lung. T4-PA (2, 3) Nude
NIH Swiss 4 One died after 29 days, one had metastases near the
liver, lungs and in the neck region (inbred) after 24 days; one had
tumor at base of tail, metastases in intestines and near spleen
after 30 days; one had met on right hind leg and lower groin area
after 31 days. Cells cultured from these lesions failed to thrive.
.sup.an.a. not available.
Example 7
T3-HA Metastatic Cells from Distant Liver Metastasis in Nude NIH
Swiss Mouse
T2-A cells (1.times.10.sup.6/mouse) were injected into the tail
veins of four immune compromised nude NIH/Swiss mice. Each week one
mouse was dissected to determine the existence of metastatic
lesions. At 3.5 weeks, a mouse was sacrificed and observed to
contain a metastatic lesion in both the liver and a lung. Both
tumors were excised, minced, cultured and designated T3-HA
(H=hepatic) and T3-PA (P=pulmonary) cells, respectively. Therefore,
these cell lines were derived from the local metastatic type T2-A
cells migrating from the tail vein injection site of the nude mouse
to the liver (T3-HA) or to the lung (T3-PA) to form distant
metastatic lesions. This showed that the T2-A cells were capable of
metastasizing to distant locations in the nude NIH/Swiss mice. This
illustrated the first distant metastatic property by the T2-A cells
and the establishment of the T3-HA and T3-PA metastasized cell
lines in this tumor progression model. WGC and SKY analysis of T2-A
and these two metastatic cell lines generated in vivo in a single
mouse might indicate which chromosomal arrangements were common or
not between populations of each cell line. These analyses would
provide interesting aspects of chromosomal arrangements related to
metastatic potential of T2-A cells in vivo giving rise to two cell
lines, each derived from the T2-A cell line, that grew
simultaneously in separate liver or lung microenvironments in the
same mouse.
Example 8
T4-PA Metastatic Cells from Distant Lung Metastasis in Nude NIH
Swiss Mouse
To determine if the T3-HA (liver derived) cells from the first
distant metastatic liver lesion would go to the liver and generate
second metastatic lesions in the liver, 0.7.times.10.sup.6 T3-HA
cells were injected into the tail veins of four NIH/Swiss nude
mice. [T3-PA (lung derived) cells were not tested.] Each week one
mouse was dissected to determine metastatic lesions. After 4 weeks,
a metastatic lesion occurred in the lung of one mouse. The lesion
was excised, minced, cultured and designated T4-PA (P=pulmonary)
cell line. This T4-PA cell line was derived from a tumor that had
formed from hepatic derived metastatic cells migrating from the
tail vein injection site to the lung leaving the circulation to
form a metastatic lesion, thereby showing that the T3-HA cells were
capable of metastasizing to a distant location other than the liver
in the nude NIH/Swiss mice. This illustrated the distant metastatic
property of the T3-HA cells and the establishment of the T4-PA
metastasized cell lines in this tumor progression model.
Example 9
Overall Comparisons of Tumorigenic and Metastatic Properties
Further studies were undertaken to determine if the T3-HA cells and
T4-PA cells metastasize to specific sites. After the T1-A and T-1C
cell lines were discovered to rapidly produce primary tumors when
injected s.c. in immune-competent NIH Swiss outbred mice (Table 1).
They were then injected in the tail veins (i.v.) of these NIH/Swiss
mice to search for metastatic lesions. These produced only local
metastatic lesions near the tail (Table 2). The T2-A cell line was
derived from one of these local metastatic lesions. To enhance the
opportunity to produce a metastatic lesion, T2-A cells were
injected in the tail vein of a group of partially
immune-compromised nude NIH Swiss mice (Table 2). This approach
with these T2-A cells, derived from a single local metastasis in a
NIH/Swiss mouse, did produce distant metastatic liver, lung,
pericardium or throat lesions in nude mice. Cells from a liver
lesion were used to establish the T3-HA cell line (and cells from
the lung of the same mouse were used to establish the T-3-PA cell
line). Thus, this new distant metastatic T3-HA cell line in a nude
mouse was derived serially from cells cultured from a local
metastatic lesion in a NIH/Swiss mouse. These outcomes (Table 2)
indicated that the lung and liver are the most common sites for
distant metastasis to occur in nude mice injected i.v. with either
the T2-A or T3-HA cells. (As stated above, the T3-PA metastatic
cell line has not been evaluated for additional tumorigenic
capabilities.) The T4-PA cells were more metastatic than the other
cell lines since the few nude mice injected developed lesions in a
broader variety of target tissues (Table 2). Although only a few
mice were injected with either cell line, the T4-PA cell line
appears to more likely have undergone a cellular change allowing it
to escape the circulation more easily and invade a broader variety
of distant tissues (Table 2). Representative photomicrographs of
all the cell types are shown in FIGS. 2A through 2N. Further
studies are needed to confirm these initial apparent tumorigenic
differences among these new cell lines that have progressed in
series from a common origin, the GhrasT-NIH/3T3 cells.
Example 10
Location of the Ras Oncogene in the Cell Lines by PCR
The presence of the human HRAS oncogene was confirmed by PCR
amplification using human specific HRAS primers that do not amplify
the mouse h-ras proto-oncogene, but do amplify regions of the human
proto-oncogene or oncogene (FIG. 3). To determine if any tumors
arose from spontaneous tumors and therefore were unrelated to the
original GhrasT-NIH/3T3 cell line, four primers for two PCR assays
were designed. This spontaneous occurrence was a possibility, since
one spontaneous tumor did occur in the nude NIH Swiss mouse colony
used to generate nude mice for these studies. Although the coding
portion of the c-H-ras-1 gene in mouse, human, rat and hamster are
extremely similar, there are significant differences among these
species in their gene's three introns [25]. The two PCR primer sets
described in the material and methods were able to successfully
discriminate between mouse and human h-ras sequences. The human
primers only detect either the human c-H-ras-1 oncogene or the
human normal proto-oncogene (yielding a 1152 bp PCR product from
the 3' nontranslated region). The mouse primers detect only the
mouse h-ras proto-oncogene (the M. musculus gene for C--H-Ras;
yielding a 216 bp PCR product coded in the third intron) present in
the NIH/Swiss genome. The normal mouse ras proto-oncogene should
only be present in the mouse cells, including the mouse cell lines
and mouse liver (control). The human H-ras oncogene should only be
in the GhrasT-NIH/Swiss, T1-A, T2-A, T3-HA and T4-PA cell lines as
well as in human placenta DNA (control) but not in the NIH/Swiss
cells or the NIH/3T3 cell line. This pattern of ras detection was
observed in all lines tested (FIG. 3). The PCR results confirm that
the tumors used to propagate the T1-A, T2-A, T3-HA and T4-PA cell
lines in this tumorigenic model were all derived from the
GhrasT-NIH/3T3 cells and not produced simply by random spontaneous
tumorigenic events, since the human ras oncogene was still found in
the T2-A and T4-PA mouse cell lines. The presence of human ras in
the T1-C and T3-PA cell lines remains untested since they are not
direct precursors to the T2-A or T4-PA cell lines. They are
presumed to contain the human ras oncogene as well since there is
no obvious occurrence of spontaneous tumors in these studies.
Example 11
Growth Rates of Each Cell Line
The growth rates (FIG. 4) for each cell line were determined from
photomicrographs taken at different times of six pre-specified
2.times.2 mm.sup.2 grids on gridded plates in each experiment (FIG.
4 inset). The doubling time in log phase growth for NIH/3T3 cells
determined by this new method compared well with values obtained
from counting cells by hemocytometry in which cells were harvested
from multi-well plates on a daily basis to obtain doubling times
(Table 3 and FIG. 4). An advantage of this new method includes the
ability to quantify the cell growth within the same six defined
areas within a single dish over the period of the experiment. This
is accomplished with fewer dishes plated initially and eliminates
variations in cell counts inherent in recovering an unequal
percentage of cells from replicate dishes in the preparations
required to use the hemocytometer. Moreover the cells can be
recounted from the photomicrographic records if necessary. The
average doubling time of the transformed cells GhrasT-NIH/3T3 (17
h), TIA (17.5 h), T2A (15.5 h), T3-HA (17.5 h) and T4-PA (18.5 h)
was 17.2 (st.dev. 1.1 h). The doubling times for the NIH Swiss
primary cells and NIH/3T3 cells were 22 h each. Therefore the
average doubling time for the transformed cell lines was
significantly shorter than that of the non-transformed cell types
(P 1/4 0.006). In cell growth inhibitor studies ethanol or DMSO
needed to solubilize test compounds can be as high as 0.1% in
control cultures without significant growth rate effects compared
to growth rates in media without added ethanol or DMSO. (D. B. Ray,
pers. comm.).
TABLE-US-00006 TABLE 3 Doubling times for normal and transformed
cells from photomicrographs or hemocytometer. R.sup.2 in log Growth
phase Graph Doubling curve exponential data day times in fit pts
zero Cells hours.sup.a R.sup.2 used Counting method 0 NIH Swiss 22
0.99783 0, 1, 2, 3 Gridded plate.sup.b 1 NIH/3T3 22 1, 2 Gridded
plate 1 NIH/3T3 22 0.99966 1, 2, 3 Hemocytometer-1.sup.c 1 NIH/3T3
22 1, 4 Hemocytometer-2 Average for 22 normal 2 GhrasT-NIH/ 17
0.99402 1, 2, 5 Gridded plate 3T3 3 T1-A 17.5 0.99939 3, 4, 5
Gridded plate 4 T2-A 15.5 4, 7 Gridded plate 5 T3-HA 17.5 0.99956
5, 6, 7 Gridded plate 6 T4-PA 18.5 0.99997 7, 9, 11 Gridded plate
Average for 17.2 transformed Std dev 1.095 P value = 0.0006 between
average normal cell and average transformed cell doubling.
.sup.aCells were grown and photographed or harvested at defined
time intervals to obtain cell counts in log phase. .sup.bData was
obtained from photographs of six grids on gridded plates.
.sup.cCells were harvested daily from places and live cells,
showing trypan blue exclusion, were counted in 4 chambers of a
hemocytometer.
REFERENCES
[1] A. Adey, J. N. Burton, J. O. Kitzman, J. B. Hiatt, A. P. Lewis,
B. K. Martin, . . . J. Shendure, The haplotype-resolved genome and
epigenome of the aneuploid HeLa cancer cell line, Nature 500 (7461)
(2013) 207-211, http://dx.doi.org/10.1038/nature12064
http://www.nature.com/nature/journal/v500/n7461/abs/nature12064.html-supp-
lementary-information. [2] Sylvan C. Baca, D. Prandi, Michael S.
Lawrence, Juan M. Mosquera, A. Romanel, Y. Drier, . . . Levi A.
Garraway, Punctuated evolution of prostate cancer genomes, Cell 153
(3) (2013) 666-677. [3] S. C. Bernstein, R. A. Weinberg, Expression
of the metastatic phenotype in cells transfected with human
metastatic tumor DNA, Proc. Natl. Acad. Sci. USA 88 (6) (1985)
1726-1730. [4] F. Z. Bischoff, L. C. Strong, S. O. Yim, D. R.
Pratt, M. J. Siciliano, B. C. Giovanella, M. A. Tainsky,
Tumorigenic transformation of spontaneously immortalized
fibroblasts from patients with a familial cancer syndrome, Oncogene
6 (2) (1991) 183-186. [5] L. B. Davis, W. M. Kuehl, J. F. Battey,
Basic Methods in Molecular Biology, Elsevier Press, New York, 1986.
[6] V. Espina, L. A. Liotta, What is the malignant nature of human
ductal carcinoma in situ?Nat. Rev. Cancer 11 (1) (2011) 68-75,
http://dx.doi.org/10.1038/nrc2950. [7] M. Goldfarb, K. Shimizu, M.
Perucho, M. Wigler, Isolation and preliminary characterization of a
human transforming gene from T24 bladder carcinoma cells, Nature
296 (5856) (1982) 404-409. [8] R. G. Greig, T. P. Koestler, D. L.
Trainer, S. P. Corwin, L. Miles, T. Kline, . . . G. Poste,
Tumorigenic and metastatic properties of "normal" and
ras-transfected NIH/3T3 cells, Proc. Natl. Acad. Sci. USA 82 (11)
(1985) 3698-3701. [9] H. H. Heng, J. B. Stevens, G. Liu, S. W.
Bremer, K. J. Ye, P. V. Reddy, . . . C. J. Ye, Stochastic cancer
progression driven by non-clonal chromosome aberrations, J. Cell
Physiol. 208 (2) (2006) 461-472,
http://dx.doi.org/10.1002/jcp.20685. [10] J. L. A. Jainchil, S. A.
Aaronson, G. J. Todaro, Murine sarcoma and leukemia viruses: assay
using clonal lines of contact-inhibited mouse cells, J. Virol. 4
(1969) 549. [11] V. M. Kavsan, V. P. Baklaushev, O. V. Balynska, A.
V. Iershov, P. O. Areshkov, G. M. Yusubalieva, . . . V. P.
Chekhonin, Gene encoding chitinase 3-like 1 protein (CHI3L1) is a
putative oncogene, Int. J. Biomed. Sci. 7 (3) (2011) 230-237. [12]
H. Korkaya, M. S. Wicha, HER2 and breast cancer stem cells: more
than meets the eye, Cancer Res. 73 (12) (2013) 3489-3493,
http://dx.doi.org/10.1158/0008-5472.can-13-0260. [13] K. Koziel, J.
Smigelskaite, A. Drasche, M. Enthammer, M. I. Ashraf, S. Khalid, J.
Troppmair, RAF and antioxidants prevent cell death induction after
growth factor abrogation through regulation of Bcl-2 proteins, Exp.
Cell Res. 319 (17) (2013) 2728-2738. [14] J. J. M. Landry, P. T.
Pyl, T. Rausch, T. Zichner, M. M. Tekkedil, A. M. Stutz, . . . L.
M. Steinmetz, The genomic and transcriptomic landscape of a HeLa
cell line, G3: Genes|Genomes|Genet. 3 (8) (2013) 1213-1224,
http://dx.doi.org/10.1534/g3.113.005777. [15] G. Leprivier, M.
Remke, B. Rotblat, A. Dubuc, A. Mateo, F. Rachele, M. Kool, . . .
Poul H. Sorensen, The eEF2 kinase confers resistance to nutrient
deprivation by blocking translation elongation, Cell 153 (5) (2013)
1064-1079. [16] L. A. Liotta, H-ras p21 and the metastatic
phenotype, J. Natl Cancer Inst. 80 (1988) 468-469. [17]
Littlefield, J. W., 1982. NIH/3T3 Editorial Comment
Science-1982-LITTLEFIELD-214-6.pdf. [18] G. Liu, J. B. Stevens, S.
D. Home, B. Y. Abdallah, K. J. Ye, S. W. Bremer, . . . H. H. Heng,
Genome chaos: survival strategy during crisis, Cell Cycle 13 (4)
(2014) 528-537, http://dx.doi.org/10.4161/cc.27378. [19] S. Liu, Y.
Cong, D. Wang, Y. Sun, L. Deng, Y. Liu, . . . M. S. Wicha, Breast
cancer stem cells transition between epithelial and mesenchymal
states reflective of their normal counterparts, Stem Cell Rep. 2
(1) (2014) 78-91, http://dx.doi.org/10.1016/j.stemcr.2013.11.009.
[20] T. Lowe, J. Sharefkin, S. Q. Yang, C. W. Dieffenbach, A
computer program for selection of oligonucleotide primers for
polymerase chain reactions, Nucleic Acids Res. 18 (7) (1990)
1757-1761. [21] M. Malumbres, M. Barbacid, RAS oncogenes: the first
30 years, Nat. Rev. Cancer 3 (2003) 7-13. [22] Meeker, L. D.,
Thompson, H. J., 1985. In: Rein, Robert (Ed.), A Multi-Path Theory
of Chemical Carcinogenesis in the Rat Mammary Gland. A. R. Liss,
Inc., N. Y., pp. 379-388. [23] N. Miliaras, Clinical genomics
transcends sequencing, Genet. Eng. Biotechnol. News 34 (2014)
18-21. [24] J. Pan, S.-S. Feng, Targeting and imaging cancer cells
by folate-decorated, quantum dots (QDs) loaded nanoparticles of
biodegradable polymers, Biomaterials 30 (6) (2009) 1176-1183,
http://dx.doi.org/10.1016/j. biomaterials. 2008.10.039. [25] B.
Przybojewska, G. Plucienniczak, Nucleotide sequence of c-H-ras-1
gene from B6C3F1 mice, Acta Biochim. Pol. 43 (3) (1996) 575-578.
[26] Y. Pylayeva-Gupta, E. Grabocka, D. Bar-Sagi, RAS oncogenes:
weaving a tumorigenic web, Nat. Rev. Cancer 11 (11) (2011) 761-774.
[27] D. B. Ray, F. J. Brenner, M. Show, A. Baker, K. Gleason, J.
Gressley, . . . P. Barry, Diagnosis of diseases: sample collection,
record keeping, processing techniques for molecular analysis of
protein, nuclear and mitochondrial DNA and RNA, in: S. K. Majumdar,
J. E. Huffman, F. J. Brenner, A. I. Panah (Eds.), Wildlife
Diseases: Landscape Epidemiology, Spatial Distribution and
Utilization of Remote Sensing Technology, Pennsylvania Academy of
Science, Easton, Pa., 2005, pp. 415-427. [28] E. P. Reddy,
Nucleotide sequence analysis of the T24 human bladder carcinoma
oncogene, Science 220 (1983) 1061-1063. [29] E. P. Reddy, R. K.
Reynolds, E. Santos, M. Barbacid, A point mutation is responsible
for the acquisition of transforming properties by the T24 human
bladder carcinoma oncogene, Nature 300 (5888) (1982) 149-152. [30]
H. Rubin, Growth regulation, reverse transformation, and
adaptability of 3T3 cells in decreased Mg2 concentration, Proc.
Natl. Acad. Sci. 78 (1) (1981) 328-332. [31] C. Scheel, R. A.
Weinberg, Cancer stem cells and epithelial-mesenchymal transition:
concepts and molecular links, Sem. Cancer Biol. 22 (5-6) (2012)
396-403, http://dx.doi.org/10.1016/j.semcancer.2012.04.001. [32] T.
Shibue, R. A. Weinberg, Metastatic colonization: settlement,
adaptation and propagation of tumor cells in a foreign tissue
environment, Sem. Cancer Biol. 21 (2) (2011) 99-106,
http://dx.doi.org/10.1016/j.semcancer.2010.12.003. [33] A.
Stepanenko, S. Andreieva, K. Korets, D. Mykytenko, N. Huleyuk, Y.
Vassetzky, V. Kavsan, Step-wise and punctuated genome evolution
drive phenotype changes of tumor cells, Mutat. Res./Fundam. Mol.
Mech. Mutagen. 771 (0) (2015) 56-69,
http://dx.doi.org/10.1016/j.mrfmmm.2014.12.006. [34] P. J.
Stephens, C. D. Greenman, B. Fu, F. Yang, G. R. Bignell, L. J.
Mudie, . . . P. J. Campbell, Massive genomic rearrangement acquired
in a single catastrophic event during cancer development, Cell 144
(1) (2011) 27-40, http://dx.doi.org/10.1016/j.cell.2010.11.055.
[35] J. B. Stevens, G. Liu, B. Y. Abdallah, S. D. Home, K. J. Ye,
S. W. Bremer, . . . H. H. Heng, Unstable genomes elevate
transcriptome dynamics, Int. J. Cancer 134 (9) (2014) 2074-2087,
http://dx.doi.org/10.1002/ijc.28531. [36] C. J. Tabin, S. M.
Bradley, C. I. Bargmann, R. A. Weinberg, A. G. Papageorge, E. M.
Scolnick, . . . E. H. Chang, Mechanism of activation of a human
oncogene, Nature 300 (5888) (1982) 143-149. [37] G. J. Todaro, H.
Green, Quantitative studies of the growth of mouse embryo cells in
culture and their development into established lines, J. Cell Biol.
17 (1963) 299-313. [38] C. M. Towne, J. F. Dudt, D. B. Ray, Effect
of Mansoa alliacea (bignonaceae) leaf extract on embryonic and
tumorigenic mouse cell lines, Journal of Medicinal Plants Research
9 (29) (2015) 799-805, http://dx.doi.org/10.5897/jmpr2015.5823.
[39] D. C. Wallace, weiwei Fan, Vincent Procaccio, Mitochondrial
energetics and therapeutics, Ann. Rev. Pathol. Mech. Dis., 5,
(2010) 297-348. [40] Y. Wang, H. Dong, M. Xu, B. Xin, W. Niu, D.
Xu, L. Liu, 37-kDa laminin receptor precursor promotes lung
adenocarcinoma cell invasion and metastasis by
epithelial-to-mesenchymal transition, Cancer Gene Ther. 21 (4)
(2014) 150-157, http://dx.doi.org/10.1038/cgt.2014.10. [41] O.
Warburg, On respiratory impairmant in cancer cells, Science 124
(1956) 269-270. [42] R. A. Weinberg, Coming full circle--from
endless complexity to simplicity and back again, Cell 157 (1)
(2014) 267-271, doi.
SEQUENCE LISTINGS
1
4123DNAHomo sapiens 1ctgtcttcaa catcccaaat gcc 23225DNAHomo sapiens
2agtgtggtat tccctggaca aaagg 25320DNAMus musculus 3ggccttagtt
cttcttgtcc 20420DNAMus musculus 4aaccaacaca aatagggagc 20
* * * * *
References